Contents
What is proprioception
Proprioception is the nonvisual sense of the position and movements of the body. Proprioception is the sense of determining the position and movement of the musculoskeletal system detected by special receptors in muscles and tendons. Proprioception can be defined as a specialized variation of the sensory modality of touch that encompasses the sensation of joint movement (kinesthesia) and joint position (joint position sense) 1. Proprioceptive information is provided through a variety of receptors in the periphery, including Ruffini receptors, paciniform afferents, and pacinian corpuscles. Paciniform afferents are best activated by compression stimuli 2. Ruffini receptors and pacinian corpuscles are both classified as dynamic receptors; however, Ruffini receptors have also been described as static receptors based on their low-threshold, slow-adapting characteristics. It has been suggested that these types of receptors are stimulated when a joint is moved near the end range of motion 2. Two other receptors that play a primary role in proprioception are the muscle spindle and Golgi tendon organ. The muscle spindle senses changes in muscle length, and the Golgi tendon organ identifies changes in muscle tension. Although the functions of these receptors have been previously established, their specific contributions to proprioception are still controversial.
Figure 1. Sensory receptors free nerve endings
The spinal nerves and cranial nerves carry the somatosensory signals from bones, joints, muscles, and the skin. These somatosensory signals are for touch, heat, cold, stretch, pressure, pain, and other sensations. One of the most important sensory roles of these nerves is proprioception, in which the brain receives information about body position and movements from nerve endings in the muscles, tendons, and joints. The principal receptor involved in proprioception is the muscle spindle, which includes the primary and secondary endings of spindles. The brain uses this information to adjust muscle actions and thereby maintain equilibrium (balance) and coordination. Neuroimaging studies showed that human kinesthesia is associated with a network of active brain areas that consists of motor areas, cerebellum, and the right fronto-parietal areas, including high-order somatosensory areas 3. Motor control for even simple tasks is a plastic process that undergoes constant review and modification based upon the integration and analysis of sensory input, efferent motor commands, and resultant movements 4.
Figure 2. Proprioceptors – muscle spindle
The role of proprioceptive information in motor control can be separated into 2 categories 5. The first category involves the role of proprioception with respect to the external environment. Motor programs often have to be adjusted to accommodate unexpected perturbations or changes in the external environment. Although the source of this information is often largely associated with visual input, there are many circumstances in which proprioceptive input is the quickest or the most accurate, or both. For example, modification of the motor program for walking was required in response to the uneven support surface. If the person’s vision was fixed on the box to be picked up, he or she might not have visually noted the uneven support surface. In addition to alterations in the plantar cutaneous receptors, muscle and joint mechanoreceptors would have reported the degree of altered ankle joint position and stimulated the motor program modification required. The planning of movements also requires attention to environmental constraints. This is especially true with respect to the selection of strategies for the maintenance of postural control. For example, sensory detection of an unstable handrail from peripheral signals (kinesthesia, changing joint positions) would alter the motor program used to avoid falling on a slippery staircase. During the planning stages of a movement, visual images are used to create a model of the environment in which the movement will occur. Proprioception has been described as essential during the movement execution to update the feed-forward commands derived from the visual image.
The second category of roles proprioceptive information plays in motor control is in the planning and modification of internally generated motor commands. Before and during a motor command, the motor control system must consider the current and changing positions of the joints involved to account for the complex mechanical interactions within the components of the musculoskeletal system. Proprioception best provides the needed segmental movement and position information to the motor control system. In the situation of a single joint moving through a 10° arc of motion, the precise muscle force required to perform the task depends upon the joint angle. As one can surmise, the task of determining how much tension in a muscle is required for a movement becomes extremely complex and important with movements involving several joints. Accompanying each angular change in joint position are changes in the mechanical advantages associated with all the muscles that traverse the joint. Many tasks involve a sequence of overlapping joint movements. The motor control system must consider the multiple motions occurring as both a direct function of muscle activation and indirectly from intersegmental dynamics (movement of one joint inducing movement of another). Proprioception provides much of the information required to solve all these movement problems.
Ascending Tracts (Spinal Cord White Matter)
The spinal cord has two principal functions in maintaining homeostasis: nerve impulse propagation and integration of information.
The white matter tracts in the spinal cord are highways for nerve impulse propagation (see Figure 3, 5 and 6). Sensory input travels along these tracts toward the brain, and motor output travels from the brain along these tracts toward skeletal muscles and other effector tissues.
The gray matter of the spinal cord receives and integrates incoming and outgoing information (see Figure 4).
Nerve impulses from sensory receptors propagate up the spinal cord to the brain along two main routes on each side:
- Spinothalamic tract: the spinothalamic tract conveys nerve impulses for sensing pain, temperature, itch, and tickle.
- Posterior column: The posterior column consists of two tracts: the gracile fasciculus and the cuneate fasciculus. The posterior column tracts convey nerve impulses for touch, pressure, vibration, and conscious proprioception (the awareness of the positions and movements of muscles, tendons, and joints).
The sensory systems keep the central nervous system (brain and spinal cord) informed of changes in the external and internal environments. The sensory information is integrated (processed) by interneurons in the spinal cord and brain. Responses to the integrative decisions are brought about by motor activities (muscular contractions and glandular secretions). The cerebral cortex, the outer part of the brain, plays a major role in controlling precise voluntary muscular movements. Other brain regions provide important integration for regulation of automatic movements. Motor output to skeletal muscles travels down the spinal cord in two types of descending pathways: direct and indirect. The direct motor pathways, also called pyramidal pathways, include the lateral corticospinal, anterior corticospinal, and corticobulbar tracts. They convey nerve impulses that originate in the cerebral cortex and are destined to cause voluntary movements of skeletal muscles. Indirect motor pathways, also called extrapyramidal pathways, include the rubrospinal, tectospinal, vestibulospinal, lateral reticulospinal, and medial reticulospinal tracts. These tracts convey nerve impulses from the brainstem to cause automatic movements and help coordinate body movements with visual stimuli. Indirect pathways also maintain skeletal muscle tone, sustain contraction of postural muscles, and play a major role in equilibrium by regulating muscle tone in response to movements of the head.
Ascending tracts carry sensory signals up the spinal cord. Sensory signals typically travel across three neurons from their origin in the receptors to their destination in the brain: a first-order neuron that detects a stimulus and transmits a signal to the spinal cord or brainstem; a second-order neuron that continues as far as a “gateway” called the thalamus at the upper end of the brainstem; and a third-order neuron that carries the signal the rest of the way to the cerebral cortex. The axons of these neurons are called the first- through third-order nerve fibers (Figure 3).
Three neurons are involved in the transmission of information from the periphery to the cerebrum (the brain): first-order, second-order, and third-order neurons. The first-order neurons of the gracile fasciculus enter the spinal cord by the dorsal root of the spinal nerve and synapse with the second-order neurons within the gracile nucleus (also termed the nucleus gracilis) in the medulla oblongata.
First-order neurons of the fasciculus cuneatus synapse with the second-order neurons within the cuneate nucleus (also termed the nucleus cuneatus) in the medulla oblongata. All of the second-order neurons in the posterior columns immediately decussate (cross over) to the contralateral (opposite side) of the medulla and ascend to the thalamus on the opposite side of the brain.
These second-order neurons form the medial lemniscus (lemniskos, ribbon). As the information travels toward the thalamus, neurons carrying the same type of sensory information (fine touch, pressure, and vibration) collected by cranial nerves V, VII, IX, and X enter the medial lemniscus. The ventral posterolateral nucleus of the thalamus integrates sensory information carried within the posterior columns. The ventral posterolateral nucleus sorts the sensory information according to the region of the body involved and sends it to specific regions of the primary somatosensory cortex. As a result of this sorting, you “know” the nature of the stimulus and its location. If it is relayed to another part of the somatosensory cortex, you will perceive the sensation as having originated in a different part of the body. For example, sensory information from the little finger on your right hand is sent to a specific part of the primary somatosensory cortex, and sensory information from your left knee is sent to another part of the somatosensory cortex. Your understanding of a particular stimulus as touch, rather than as temperature or pain, is due to the processing of the sensory information within the thalamus.
Figure 3. Sensory Ascending Tracts
Figure 4. Processing of sensory input and motor output by the spinal cord
Note: Sensory input is conveyed from sensory receptors to the posterior gray horns of the spinal cord, and motor output is conveyed from the anterior and lateral gray horns of the spinal cord to effectors (muscles and glands). Many nerves often described as motor are actually mixed because they carry sensory signals of proprioception from the muscles back to the central nervous system (spinal cord and brain).
The major ascending tracts are as follows. The names of most of them consist of the prefix spino- followed by a root denoting the destination of its fibers in the brain, although this naming system does not apply to the first two.
The major ascending tracts are as follows. The names of most of them consist of the prefix spino- followed by a root denoting the destination of its fibers in the brain, although this naming system does not apply to the first two.
Axons from the dorsal roots of spinal nerves entering the spinal cord inferior to T6 (thoracic spine #6) travel superiorly within the gracile fasciculus of the spinal cord. Those entering the spinal cord at or superior to T6 ascend within the cuneate fasciculus (also termed the fasciculus cuneatus) of the spinal cord.
Gracile fasciculus
The gracile fasciculus (also termed the fasciculus gracilis) carries signals from the midthoracic and lower parts of the body. Below vertebra T6, it composes the entire posterior spinal cord column. At T6, it is joined by the cuneate fasciculus, discussed next. It consists of first-order nerve fibers that travel up the ipsilateral side of the spinal cord and terminate at the gracile nucleus in the medulla oblongata of the brainstem. These fibers carry signals for vibration, visceral pain, deep and discriminative touch (touch whose location one can precisely identify), and especially proprioception from the lower limbs and lower trunk. Proprioception is the nonvisual sense of the position and movements of the body.
Cuneate fasciculus
The cuneate fasciculus joins the gracile fasciculus at the T6 level. It occupies the lateral portion of the posterior column and forces the gracile fasciculus medially. It carries the same type of sensory signals, originating from T6 and up (from the upper limbs and chest). Its fibers end in the cuneate nucleus on the ipsilateral side of the medulla oblongata. In the medulla, second-order fibers of the gracile and cuneate systems decussate and form the medial lemniscus, a tract of nerve fibers that leads the rest of the way up the brainstem to the thalamus. Third-order fibers go from the thalamus to the cerebral cortex. Because of decussation, the signals carried by the gracile and cuneate fasciculi ultimately go to the contralateral cerebral hemisphere.
Spinothalamic tract
The spinothalamic tract and some smaller tracts form the anterolateral system, which passes up the anterior and lateral columns of the spinal cord. The spinothalamic tract carries signals for pain, temperature, pressure, tickle, itch, and light or crude touch. Light touch is the sensation produced by stroking hairless skin with a feather or cotton wisp, without indenting the skin; crude touch is touch whose location one can only vaguely identify.
In this pathway, first-order neurons end in the posterior horn of the spinal cord near the point of entry. Here they synapse with second-order neurons, which decussate and form the contralateral ascending spinothalamic tract. These fibers lead all the way to the thalamus. Third-order neurons continue from there to the cerebral cortex. Because of decussation, sensory signals in this tract arrive in the cerebral hemisphere contralateral to their point of origin.
Spinoreticular tract
The spinoreticular tract also travels up the anterolateral system. It carries pain signals resulting from tissue injury. The first-order sensory neurons enter the posterior horn and immediately synapse with second-order neurons. These decussate to the opposite anterolateral system, ascend the cord, and end in a loosely organized core of gray matter called the reticular formation in the medulla and pons. Third-order neurons continue from the pons to the thalamus, and fourth-order neurons complete the path from there to the cerebral cortex.
Posterior and anterior spinocerebellar tracts
The posterior and anterior spinocerebellar tracts travel through the lateral column and carry proprioceptive signals from the limbs and trunk to the cerebellum at the rear of the brain. Their first-order neurons originate in muscles and tendons and end in the posterior horn of the spinal cord. Second-order neurons send their fibers up the spinocerebellar tracts and end in the cerebellum.
Fibers of the posterior tract travel up the ipsilateral side of the spinal cord. Those of the anterior tract cross over and travel up the contralateral side but then cross back in the brainstem to enter the ipsilateral side of the cerebellum. Both tracts provide the cerebellum with feedback needed to coordinate muscle action.
Figure 5. Locations of major sensory system shown in cross section of the spinal cord
Figure 6. Spinal cord ascending tracts to the brain
Table 1. Major Ascending (Sensory) Tracts and the Sensory Information They Provide
Proprioception disorder
Proprioceptive signals from mechanoreceptors of the joints, muscles, tendons, and skin are essential for the intact neural control of movement. The loss of proprioceptive afferents may affect the control of muscle tone, disrupts postural reflexes 6 and severely impairs spatial 7 as well as temporal aspects 8 of volitional movement. Numerous neurological and orthopedic conditions are associated with proprioceptive and kinesthetic impairment such as stroke 9, Parkinson’s disease 10, focal dystonia 11, peripheral sensory neuropathies 12, or injuries to ligaments, joint capsules, and muscles 13.
Recognizing that the processing of proprioceptive signals has conscious and unconscious components implies that the available methods for assessing proprioceptive function may only address one of the two aspects of proprioception. For assessing the perceptual aspect of proprioception, psychophysical thresholds represent the gold standard 14. In addition, determining a joint position error when matching the position of two homologous limbs (e.g., two arms), is the most easily acquired measure of proprioceptive function and common in clinical practice 15. For determining the contribution of proprioceptive signals for balance control, many biomechanical measures have been employed such as latencies and amplitudes of electromyographic signals, joint kinematics or kinetics, or variables indicative of the postural sway of the body’s center of mass. With respect to proprioceptive training this means that an intervention focusing on training the proprioceptive sense may train one or both aspects of proprioception, that is, the conscious perceptual or the unconscious or implicit sensorimotor aspect.
Falls and Balance Disorder
Falls are one of the most serious health risks for older adults. They are not only the leading cause of injury-related deaths in this population, but they are also a significant cause of disability. In fact, falls cause ten percent of all emergency department visits and more than half of injury-related hospitalizations. Approximately one in three older adults falls each year. As the number of older adults increases rapidly over the next decade, the annual cost for fall-related injuries is expected to skyrocket, reaching $44 billion by 2020 16. Although most falls don’t result in severe physical injuries, a fall or near-fall often produces a psychological fear of falling. This contributes to a self-imposed decrease in activity, followed by functional declines and a greater risk for falls.
Falls in older adults are often viewed as unpredictable and unavoidable accidents. However, in many cases, falls are caused by a loss of balance or the inability to maintain the body’s center of gravity over its base of support. There are two types of balance:
- Static balance, which is the ability to control postural sway during quiet standing; and
- Dynamic balance, which is the ability to react to changes in balance and to anticipate changes as the body moves. Dynamic balance includes maintaining balance while walking and stepping over or around objects.
The ability to balance depends in large part on sensory, muscular and motor systems. The three most influential sensory systems are the visual, vestibular and somatosensory systems. With advancing age, balance tends to decline due to lower muscular strength and flexibility, as well as numerous other causes including loss of proprioception and inner ear problems, which negatively affects balance. Understanding these systems is essential to providing exercise programs that target balance for older adults.
The visual system is a major contributor to balance, providing information about the environment, the location of the person and the direction and speed of the person’s movement in the environment. Visual acuity, depth perception, peripheral field and sensitivity to low spatial frequencies (requiring more contrast to detect spatial differences) decrease with age. As a result, older adults tend to have a reduced ability to use visual cues to control balance.
The vestibular system, located in the ears, provides information about movement of the head, independent of visual cues. One component, the otoliths, detects head movement in relation to gravity, such as degree and direction of head tilt. The other component, the semicircular canals, are fluid-filled canals composed of three half circles positioned in three different planes. As the head moves, fluid in the canals triggers receptors and information is sent to the brain where it provides input about head orientation. At approximately age 40, vestibular neurons start to decrease in number and size, resulting in various impairments including dizziness.
According to the National Institute of Health, nine percent of adults ages 65 and older report having difficulty with balance. This, along with a decline in lower body strength and stability, leads to an alarming 300,000 hospital admissions for fall-related injuries among older adults each year. The good news is that balance can be improved with simple exercises that can be done in therapy settings, health clubs or even at home.
Proprioception training
Proprioceptive training is an intervention that targets the improvement of proprioceptive function, focusing on the use of somatosensory signals such as proprioceptive or tactile afferents in the absence of information from other modalities such as vision. Its ultimate goal is to improve or restore sensory and/or sensorimotor function.
Given the importance of proprioception for motor control, it has been argued that therapies aiming to restore motor function after injury should focus on training the proprioceptive sense. Numerous interventions claim to constitute a form of proprioceptive training that improves proprioception and aids motor recovery. Unfortunately, there is little agreement of what actually constitutes proprioceptive training, which may be partially owed to the fact that there are various definitions for the term proprioception 17. Broadly defined, proprioception refers to the conscious awareness of body and limbs and has several distinct properties: passive motion sense, active motion sense, limb position sense, and the sense of heaviness. However, it has long been established that proprioception has an unconscious component in which proprioceptive signals are used for the reflexive control of muscle tone and the control of posture that has long been recognized. In order to distinguish between the conscious and unconscious processing of proprioceptive afferents it has been suggested to refer to kinesthesia as the conscious perception of limb and body position and motion and to reserve the term proprioception for referring to the unconscious processing of proprioceptive information. However, this distinction is not without problems, because the term kinesthesia has also been used to indicate motion sense in distinction to position sense.
The somatosensory system provides information about the body’s position and contact from the skin through pressure, vibration and tactile sensors, as well as joint and muscle proprioceptors. Skin sensation via tactile, vibration and pressure sensors is important in all activities of daily living, especially those involving movement. Skin sensitivity is reduced with increasing age. Lack of input from tactile, pressure and vibration receptors makes it difficult to stand or walk and detect changes in heel-to-toe body weight shifts, which are important in maintaining balance.
In addition to the three sensory systems, muscle strength plays a role in balance and mobility. Muscles are particularly important in stability, since they work to keep the center of gravity within the base of support.
A basic premise of exercise science is that in order to improve the function of a system through exercise, the exercise must stimulate that system. Many studies have shown that strength can be improved in older adults using different types of resistance training. However, resistance training alone has only a modest effect on improving balance, even though strength and balance are related. This is likely because the ability to maintain balance involves a several processes that require the successful integration of multiple components, including several sensory systems not typically affected by resistance training. Exercise programs that target the physiological systems in balance control, specifically the visual, vestibular, somatosensory and muscular systems, can improve balance and reduce the risk for falls.
Balance exercises involve maintaining standing and postural stability under a variety of static and dynamic conditions. Activities in a balance-training program can include standing with one foot in front of the other to alter the base of support, shifting the body weight in different directions and lifting the feet from the floor. Exercises can also be performed with the eyes closed, while moving the head and/or while standing on foam to target the visual, vestibular and somatosensory systems respectively. A variety of activities are used to increase strength using body weight or equipment such as cuff weights or elastic resistance bands. Exercises which simulate activities of daily living, such as reaching forward to put something on a shelf, can then incorporate resistance and dynamic balance to challenge balance further. However, when performing these exercises it is important to have a chair or other sturdy surface within reach in case it becomes necessary to steady one’s self.
The potential applications of balance training are tremendous. Training can be performed to reduce the risk of additional falls in chronic fallers or improve balance in aging adults who want to reduce the risk of their first fall.
Furthermore, balance exercises can be incorporated into rehabilitation programs for people with hip fracture, stroke and arthritis.
Reducing fall risk by improving balance in older adults will not only avoid increasing health care costs, but it also will give older adults a more active life. Further, providing effective interventions to target fall risk can make a major difference in quality of life for these individuals.
Types of proprioceptive training
Active movement/balance training
The active movement/balance training included studies in which participants actively moved a limb, limb segment or the whole body. The following interventions were employed: single-joint active movements, single-joint passive and active movements, multi-joint passive and active movements, multi-joint active movements, and whole body balance training.
Multi-joint active movements consisted of upper limb tasks such as reaching to or grasping a target with or without additional sensory feedback (e.g., vision) 18 or lower limb tasks such as stepping on specific targets 19. In general, studies applying multi-joint, active movement training tasks reported improvements that ranged widely from 2.5 to 80.2% (mean: 39%) from pre- to post-test.
With respect to goal-directed reaching or grasping with or without assistance, robot-aided upper limb movements guided by online haptic feedback (assist-as-needed force) showed the greatest degree of accuracy improvement 20. After 10-h of training, nine chronic stroke patients (vision occluded) substantially reduced reaching endpoint error by 81.4% (mean change: 53.2–9.9 cm) and by 80% when visual feedback was present.
Studies seeking to train balance applied a multitude of activities including walking and stair-stepping exercises, single and double leg balance exercises with and without vision, sit-to-stand exercises, standing, walking, or jumping on stable and unstable surfaces and sport specific exercises. Across all studies pre- to post-test improvements ranged between 16 and 97% (mean: 41%). Three of these studies conducted active dynamic exercise training and examined knee position sense using active/passive joint matching tests 21, 22, 23 reporting reductions in knee joint position error between 15 and 63% as a result of training.
Passive movement training
These interventions typically required some type of passive motion apparatus and focused either on single-joint (wrist or knee) 24 or multi-joint movement (thumb movement or assisted reaching via robotic arm) (Kaelin-Lang et al., 2005; Wong et al., 2011). Although there are several forms of apparatus’ that have been used that are often customized for a specific joint or movement type. Like active training protocols widely divergent rates of functional or sensory improvement were reported ranging from a 0 to 47% change from pre- to post-test. Most occluded vision of the moving limb but some allowed vision or provided additional synchronized auditory feedback. All studies reported a range of 0–23% improvements.
Somatosensory stimulation training
This type of training included various forms of stimulation that was geared exclusively to somatosensation. Somatosensory stimulation training apply muscle or vibro-tactile vibration ranging from whole-body vibration to local vibration of a single segment. Other forms of stimulation included thermal stimulation, multi-somatosensory stimulation, magnetic stimulation, electrical stimulation and acupuncture.
Studies utilizing whole-body vibration during training mostly targeted patients with Parkinson’s disease or stroke. Typical vibration frequencies were either between 25 and 30 Hz or <10 Hz. Most studies applied vibration for a duration of less than 1 min per trial. After four 45-second bouts of 30 Hz vibration with eyes closed, a significant reduction in whole body sway displacement was observed in stroke patients while standing. When vibration was stopped and patients were allowed to open their eyes, whole body sway velocity was reduced in a visually-guided weight-shift task.
Somatosensory discrimination training
This training focused on the ability to discriminate between two somatosensory stimuli. These discrimination training tasks consisted of haptic discrimination (e.g., active exploration of objects with the hand), tactile discrimination (of textures), wrist or ankle joint position discrimination and wrist joint velocity discrimination tasks. Improvements in haptic or proprioceptive acuity ranged from 12 to 67% (mean improvement of 38%). The two studies training wrist joint position discrimination (with graded difficulty) as a training regimen for stroke patients showed improvements of 57–67% in wrist joint angle position matching. In absolute terms joint position error was reduced from approximately 25–30° at pre-test to 8–10° at post-test.
Combined/multiple system training
Three studies used either multiple components of the three main categories mentioned above or utilized multi-sensory approaches. Noteworthy was a study by McKenzie et al. 25 who combined both active movement training with somatosensory discrimination tasks to treat focal hand dystonia patients. Subjects with writers’ cramp showed a 90% improvement in finger target location error (baseline error: 14 mm, post-intervention: 1.4 mm) and subjects with musician’s cramp showed a 22% improvement (baseline error: 3.87 mm, post-intervention: 3 mm).
Effectiveness of proprioceptive training
Evaluating scientific evidence on the effectiveness of proprioceptive training has been challenging given the heterogeneity in training methods and obtained measurements. Many studies used a variety of active and passive movement procedures, often combining both methods for their analysis, which makes it difficult to assess the contribution of each training method for improving proprioceptive and/or motor function. Another factor that very likely contributed to the variability of the reported effectiveness was the widely divergent duration of the intervention ranging from a single session to 6 months.
Based on the results of a systematic review 17 on the effectiveness of proprioceptive training, the following general conclusions can be made:
First, proprioceptive training can be effective in improving proprioceptive function. The majority of studies reported improvement rates above 20% 17.
- There is initial evidence that training methods of progressive rehabilitation that includes some form of somatosensory stimulation, utilizing a combination of both passive and active movement with and without exteroceptive feedback, are most beneficial (typically reporting large effect sizes).
- Second, longer lasting interventions seem to produce greater benefits. Training regimens lasting 6 weeks or longer tended to yield relatively higher improvements in proprioceptive and/or motor function 26, although somatosensory stimulation has shown to yield very rapid gain within a single session or few hours of intervention 27.
- Third, proprioceptive training is applicable to a wide range of clinical populations. Patients suffering from proprioceptive impairment may benefit from the procedure disregarding if the cause of the impairment is neurological or musculoskeletal in nature.
However, the reader needs to recognize that the above conclusions are preliminary in nature. While the reported effectiveness data are, in many respects, impressive, there are also reports that showed no or only minimal functional gains. The reasons for this discrepancy are not necessarily obvious. However, small differences in protocol may yield large differences in outcome. Further, there is very little clarity on what constitutes an optimal training dosage. That is, what are best practices for session duration, number of weekly sessions, and overall duration of the intervention ? While a 6-week period has shown to produce positive results, it is by no means clear that this is an optimal duration. Related to dosage is the notion of retention. Only a few studies investigated retention of function over a period of 45 min 28 or 6 weeks 29. Thus, for many of the applied training methods there are no firm data on the associated decay of learning, which would be important knowledge to have for any follow-up therapy. Another aspect rarely addressed so far concerns the specificity or generalizability of training. While the majority of studies reported gains in proprioceptive and/or motor performance, surprisingly little is known, if such gains transfer beyond the trained tasks. The data documenting cortical reorganization following treatment are helpful 30, because they hint that proprioceptive training induces generic neural changes that are not task-specific. However, there is still no comprehensive evidence available to support this claim across the reviewed training methods.
Proprioception exercises
Balance Training
Balance is key to activities of daily living, performance, fall prevention and independence. Balance can be affected by muscular strength and endurance, as well as proprioception, inner ear function and eye sight. It can be maintained and even improved as we age through practicing balance-specific training exercises. Research has shown that using specific tools in a safe environment can be particularly effective in improving your balance and enhancing your postural stability. Research has also shown that this type of training helps to reduce back, knee and ankle injuries.
Balance training tools come in all shapes and sizes. They can be as simple as your own two feet or a pillow, or they can be made of foam, wood, rubber, springs, etc. These tools, along with balance training exercises, can rehabilitate, strengthen muscles and condition the body, as well as improve stability and postural alignment and help prevent falls. Men and women of all ages and activity levels can benefit from balance training. Start simply and progress slowly, gradually increasing the difficulty of the exercises. You may start with exercises such as balancing on one foot and then progress to using simple foam filled balance pads to challenge the body on an unstable surface.
Balance Training Tools
Regular exercise, such as walking, can increase your strength and coordination, which are important for maintaining balance. The following provide more targeted balance training.
Balance Pillow
This foam-filled pad allows you to sit or stand (with one foot or two) on a spongy, unstable surface to improve posture and stability. This is a great tool for someone starting a balance training program.
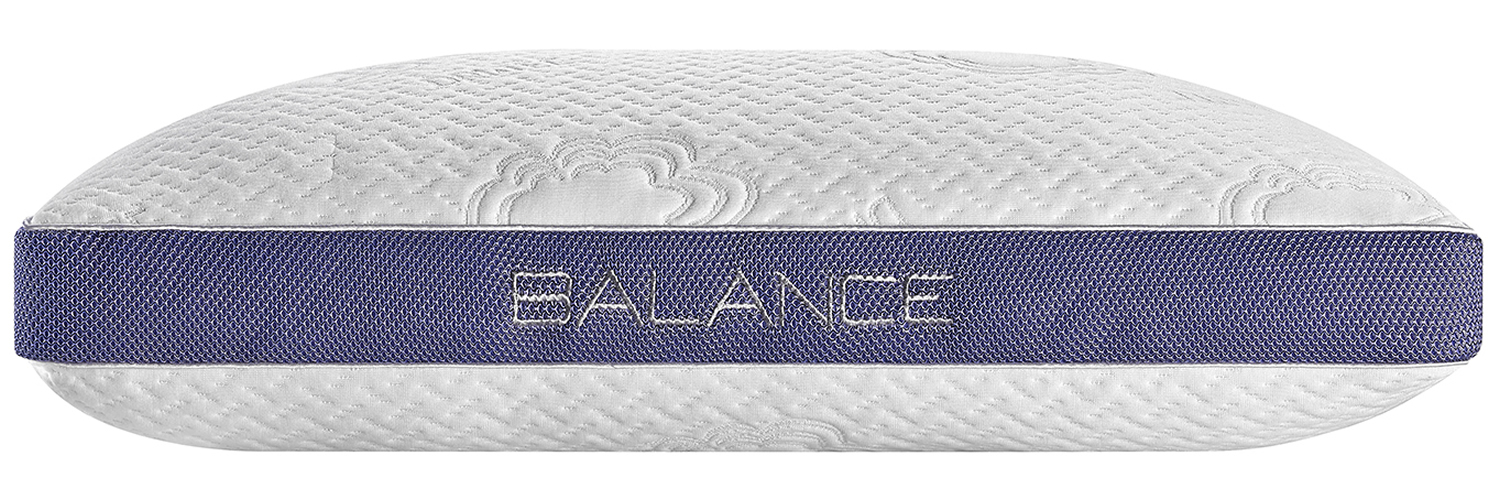
Balance Disc
This tool is a round rubber disc inflated with air to create an unstable surface. Balance discs can be used while seated or standing to improve balance and coordination or to add intensity to body-strengthening exercises.
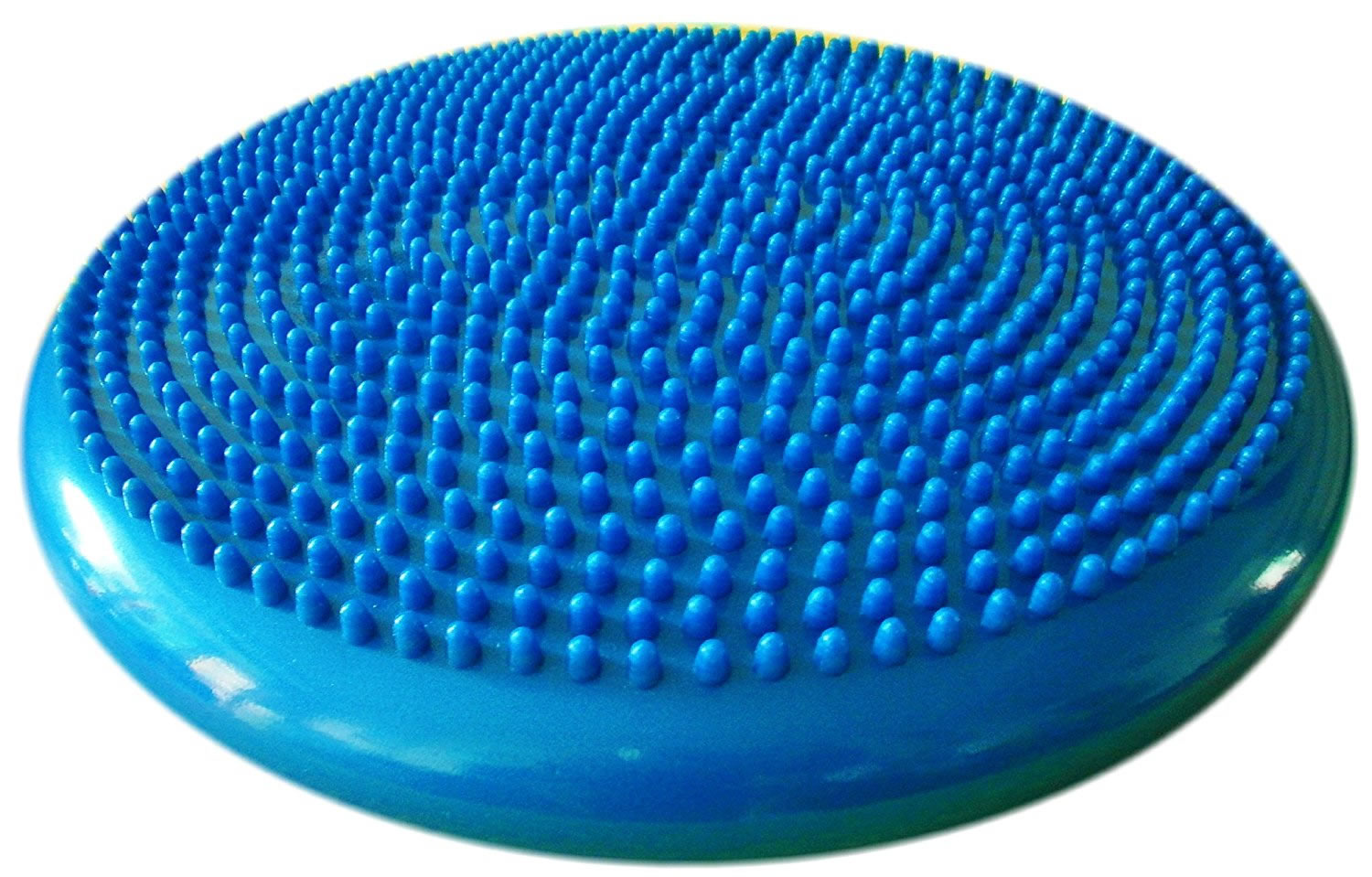
Blue Half-Ball
This is a stability ball cut in half with a flat, hard bottom. You can stand, sit or kneel on the air-filled ball portion of the tool or on the hard plastic side with the round surface down to create a rocking balance exercise. Blue half-balls are great for balance, core stability and proprioception training.
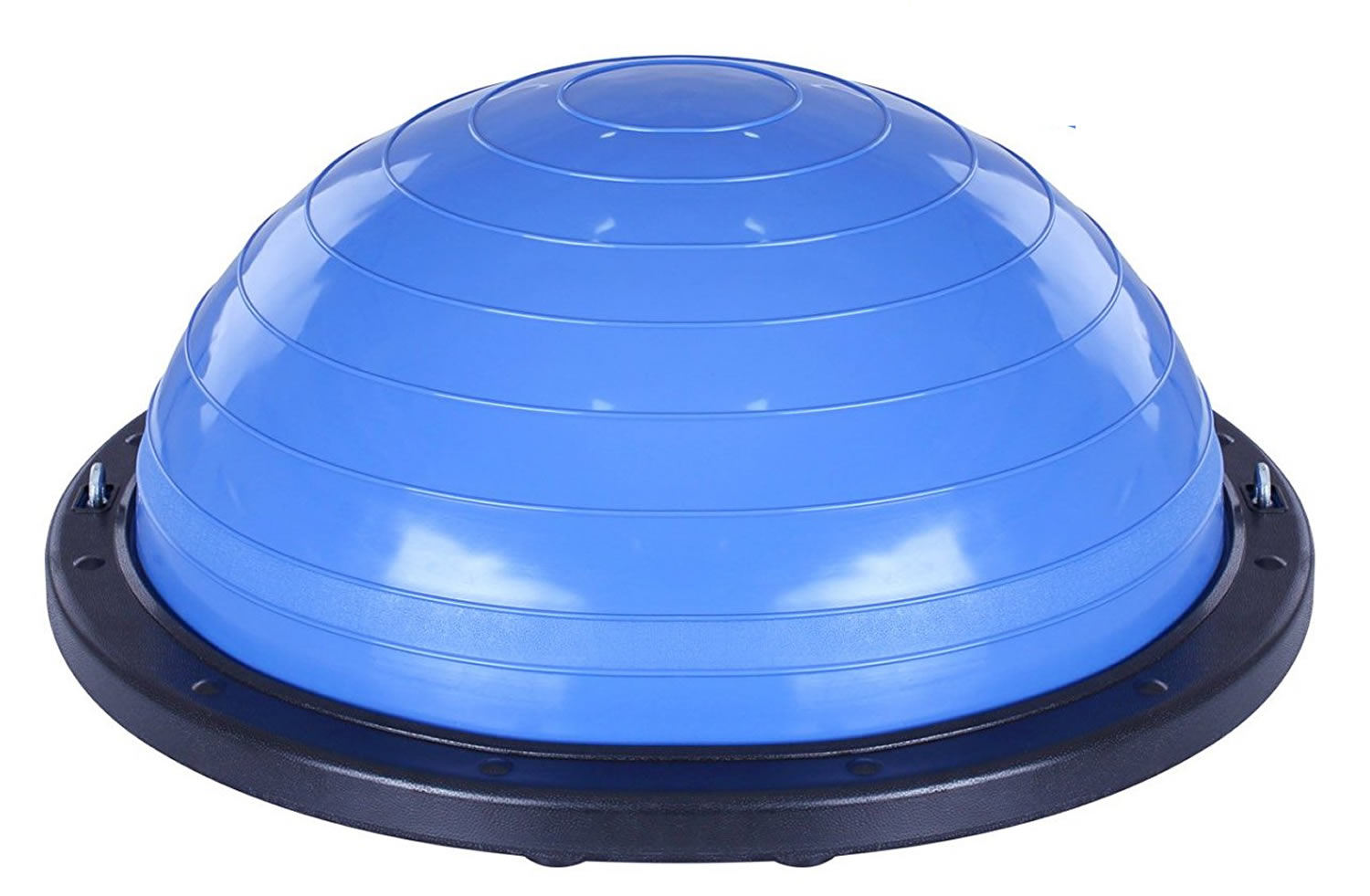
Foam Roller
This tool comes in a variety of shapes and sizes. These foam-filled balance, posture and core training tools can be round, half-round, short (1′) or long (3′). To use this tool you may kneel, stand, lie or sit on the device.
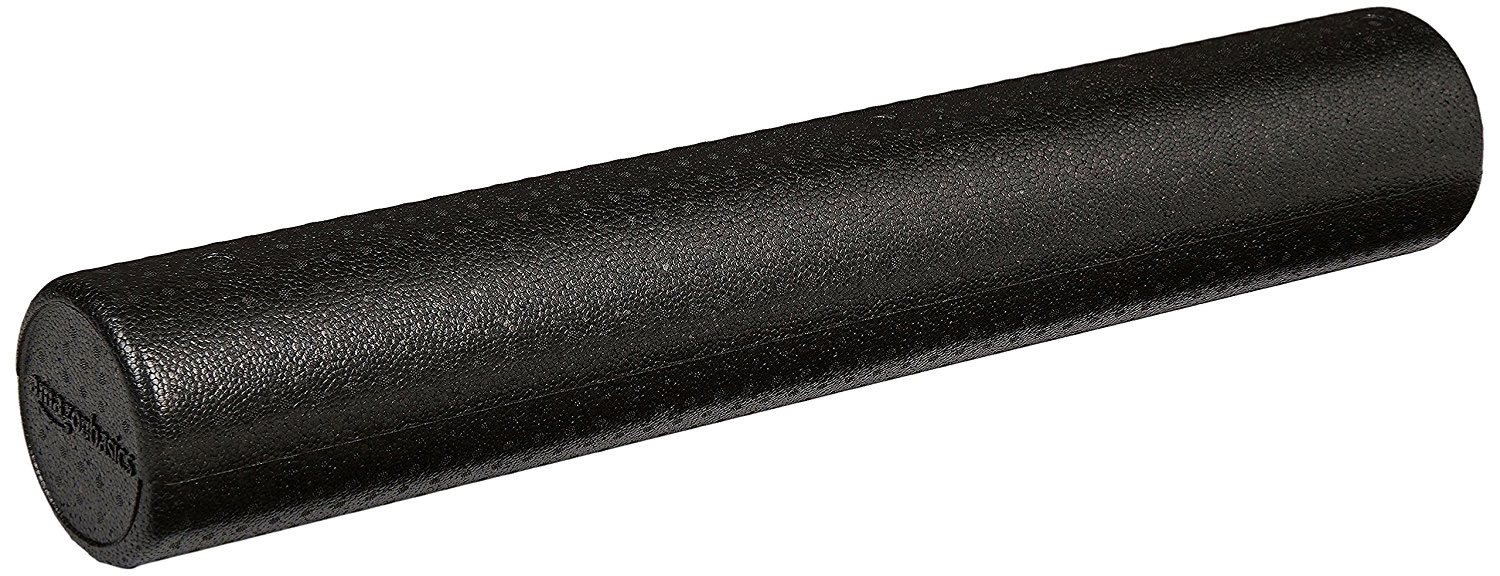
Balance Board
The flat top to this tool allows you to stand and practice your balance while elevated on a spherical base. The range of motion that the device allows can be adjusted for changes in difficulty.
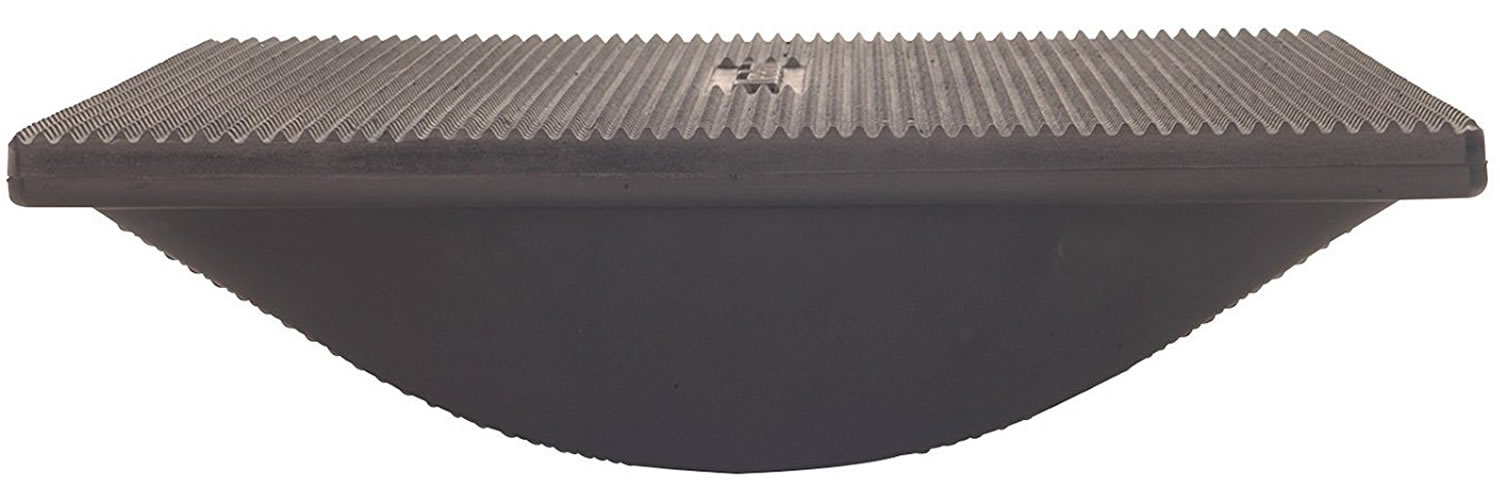
Stability Ball
This inflatable ball comes in different sizes and can be used as a balance and/or strength-training aid.
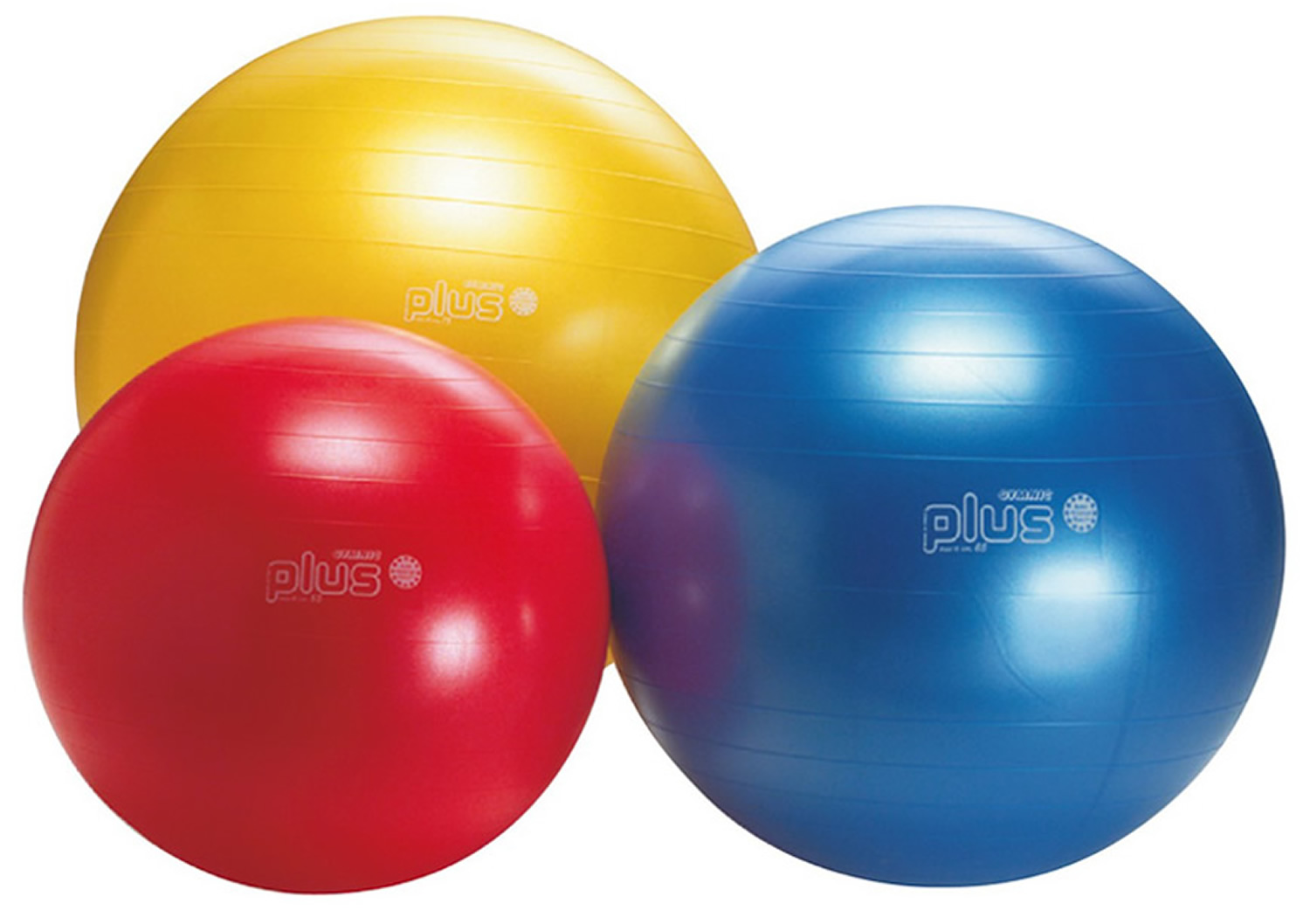
SAFETY AND CONSIDERATIONS
Balance training tools should only be used on a flat, stable, non-slip surface. Be sure to consult with your physician prior to starting a balance training program.
Always practice balance training near a stable structure (such as a wall, bar or counter) to give you some assistance if you slip or begin to fall; or practice under the supervision of a qualified professional.
IMPLEMENTING A BALANCE TRAINING PROGRAM
When starting any new training program, it is important to use a gradual progression.
Begin with low-intensity exercises and progress to more challenging exercises.
When performing balance exercises, it is helpful to establish a stable, non-moving focal point. This will keep your attention and allow you to focus your eyesight for better stability.
GETTING STARTED
At first, you may start with simple balance training exercises – such as standing on one foot for a few seconds, and then gradually increasing your time for more difficulty.
General guidelines for balance training include:
- Start with a relatively stable foundation or position before progressing to a less stable foundation or position.
- Start with static or stationary position (holding a position) before adding any movement (e.g., walking or stepping) or resistance (e.g., adding a hand weight).
- From there, you may add movement to your balance pose, such as lifting your arms while still keeping your balance on one foot.
When you need to be challenged beyond this exercise, add a balance tool of your choice. You might start by sitting on a blue half-ball with two feet on the ground, and then move to one-footed contact while seated. You can move to standing on a blue half-ball with both feet while using a stationary aid like a wall, railing or chair for balance assistance. Finally, you can progress from standing on a blue half-ball without assistance to stepping on and off the half-ball with assistance, then without.
To get set up with a personalized program, one that is appropriate for your current fitness level and balance abilities, consult your physical therapist or fitness professional. To reduce risk of injury from falls, community dwelling older adults with substantial risk of falls (e.g., with frequent falls or mobility problems) should perform exercises that maintain or improve balance. Balance training should be performed daily for improvement in overall stability. Perform balance training before you do resistance training, so your muscles are not fatigued, to ensure that it works.
- The role of proprioception in the management and rehabilitation of athletic injuries. Am J Sports Med. 1997 Jan-Feb;25(1):130-7. http://journals.sagepub.com/doi/pdf/10.1177/036354659702500126[↩]
- Grigg P. Peripheral neural mechanisms in proprioception. J Sport Rehabil. 1994;3:2–17.[↩][↩]
- Visuokinesthetic perception of hand movement is mediated by cerebro-cerebellar interaction between the left cerebellum and right parietal cortex. Hagura N, Oouchida Y, Aramaki Y, Okada T, Matsumura M, Sadato N, Naito E. Cereb Cortex. 2009 Jan; 19(1):176-86. https://www.ncbi.nlm.nih.gov/pmc/articles/PMC2638744/[↩]
- Leonard C T. The Neuroscience of Human Movement. Mosby-Year Book Inc; St Louis, MO: 1998.[↩]
- Animal solutions to problems of movement control: the role of proprioceptors. Hasan Z, Stuart DG. Annu Rev Neurosci. 1988; 11():199-223. http://www.annualreviews.org/doi/pdf/10.1146/annurev.ne.11.030188.001215[↩]
- Dynamic sensorimotor interactions in locomotion. Rossignol S, Dubuc R, Gossard JP. Physiol Rev. 2006 Jan; 86(1):89-154. http://physrev.physiology.org/content/86/1/89.long[↩]
- Impairments of reaching movements in patients without proprioception. I. Spatial errors. Gordon J, Ghilardi MF, Ghez C. J Neurophysiol. 1995 Jan; 73(1):347-60. https://www.ncbi.nlm.nih.gov/pubmed/7714577/[↩]
- The role of proprioception in the control of prehension movements: a kinematic study in a peripherally deafferented patient and in normal subjects. Gentilucci M, Toni I, Chieffi S, Pavesi G. Exp Brain Res. 1994; 99(3):483-500. https://www.ncbi.nlm.nih.gov/pubmed/7957728/[↩]
- Anatomical correlates of proprioceptive impairments following acute stroke: a case series. Kenzie JM, Semrau JA, Findlater SE, Herter TM, Hill MD, Scott SH, Dukelow SP. J Neurol Sci. 2014 Jul 15; 342(1-2):52-61. https://www.ncbi.nlm.nih.gov/pubmed/24819922/[↩]
- Parkinson’s disease accelerates age-related decline in haptic perception by altering somatosensory integration. Konczak J, Sciutti A, Avanzino L, Squeri V, Gori M, Masia L, Abbruzzese G, Sandini G. Brain. 2012 Nov; 135(Pt 11):3371-9. https://www.ncbi.nlm.nih.gov/pubmed/23169922/[↩]
- Kinesthesia is impaired in focal dystonia. Putzki N, Stude P, Konczak J, Graf K, Diener HC, Maschke M. Mov Disord. 2006 Jun; 21(6):754-60. https://www.ncbi.nlm.nih.gov/pubmed/16482525/[↩]
- Roles of proprioceptive input in the programming of arm trajectories. Ghez C, Gordon J, Ghilardi MF, Christakos CN, Cooper SE. Cold Spring Harb Symp Quant Biol. 1990; 55():837-47. http://symposium.cshlp.org/content/55/837.long[↩]
- Proprioception after an acute knee ligament injury: a longitudinal study on 16 consecutive patients. Fridén T, Roberts D, Zätterström R, Lindstrand A, Moritz U. J Orthop Res. 1997 Sep; 15(5):637-44. https://www.ncbi.nlm.nih.gov/pubmed/9420590/[↩]
- Assessing proprioceptive function: evaluating joint position matching methods against psychophysical thresholds. Elangovan N, Herrmann A, Konczak J. Phys Ther. 2014 Apr; 94(4):553-61. https://www.ncbi.nlm.nih.gov/pubmed/24262599/[↩]
- Proprioceptive acuity assessment via joint position matching: from basic science to general practice. Goble DJ. Phys Ther. 2010 Aug; 90(8):1176-84. https://www.ncbi.nlm.nih.gov/pubmed/20522675/[↩]
- Balance and Fall Prevention. American College of Sports Medicine. http://www.acsm.org/public-information/articles/2016/10/07/balance-and-fall-prevention[↩]
- Aman JE, Elangovan N, Yeh I-L, Konczak J. The effectiveness of proprioceptive training for improving motor function: a systematic review. Frontiers in Human Neuroscience. 2014;8:1075. doi:10.3389/fnhum.2014.01075. https://www.ncbi.nlm.nih.gov/pmc/articles/PMC4309156/[↩][↩][↩]
- Can proprioceptive training improve motor learning? Wong JD, Kistemaker DA, Chin A, Gribble PL. J Neurophysiol. 2012 Dec; 108(12):3313-21. https://www.ncbi.nlm.nih.gov/pmc/articles/PMC3544879/[↩]
- Efficacy of 2 non-weight-bearing interventions, proprioception training versus strength training, for patients with knee osteoarthritis: a randomized clinical trial. Lin DH, Lin CH, Lin YF, Jan MH. J Orthop Sports Phys Ther. 2009 Jun; 39(6):450-7. https://www.ncbi.nlm.nih.gov/pubmed/19531879/[↩]
- Minimally assistive robot training for proprioception enhancement. Casadio M, Morasso P, Sanguineti V, Giannoni P. Exp Brain Res. 2009 Apr; 194(2):219-31. https://www.ncbi.nlm.nih.gov/pubmed/19139867/[↩]
- Effects of kinesthesia and balance exercises in knee osteoarthritis. Diracoglu D, Aydin R, Baskent A, Celik A. J Clin Rheumatol. 2005 Dec; 11(6):303-10. https://www.ncbi.nlm.nih.gov/pubmed/16371799/[↩]
- A multi-station proprioceptive exercise program in patients with bilateral knee osteoarthrosis: functional capacity, pain and sensoriomotor function. A randomized controlled trial. Sekir U, Gür H. J Sports Sci Med. 2005 Dec; 4(4):590-603. https://www.ncbi.nlm.nih.gov/pmc/articles/PMC3899676/[↩]
- Effect of proprioception training on knee joint position sense in female team handball players. Pánics G, Tállay A, Pavlik A, Berkes I. Br J Sports Med. 2008 Jun; 42(6):472-6. https://www.ncbi.nlm.nih.gov/pubmed/18390919/[↩]
- Active versus passive training of a complex bimanual task: is prescriptive proprioceptive information sufficient for inducing motor learning? Beets IA, Macé M, Meesen RL, Cuypers K, Levin O, Swinnen SP. PLoS One. 2012; 7(5):e37687. https://www.ncbi.nlm.nih.gov/pmc/articles/PMC3359339/[↩]
- Differences in physical characteristics and response to rehabilitation for patients with hand dystonia: musicians’ cramp compared to writers’ cramp. McKenzie AL, Goldman S, Barrango C, Shrime M, Wong T, Byl N. J Hand Ther. 2009 Apr-Jun; 22(2):172-81; quiz 182. https://www.ncbi.nlm.nih.gov/pubmed/19389604/[↩]
- Multistation proprioceptive exercise program prevents ankle injuries in basketball. Eils E, Schröter R, Schröder M, Gerss J, Rosenbaum D. Med Sci Sports Exerc. 2010 Nov; 42(11):2098-105. https://www.ncbi.nlm.nih.gov/pubmed/20386339/[↩]
- Effects of wrist tendon vibration on arm tracking in people poststroke. Conrad MO, Scheidt RA, Schmit BD. J Neurophysiol. 2011 Sep; 106(3):1480-8. https://www.ncbi.nlm.nih.gov/pmc/articles/PMC3174817/[↩]
- Short-term effects of whole-body vibration on postural control in unilateral chronic stroke patients: preliminary evidence. van Nes IJ, Geurts AC, Hendricks HT, Duysens J. Am J Phys Med Rehabil. 2004 Nov; 83(11):867-73. https://www.ncbi.nlm.nih.gov/pubmed/15502741/[↩]
- Long-term effects of 6-week whole-body vibration on balance recovery and activities of daily living in the postacute phase of stroke: a randomized, controlled trial. van Nes IJ, Latour H, Schils F, Meijer R, van Kuijk A, Geurts AC. Stroke. 2006 Sep; 37(9):2331-5. http://stroke.ahajournals.org/content/37/9/2331.long[↩]
- Fiehler K., Rosler F. (2010). Plasticity of multisensory dorsal stream functions: evidence from congenitally blind and sighted adults. Restor. Neurol. Neurosci. 28, 193–205. 10.3233/RNN-2010-0500. https://www.ncbi.nlm.nih.gov/pubmed/20404408[↩]