Contents
- Copper
- What is the function of copper?
- Copper health benefits
- How much copper do I need?
- Food Sources of Copper
- Copper supplements
- Can copper be harmful?
- Copper and Drug interactions
- Am I getting enough copper?
- What happens if I don’t get enough copper?
- Copper deficiency
Copper
Copper is an essential trace mineral present in all body tissues that is essential for adequate use of iron and zinc, two other essential transition metals by your body 1. Copper is also a cofactor of proteins and enzymes called cuproenzymes (copper-dependent enzymes) that are involved in regulation of iron metabolism, formation of connective tissue, energy (ATP) production at the cellular level, oxygen transportation, formation of blood cells such as red blood cells, platelets, and white blood cells (hematopoiesis), the formation of new blood vessels (angiogenesis), neurohormone homeostasis, the production of melanin (the pigment that produces skin color), the function of the nervous system, brain development, aids in iron absorption, regulation of gene expression, immune system functioning, mitochondrial electron transport and free radical scavenging (redox reactions or oxidation-reduction reactions) 2, 3, 4, 5, 6. Furthermore, defense against oxidative damage depends mainly on the copper-containing superoxide dismutases 7, 8. Copper also helps keep the blood vessels, nerves, immune system, and bones healthy. Copper is vital to the normal function of the hematologic, vascular, skeletal, antioxidant, and neurologic systems 9, 5, 6. Copper is therefore essential for living cells. Both the accumulation of copper and copper deficiency are associated with brain dysfunction.
More recently, new evidence shows that copper is involved in the pathogenesis of neurodegenerative disorders. Copper is an essential transition metal. Copper participates in critical cuproenzymes preventing neurodegeneration and regulating neurotransmission. Via the ferroxidase activity of ceruloplasmin, copper is a metabolic regulator of the contents of iron in the CNS (central nervous system). An excess of free copper is directly involved to neurodegeneration. Wilson’s disease, Alzheimer’s disease and Parkinson’s disease are major neurodegenerative disorders associated with copper dyshomeostasis 10. Wilson disease is a rare inherited disorder that prevents your body from getting rid of extra copper, which can lead to excess storage of copper in the liver, brain, and other organs 11, 12. Copper excess (toxicity) can also occur when a person is exposed to and absorbs large amounts over a short period of time (acute exposure) or various amounts over a long period (chronic exposure).
In the human body, most of the copper (Cu) is present as cuprous (Cu+) and oxidized cupric (Cu2+) compounds 10. Copper is therefore an intermediary for electron transfer in redox reactions (oxidation-reduction reactions and in scavenging free radicals) 13. The oxidation states, Cu3+ and Cu4+, are uncommon 14. Copper is mainly absorbed in the duodenum and proximal jejunum, with a little bit of absorption occurring in the stomach and the distal portion of the small intestine 15. The human copper transport protein 1 (hCTR1), located at the level of enterocytes, transfers the copper ion (Cu+) following the reduction of dietary cupric (Cu2+) into cuprous (Cu+). In hepatocytes, copper binds to metallothioneins, to reduced glutathione or to one of the copper chaperones regulating the traffic of intracellular copper (chaperone for superoxide dismutase 1; SOD1, which is the sole cytosolic cuproenzyme; COX17: chaperone for cytochrome C oxygenase; ATOX1 antioxidant-1: chaperone for the ATPases, ATP7A and ATP7B). The group of transmembrane copper transporters includes CTR1, ATP7A and ATP7B. ATP7A (expressed in the placenta, gut and nervous system) and ATP7B (expressed in the hepatocytes, where it exports copper into the bile and provides copper to nascent ceruloplasmin, and in the nervous system) are linked to the enzyme, tyrosinase, and the ceruloplasmin, respectively. In blood, about 65%–90% of the copper Cu2+ is bound to ceruloplasmin. The remaining 10–35 percent participate in exchanges with albumin, transcuprein, alpha 2 macroglobulin, and low-molecular-weight compounds 16.
Copper is found in many foods including nuts, chocolate, mushrooms, shellfish, whole grains, dried fruits, and liver. Drinking water may acquire copper as it flows through copper pipes, and food may acquire it when people cook or serve food in copper dishes. Normally, the body absorbs copper from food or liquids in the intestines, converts it to a non-toxic form by binding it to a protein, and transports it to the liver. The liver stores some of the copper and binds most of the rest to another protein called apoceruloplasmin to produce the enzyme ceruloplasmin. About 95% of the copper in the blood is bound to ceruloplasmin, and most of the rest is bound to other proteins such as albumin. Only a small amount is normally present in the blood in a free (unbound) state. The liver eliminates excess copper into the bile and it is removed from the body in the stool. Some copper is also eliminated in the urine.
The average daily intake of copper is approximately 1,400 mcg/day for men and 1,100 mcg/day for women, coming mainly from seeds, grains, shellfish, nuts, beans and liver 17, 4, 18, 19, 20, 21. The current recommended dietary intake of copper in the USA is 900 mcg/day 22, 23.
Copper is absorbed mainly from the duodenum and proximal jejunum, and partly from the stomach 24. Low dietary copper intake reduces immune response, which is not restored to normal levels even after several weeks of a high copper intake 25. Only small amounts of copper are typically stored in your body, and the average adult has a total body content of 50–120 mg copper 4, 18. Almost two-thirds of the body’s copper is located in the bone and muscle 4, 22.
Most copper is excreted in bile, and a small amount is excreted in urine. Total fecal losses of copper of biliary origin and nonabsorbed dietary copper are about 1 mg/day 4, 18. Copper levels in your body are homeostatically maintained by copper absorption from the intestine and copper release by the liver into bile to provide protection from copper deficiency and copper toxicity 22.
Copper status is not routinely assessed in clinical practice, and no biomarkers that accurately and reliably assess copper status have been identified 18. Human studies typically measure copper and cuproenzyme activity in plasma and blood cells because individuals with known copper deficiency often have low blood levels of copper and ceruloplasmin (an abundant cuproenzyme) 18. Serum copper and ceruloplasmin (CP) levels may fall to 30% of normal in cases of severe copper deficiency 5. However, plasma ceruloplasmin (CP) and copper levels can be influenced by other factors, such as estrogen status, pregnancy, infection, inflammation, and some cancers 18. Low serum copper level or hypocupremia is also observed in genetic disorders of copper metabolism, including Wilson disease and aceruloplasminemia (a rare inherited autosomal recessive genetic disorder in which a mutation in the ceruloplasmin (CP) gene leads to the absence or dysfunction of ceruloplasmin); however, neither disorder has been linked to low dietary copper intake 5.
Normal serum concentrations are 10–25 micromol/L (63.5–158.9 mcg/dL) for copper and 180–400 mg/L for ceruloplasmin (CP) 26.
Figure 1. Copper metabolism in the brain
Footnote:
(A) A blood vessel (in red on the left), the blood-brain barrier (BBB), the blood-CSF barrier (shown by a rectangle with two compartments), a choroid plexus (CP) and an astrocyte are illustrated;
(B) A neuron, a synapse and an astrocyte are shown. Copper is represented by a green circle. At the blood-CSF barrier, CTR1 (copper transporter 1), DMT1 (divalent metal transporter) and the ATPase, ATP7A, transport copper towards the blood, whereas the ATPase, ATP7B, and CTR1 transport copper in the opposite direction. Organelles and proteins involved in the cellular regulation of copper are represented. CTR1 is the main transporter for transferring copper within cells. Chaperones (ATOX1, COX17, CCS) deliver copper to the ATPases, ATP7A/ATP7B, and to cuproenzymes, including in the mitochondria. Metallothioneins (MT-1, MT-2, MT-3) exert a function as a buffer. Copper is also found in secretory granules.
Abbreviations: APP = amyloid precursor protein; GSH= glutathione; NMDA-R = NMDA receptor; AMPA-R = AMPA receptor; GABAA-R = GABAA receptor; TGN = trans-Golgi network.
[Source 10 ]Free Copper
The fact that cells contain one free copper ion (Cu+) or less highlights the tight regulation 27. The redox (oxidation-reduction reactions) capacities of free copper and its ability to initiate the production of free radicals are two important features. Free copper is a catalyst of the Fenton reaction (Cu+ and H2O2 generate Cu2+ + OH– + OH). The hydrogen peroxide (H2O2) is transformed into the very reactive hydroxyl (OH–) radical, which combines with nucleic acids, proteins and lipids. An increase in free copper is potentially harmful for brain circuits, despite the presence of metallothioneins and chaperones (see below).
Ceruloplasmin
Ceruloplasmin is a multicopper-containing protein mainly synthesized by the liver. Although the rate of synthesis is not influenced by copper intake, ceruloplasmin lacking bound copper has a shorter half-life 28. Ceruloplasmin plays important functions, acting as an iron oxidase, an amine oxidase, an antioxidant and a glutathione peroxidase 29. As a multicopper oxidase, ceruloplasmin reduces dioxygen, O2, to two water molecules. Ceruloplasmin is a scavenger of reactive oxygen species (ROS). It can be considered that the genuine link between copper metabolism and iron metabolism is mediated by ceruloplasmin. Its soluble form controls the oxidation of iron to be included into transferrin. A deficit in copper reduces the ferroxidase activity of ceruloplasmin (Fe2+ to Fe3+). Dietary and recycled iron are in the Fe2+ oxidation state, but iron is transported in serum by transferrin only as Fe3+ after its export by ferroportin 30. Iron itself contributes to the formation of reactive oxygen species (ROS). A deficit of dietary copper leads not only to an accumulation of iron in the liver, but also to an impaired distribution within the spinal cord (see zinc-induced myeloneuropathy in the next section). In the central nervous system (CNS), a glycosylphosphatidylinositol-linked ceruloplasmin bound to the cell membranes is the major isoform of this protein 31. Astrocytes can synthesize their own ceruloplasmin. This glial ceruloplasmin controls also the process of iron oxidation, which allows the clearance of iron from the CNS (central nervous system) 32. Overall, functional ceruloplasmin promotes the synthesis of proteins involved in iron efflux. The maintenance of the iron balance in the brain is thus closely linked to the metabolism of copper.
What is the function of copper?
Your body uses copper to carry out many important functions, including making energy, connective tissues, and blood vessels. Copper also helps maintain the nervous and immune systems, and activates genes 33, 34, 35, 36. Your body also needs copper for brain development. Physiologic functions of these copper-dependent enzymes, and the biochemical pathways in which they function, are outlined below.
Energy production
The copper-dependent enzyme cytochrome C oxidase (CCO) plays a critical role in cellular energy (ATP) production in mitochondria by catalyzing the reduction of molecular oxygen (O2) to water (H2O), thereby generating an electrical gradient that is required for ATP production 37. Redox-active copper contained within the cytochrome C oxidase (CCO) enzyme complex is required for the electron transfer reactions that are critical for its function.
Connective tissue formation
Another cuproenzyme, lysyl oxidase (LOX), is required for the cross-linking of collagen and elastin fibers, which is essential for the formation of strong and flexible connective tissue. Lysyl oxidase (LOX) function is critical for bone formation and maintenance of connective tissue in the heart and blood vessels 38.
Iron metabolism
Multi-copper oxidases (MCOs) are copper-dependent ferroxidases that function in iron homeostasis. Multi-copper oxidases (MCOs) oxidize ferrous iron (Fe2+) to the ferric (Fe3+) form, which enables binding to transferrin (the main iron carrier) in the blood, thus allowing iron transport to sites of utilization (e.g., the bone marrow). The multi-copper oxidases (MCOs) include: (1) ceruloplasmin (CP), which contains 60%-95% of plasma copper; (2) a membrane-bound form of ceruloplasmin (CP) (GPI-CP), expressed in brain and other tissues; and (3) the membrane-bound ferroxidases hephaestin (HEPH) and zyklopen, which function in the intestine and placenta, respectively 39, 40. Ceruloplasmin (CP) knockout (Cp-/-) mice accumulate excess iron in their liver but have normal copper status 41, 42. Similarly, humans with aceruloplasminemia, who lack functional ceruloplasmin (CP), display iron overload in liver, brain, and retina but have no observable defects in copper homeostasis 43. Moreover, absorption of dietary iron and iron mobilization from storage sites (e.g., liver) are impaired in copper deficiency, when ceruloplasmin (CP) and membrane-bound ferroxidases hephaestin (HEPH) activity is reduced, further supporting a role for the multi-copper oxidases (MCOs) in iron metabolism 44.
Central nervous system function
Several physiological processes within the brain and nervous system, including neurotransmitter synthesis and formation and maintenance of myelin, depend upon catalysis mediated by cuproenzymes. Dopamine beta-hydroxylase, for example, catalyzes the conversion of dopamine to the neurotransmitter norepinephrine 45. Also, cytochrome C oxidase (CCO) is required for the biosynthesis of phospholipids, which are critical structural components of the myelin sheath 38.
Melanin biosynthesis
The cuproenzyme tyrosinase (TYR) is required for the biosynthesis of melanin in melanocytes, which is critical for normal pigmentation of hair, skin, and eyes 38. Low tyrosinase (TYR) activity most likely explains the absence or loss of pigmentation in the hair (achromotrichia) seen in copper-deficient laboratory and agricultural animals, and the depigmentation noted in severely copper-depleted patients with Menkes disease.
Antioxidation
Superoxide dismutase (SOD) functions as an antioxidant by catalyzing the conversion of reactive oxygen species, such as the superoxide anion (O2-) and the hydroxyl radical (•OH), to hydrogen peroxide (H2O2), which is subsequently reduced to water by other antioxidant systems 46. Two forms of superoxide dismutase (SOD) contain copper: copper/zinc SOD (SOD1), which is expressed in most cells, including red blood cells; and extracellular SOD (EcSOD), which is highly expressed in the lungs and found at lower levels in plasma 38. Also, ceruloplasmin (CP) has antioxidant properties relating to iron metabolism. The ferroxidase activity of ceruloplasmin (CP) may prevent ferrous iron (Fe2+) from participating in harmful free-radical-generating reactions via Fenton chemistry 46.
Regulation of gene expression
Copper-related gene expression pathways seem to be mainly regulated at a post-translation level, in some cases via protein trafficking-related mechanisms that respond to intracellular copper levels 47. Cytosolic copper may also influence mRNA expression levels of specific genes, in a dose-dependent manner, implicating possible transcriptional regulation 48, 49, 50. For example, intracellular copper may alter the redox state of cells and thus induce oxidative stress, which can activate signal transduction pathways that increase the expression of genes encoding proteins involved in the detoxification of reactive oxygen species 51.
Immune system function
Copper is known to play several important roles in the development and maintenance of immune system function, including innate and adaptive immunity 52. Neutropenia is a clinical sign of copper deficiency in humans. Adverse effects of insufficient copper on immune system function appear most pronounced in infants. For example, infants with Menkes disease, a genetic copper-deficiency disorder, suffer from frequent and severe infections 53, 54. Moreover, in a study of 11 malnourished infants with evidence of copper deficiency, the ability of white blood cells to engulf pathogens increased significantly after one month of copper supplementation 55. Moreover, 11 men on a low-copper diet (660 mcg/day of copper for 24 days and 380 mcg/day for another 40 days) showed an impaired monocyte proliferative response in an ex vivo immune challenge assay 25. Mechanistic studies also support a role for copper in the innate immune response to bacterial and viral infections 56, 57. Severe copper deficiency thus has adverse effects on immune system function; however, whether marginal copper insufficiency impairs immunity in humans has not been established.
Copper health benefits
Cardiovascular disease
Copper deficiency leads to changes in blood lipid levels, a risk factor for atherosclerotic cardiovascular disease 4. Animal studies have shown that copper deficiency is associated with cardiac abnormalities, possibly because of the resulting decreases in the activity of several cardiac cuproenzymes 4, 18.
Observational studies have linked elevated serum copper levels to an increased risk for developing cardiovascular disease. For example, a prospective cohort study examined serum copper levels in more than 4,500 men and women 30 years of age and older in the United States 58. During the subsequent 16 years, 151 participants died from coronary heart disease (coronary artery disease). After adjusting for other risk factors, those with serum copper levels in the two highest quartiles had a significantly greater risk of dying from coronary artery disease. Case-control studies conducted in Europe also had similar outcomes. For example, a case-cohort study of 2,087 adults in Germany reported an association between higher serum copper concentrations and increased risk of incident cardiovascular disease, including myocardial infarction and stroke 59. Another study in 60 patients with chronic heart failure or ischemic heart disease reported that serum copper was a predictor of short-term outcomes 60. Higher serum copper was also linked to an increased risk of heart failure in a prospective cohort study of 1,866 middle-aged and older men in Finland 61. Another prospective cohort study in 4,035 middle-aged men in France reported that high serum copper levels were significantly related to a 50% increase in all-cause mortality; however, serum copper was not significantly associated with cardiovascular disease mortality in this study 62. Serum copper was also elevated in patients with rheumatic heart disease 63. In summary, these studies may indicate that high serum copper reflects elevated body copper content, which increases oxidative stress and accelerates tissue/organ damage and disease development. Importantly, however, most copper in the serum is contained within ceruloplasmin (CP), up to 90% depending upon the species, with the remaining, smaller proportion of serum copper bound to albumin or α2-macroglobulin 64, 65. Serum ceruloplasmin (CP) is an acute-phase reactant protein, with levels increasing by up to 50% as a result of trauma or infection and during chronic inflammatory states. Changes in circulating ceruloplasmin (CP) are associated with proportional changes in serum copper levels, independent of body copper status. Therefore, elevated serum copper in coronary heart disease patients may simply reflect increased ceruloplasmin (CP) production due to the inflammation that typifies atherosclerosis. Collectively, these observations raise concerns about linking elevated serum copper to increased tissue copper content and chronic disease development in humans 66.
In contrast to the observational findings discussed above linking high serum copper levels to heart disease, two autopsy studies found copper levels in cardiac muscle were actually lower in patients who died of coronary heart disease than in those who died of other causes 67. Additionally, the copper content of white blood cells has been positively correlated with the degree of patency of coronary arteries in coronary heart disease patients 68, 69. Furthermore, patients with a history of heart attack (myocardial infarction) had lower concentrations of copper-dependent, extracellular superoxide dismutase than those without a history of heart attack (myocardial infarction) 70. Thus, due to the lack of specific, reliable biomarkers of copper nutritional status, it is not clear whether copper is related to cardiovascular disease. It is also important to note that altered copper metabolism may be symptomatic of a cardiovascular condition, rather than a factor that primarily influences its development.
Studies examining dietary intake of copper are scarce. In a prospective cohort study in Japan, which included 58,646 participants followed for a median of 19 years, dietary copper intake — measured by a food frequency questionnaire — was not associated with coronary heart disease mortality 71. Yet, this study associated higher copper intakes with an increased risk of mortality from stroke and other cardiovascular diseases 71. Notably, it was suggested that elevated plasma copper concentrations could be linked to high circulating homocysteine levels in individuals with cardiovascular disease 72, 73, 74. Increased blood homocysteine may precipitate development of arterial wall lesions and increase risk of cardiovascular disease 75; however, this matter is currently open to debate 76. In animal models, copper-homocysteine interactions were linked to impaired vascular endothelial function 76, 77. Copper restriction in experimental animals decreased homocysteine levels and reduced incidence of atherogenic lesions 78, 79, but it is not known whether copper imbalance contributes to a possible atherogenic effect of homocysteine in humans 80.
Although free copper and ceruloplasmin (CP) can promote low-density lipoprotein (LDL) cholesterol oxidation in the laboratory, there is little evidence that high dietary copper increases oxidative stress in the human body 5. Increased serum copper levels have been associated with increased cardiovascular disease risk, as outlined above, but the significance of these findings is unclear due to the complex association among serum copper, ceruloplasmin (CP), and inflammatory mediators. Clarification of the relationships of copper intake, copper nutritional status, ceruloplasmin (CP) levels, and cardiovascular disease risk thus requires further research.
A few small studies that assessed the impact of copper supplementation in healthy adults have found little evidence that copper supplementation affects cardiovascular disease risk factors 6. For example, daily supplementation with 2 mg copper as copper glycinate for 8 weeks in 70 healthy adults aged 45 to 60 years increased the activity of two cuproenzymes, erythrocyte superoxide dismutase 1 (SOD1) and plasma ceruloplasmin (CP), but had no effect on five other cardiovascular disease-related plasma markers (CRP; homocysteine; and total, high-density lipoprotein (HDL), and low-density lipoprotein (LDL) cholesterol) 81. In 16 healthy women (mean age 24 years), daily supplementation with 3 mg or 6 mg elemental copper as copper sulfate had no significant effect on cardiovascular disease risk factors, including total plasma cholesterol or triacylglycerol concentrations 82. However, the concentration of fibrinolytic factor PAI-1 decreased by about 30% (indicating reduced cardiovascular disease risk) with 6 mg/day copper supplementation compared with placebo. No clinical trials of copper supplementation have been conducted in people with increased cardiovascular disease risk.
Overall, the evidence to date is insufficient to support any conclusions about the association between copper concentrations and cardiovascular disease risk or the impact of copper supplementation on cardiovascular disease 6.
Alzheimer’s disease
Cognitive deterioration in individuals with Alzheimer’s disease is linked to the presence of beta-amyloid plaques and abnormal Tau protein-forming aggregates. The possibility that copper imbalance is involved in the onset of Alzheimer’s disease is under investigation. Some experts believe that dietary copper deficiency plays a role in the cause and pathophysiology of Alzheimer’s disease, the leading cause of dementia, because of several reports of low copper levels and low activity of copper-dependent enzymes in the brains of people with the disease 19, 83. Limited evidence shows that people with higher copper levels have a lower risk of Alzheimer’s disease 84. However, high levels of copper have also been found in the brains of people with Alzheimer’s disease, and some researchers argue that excess amounts of dietary copper are involved in the development of Alzheimer’s disease 85. Furthermore, copper accumulation in damaged brain regions in Alzheimer’s disease might not directly reflect overall body copper status or copper intakes 86.
One study assessed cognitive function using four cognitive tests during home visits every 3 years for 6 years and intakes of copper and saturated and trans fats using a food frequency questionnaire in 3,718 community-dwelling (noninstitutionalized) adults aged 65 and older 87. In the overall study population, dietary and total copper intakes were not associated with cognitive decline. However, in 604 participants (16.2%) who consumed a diet higher in saturated and trans fat, total copper intake in the highest quintile (median 2.75 mg/day) was associated with a significantly faster rate of cognitive decline compared with the lowest intake quintile (median 0.88 mg/day) 87. In contrast, an analysis of data on 1,112 adults older than 60 years found no differences in serum copper or ceruloplasmin (CP) levels between patients with Alzheimer’s disease (n=211) and healthy controls (n=695) 86. This study did reveal, however, a significant decline in serum copper not bound to ceruloplasmin (CP) in patients with mild cognitive impairment or Alzheimer’s disease compared with the healthy control group 18 months after baseline 86.
A few observational studies have assessed the relationship between dietary copper levels and Alzheimer’s disease, with mixed results. Meta-analyses have found that people with Alzheimer’s disease tend to have higher serum copper levels than adults without the disease. In an earlier meta-analysis of 26 studies in a total of 1,058 patients with Alzheimer’s disease and 932 controls, those with Alzheimer’s disease had significantly higher levels of serum copper than the healthy controls 88. In a 2014 meta-analysis of 10 studies in 867 healthy individuals and 599 with Alzheimer’s disease (mean age greater than 70 years in both groups), patients with Alzheimer’s disease had significantly higher serum levels of copper not bound to ceruloplasmin (CP) and total serum copper than healthy controls 89. A recent 2021 meta-analysis of case-control studies described higher blood concentrations of copper in Alzheimer’s disease patients (N=2,929) as compared to healthy subjects (N=3,547), from a total of 46 studies reviewed 90. Also, ‘free’ serum copper (i.e., not bound to ceruloplasmin [CP]) was higher in Alzheimer’s disease patients (N=1,595) than in healthy control subjects (N=2,399), representing 18 total studies. These observations were confirmed in another recent review of published studies 91. An additional meta-analysis of 12 case-control studies revealed Alzheimer’s disease patients had lower copper concentrations in various brain regions compared to healthy controls, further exemplifying dysregulation of copper homeostasis in Alzheimer’s disease 92.
Very little clinical evidence is available on the impact of copper supplementation in patients with Alzheimer’s disease. One clinical trial that randomly assigned 68 patients aged 50 to 80 years with mild Alzheimer’s disease to supplementation with 8 mg copper daily or placebo for 12 months found no significant differences in cognition between groups 93.
Experts participating in the 2013 International Conference on Nutrition and the Brain suggested that individuals at increased risk of Alzheimer’s disease using multivitamin-mineral supplements choose those that have no copper (or iron) because excessive intakes of these minerals could contribute to cognitive issues in some patients 94. However, much more research is needed to determine whether high or low levels of serum or plasma copper are associated with Alzheimer’s disease risk and whether supplements containing copper could affect Alzheimer’s disease risk or symptoms.
Additional research is required to determine whether genetic variation could influence individual susceptibility to environmental exposure of high copper levels. Copper administered in drinking water was associated with development of enhanced pathological features in animal models of Alzheimer’s disease 95, 96. One study in a rabbit model reported that combining a high-cholesterol diet and copper (120 mcg/L in drinking water) impaired cognition 95. A prospective cohort study in 3,718 elderly participants in the Chicago Health and Aging Project, followed for 5.5 years, evaluated the impact of fat and copper intakes using food frequency questionnaires on cognitive function. For individuals with high intakes of saturated and trans fat, cognitive decline was greater for those in the highest quintile of total copper intake compared to the lowest quintile (median intake of 2.75 vs. 0.88 mg copper per day) 87.
Although dysfunctional copper metabolism is suggested as a risk factor for Alzheimer’s disease, it could also be symptomatic of the disease, rather than causative. Moreover, it is still unclear whether copper supplementation or restriction could delay the progression of Alzheimer’s disease. A small, double-blind, placebo-controlled trial in 68 individuals with mild Alzheimer’s disease found that supplementation of 8 mg/day of copper for one year delayed the decrease of the beta-amyloid peptide Aβ42 in cerebrospinal fluid; a decrease in Aβ42 has been linked to cognitive deterioration 97. This delay, however, was not associated with improved cognitive performance 93. Relating to the use of zinc supplementation to block copper absorption in Wilson disease, slow-release zinc acetate administration (150 mg/day for six months) in a randomized, placebo-controlled study of 60 patients with mild-to-moderate Alzheimer’s disease resulted in a decrease in serum ‘free’ copper and stabilization of cognition deficits 98. A specific role for copper was not, however, determined in these notable outcomes. In summary, additional human studies are needed to clarify the role of copper in Alzheimer’s disease prevention, development, and progression.
Parkinson’s disease
Neurologically presenting Wilson disease and inherited aceruloplasminemia are characterized by copper accumulation in the brain and development of neurological symptoms, including dystonia and cognitive impairment, that resemble Parkinson’s disease 99. Disruption of copper homeostasis has been documented in Parkinson’s disease 100. Copper depletion occurs in brain regions with loss of neurons in Parkinson’s disease patients 101, 102. Furthermore, some studies have documented lower serum copper and/or ceruloplasmin (CP) concentrations in Parkinson’s disease patients compared to healthy controls 103, 104, 105. Dietary copper intake did not, however, relate to the risk of developing Parkinson’s disease in two small case-control studies 106, 107. As in Alzheimer’s disease, further research is required to elucidate whether copper imbalance contributes to the pathogenesis of Parkinson’s disease.
Osteoporosis
Progressive decrease of bone mineral density (BMD) is commonly observed in the elderly, frequently leading to development of osteopenia (pre-osteoporosis) and osteoporosis 5. Women are more often affected by osteoporosis than men, (e.g., prevalence ratio is 5:1 in non-Hispanic whites) 108, primarily due to the postmenopausal reduction in the production of estrogen, which is essential for maintaining strength of muscle, bone, and connective tissue 109. Osteoporosis is associated with an increased risk of falls, bone fracture, and mortality in individuals over 65 years of age 110.
Osteoporosis has been reported in infants with severe copper deficiency, but how copper depletion affects bone and connective tissue health in adults is less certain 111, 112. One recent investigation documented bone resorption (breakdown) in 11 healthy adult males consuming marginal copper for six weeks (700 mcg/day) 113. Also, supplementation of 3 to 6 mg/day of copper for six weeks had no effect on biochemical markers of bone resorption or bone formation in two studies of healthy adult men and women 114, 115. An effect of copper deficiency on bone integrity seems likely, since a copper-dependent enzyme, lysyl oxidase (LOX), is required for the maturation (cross-linking) of collagen, a key element in the organic matrix of bone. In individuals with marginal copper intake and less efficient copper absorption, such as the elderly, it seems plausible that lysyl oxidase (LOX) activity is decreased, possibly increasing risk for bone and connective tissue effects.
Limited studies of copper supplementation and bone health outcomes have been undertaken. A small study in perimenopausal women, who consumed ~1 mg of dietary copper daily, reported decreased loss of bone mineral density (BMD) from the lumbar spine after copper supplementation of 3 mg/day for two years 116. Additionally, a two-year, double-blind, placebo-controlled trial in 59 postmenopausal women found that daily intake of supplemental calcium plus trace minerals, including 2.5 mg of copper, resulted in maintenance of spinal bone mineral density (BMD) 117. Supplemental calcium or trace minerals, alone, were not as effective at preventing loss of bone density 117. Another randomized, double-blind, placebo-controlled study in 224 healthy, postmenopausal women ages 51 to 80 years, found daily supplementation with 600 mg of calcium, 2 mg of copper, and 12 mg of zinc for two years decreased whole-body BMD compared to supplemental calcium alone. Another trial showed that bone mineral density (BMD) was reduced in subjects with dietary copper intakes below the RDA (900 mcg/day), but copper supplementation did not prevent the progressive loss of BMD as well as a calcium regimen alone 118. Finally, several studies have suggested that tooth loss might be related to defects in the maintenance of bone mineral density (BMD) 119, 120. When compared with 20 healthy-matched controls, 50 patients (mean age, 47.5 years) with low spinal bone mineral density (BMD) and advanced tooth wear were found to have significantly lower copper content in tooth enamel. However, despite evidence of bone demineralization, serum copper levels in this population were similar to those of the healthy group 121. In summary, additional research is required to draw meaningful conclusions regarding the effects of marginal copper depletion and copper supplementation on bone metabolism and risk for developing age-related osteoporosis.
Nonalcoholic fatty liver disease (NAFLD)
Similar to findings from animal models 122, human studies have documented low circulating copper 123, 124 or low hepatic copper content in adults and children with nonalcoholic fatty liver disease (NAFLD) 125, 126. An inverse association between hepatic copper content and liver disease severity has also been observed 125, 126. However, it is not known whether low dietary intake of copper might be a contributor to disease pathogenesis or whether dysregulated copper metabolism is only a manifestation of liver disease.
How much copper do I need?
The amount of copper you need each day depends on your age. Average daily recommended amounts are listed below in micrograms (mcg).
Intake recommendations for copper and other nutrients are provided in the Dietary Reference Intakes (DRIs) developed by the Food and Nutrition Board (FNB) at the National Academies of Sciences, Engineering, and Medicine 22. Dietary Reference Intake (DRI) is the general term for a set of reference values used for planning and assessing nutrient intakes of healthy people. These values, which vary by age and sex, include:
- Recommended Dietary Allowance (RDA): Average daily level of intake sufficient to meet the nutrient requirements of nearly all (97%–98%) healthy individuals; often used to plan nutritionally adequate diets for individuals.
- Adequate Intake (AI): Intake at this level is assumed to ensure nutritional adequacy; established when evidence is insufficient to develop an RDA.
- Estimated Average Requirement (EAR): Average daily level of intake estimated to meet the requirements of 50% of healthy individuals; usually used to assess the nutrient intakes of groups of people and to plan nutritionally adequate diets for them; can also be used to assess the nutrient intakes of individuals.
- Tolerable Upper Intake Level (UL): Maximum daily intake unlikely to cause adverse health effects.
According to the National Health and Nutrition Examination Survey (NHANES), the mean dietary intake of copper in the US is 1100 mcg/day for adult women and 1300 mcg/day for adult men, levels that exceed the established Recommended Dietary Allowance (RDA) for copper for adults of 900 mcg/day (see Table 1) 127.
Table 1 lists the current Recommended Dietary Allowance (RDA) for copper 22. For infants from birth to 12 months, the Food and Nutrition Board established an Adequate Intake (intake at this level is assumed to ensure nutritional adequacy; established when evidence is insufficient to develop an RDA). for copper that is equivalent to the mean intake of copper in healthy, breastfed infants.
Table 1. Recommended Dietary Allowances (RDAs) for Copper
Life Stage | Recommended Amount |
---|---|
Birth to 6 months | 200 mcg |
Infants 7–12 months* | 220 mcg |
Children 1–3 years | 340 mcg |
Children 4–8 years | 440 mcg |
Children 9–13 years | 700 mcg |
Teens 14–18 years | 890 mcg |
Adults 19 years and older | 900 mcg |
Pregnant teens and women | 1,000 mcg |
Breastfeeding teens and women | 1,300 mcg |
Footnote: *Adequate Intake (AI) = intake at this level is assumed to ensure nutritional adequacy; established when evidence is insufficient to develop an Recommended Dietary Allowance (RDA).
[Source 6 ]Food Sources of Copper
Many foods contain copper. You can get recommended amounts of copper by eating a variety of foods, including the following 128:
- Beef liver and shellfish such as oysters
- Nuts (such as cashews), seeds (such as sesame and sunflower), and chocolate
- Wheat-bran cereals and whole-grain products
- Potatoes, mushrooms, avocados, chickpeas, and tofu
The estimated copper content of some foods that are relatively rich in copper is listed in Table 2.
For more information on the nutrient content of foods, search USDA’s FoodData Central.
Typical diets in the United States meet or exceed the copper Recommended Dietary Allowance (RDA). Mean dietary intakes of copper from foods range from 800 to 1,000 mcg per day for children aged 2–19 17. In adults aged 20 and older, average daily intakes of copper from food are 1,400 mcg for men and 1,100 mcg for women. Total intakes from supplements and foods are 900 to 1,100 mcg/day for children and 1,400 to 1,700 mcg/day for adults aged 20 and over.
Table 2. Selected Foods Copper Content
Food | Micrograms (mcg) per serving | Percent DV* |
---|---|---|
Beef, liver, pan fried (3 ounces) | 12400 | 1378 |
Oysters, eastern, wild, cooked, 3 ounces | 4850 | 539 |
Baking chocolate, unsweetened, 1 ounce | 938 | 104 |
Potatoes, cooked, flesh and skin, 1 medium potato | 675 | 75 |
Mushrooms, shiitake, cooked, cut pieces, ½ cup | 650 | 72 |
Cashew nuts, dry roasted, 1 ounce | 629 | 70 |
Crab, Dungeness, cooked, 3 ounces | 624 | 69 |
Sunflower seed kernels, toasted, ¼ cup | 615 | 68 |
Turkey, giblets, simmered, 3 ounces | 588 | 65 |
Chocolate, dark, 70%-85% cacao solids, 1 ounce | 501 | 56 |
Tofu, raw, firm, ½ cup | 476 | 53 |
Chickpeas, mature sees, ½ cup | 289 | 32 |
Millet, cooked, 1 cup | 280 | 31 |
Salmon, Atlantic, wild, cooked, 3 ounces | 273 | 30 |
Pasta, whole wheat, cooked, 1 cup (not packed) | 263 | 29 |
Avocado, raw, ½ cup | 219 | 24 |
Figs, dried, ½ cup | 214 | 24 |
Spinach, boiled, drained, ½ cup | 157 | 17 |
Asparagus, cooked, drained, ½ cup | 149 | 17 |
Sesame seeds, ¼ cup | 147 | 16 |
Turkey, ground, cooked, 3 ounces | 128 | 14 |
Cereals, Cream of Wheat, cooked with water, stove-top, 1 cup | 104 | 12 |
Tomatoes, raw, chopped, ½ cup | 53 | 6 |
Yogurt, Greek, plain, lowfat, 7-ounce container | 42 | 5 |
Milk, nonfat, 1 cup | 27 | 3 |
Apples, raw, with skin, ½ cup slices | 17 | 2 |
Footnote: *DV = Daily Value. The U.S. Food and Drug Administration (FDA) developed Daily Values (DVs) to help consumers compare the nutrient contents of foods and dietary supplements within the context of a total diet. The Daily Value (DV) for copper is 0.9 mg (900 mcg) for adults and children age 4 years and older 129. The FDA does not require food labels to list copper content unless copper has been added to the food. Foods providing 20% or more of the DV are considered to be high sources of a nutrient, but foods providing lower percentages of the DV also contribute to a healthful diet.
[Source 6 ]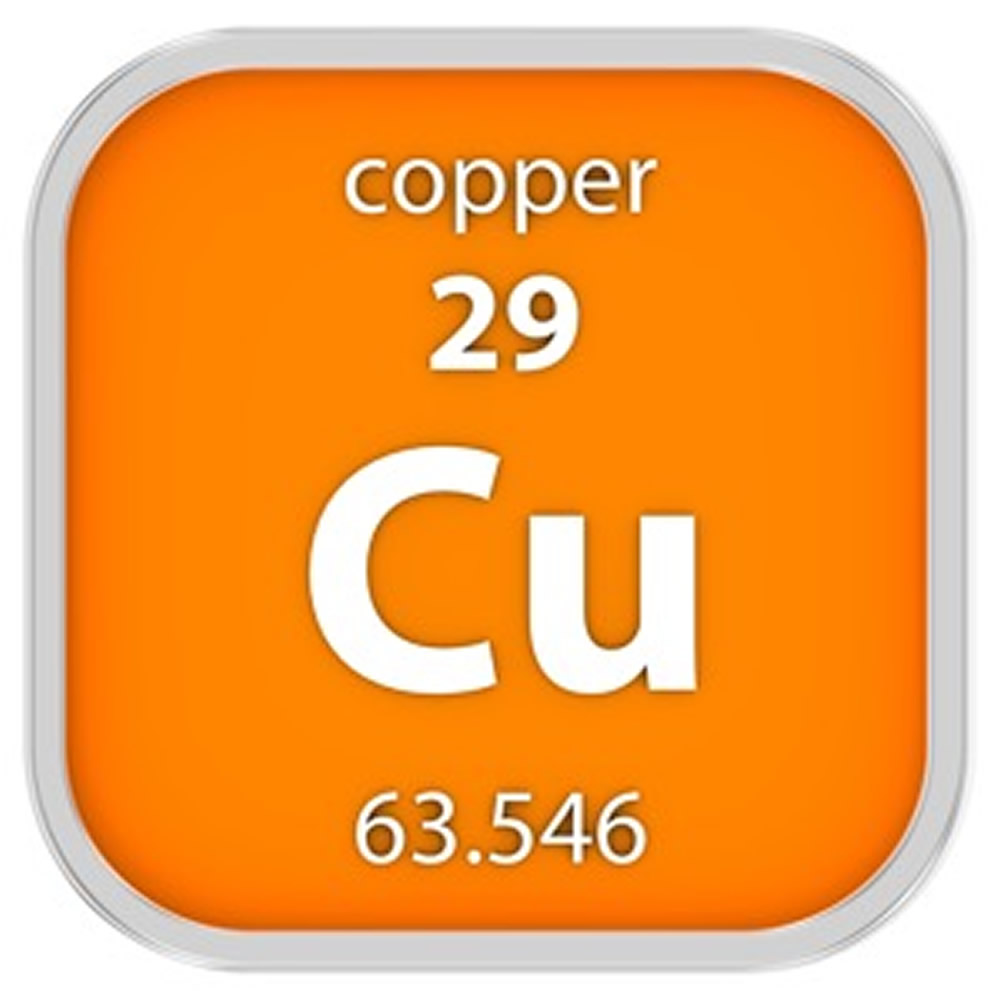
Copper supplements
Copper is available in many multivitamin or multimineral supplements, in supplements that contain only copper, and in other dietary supplements in combination with other ingredients. Copper in dietary supplements is often in the forms of cupric oxide, cupric sulfate, copper amino acid chelates, and copper gluconate. To date, no studies have compared the bioavailability of copper from these and other forms 130. The amount of copper in dietary supplements typically ranges from a few micrograms to 15 mg (about 17 times the Daily Value [DV] for copper) 6.
Supplementation of adults with 10 mg/day of cupric gluconate for 12 weeks did not cause copper toxicity 131. Importantly, however, higher copper intakes could be detrimental in some at-risk (unknown) individuals. Copper supplementation of infants should be approached with caution since homeostatic regulators of copper absorption and excretion are not fully developed, thus increasing the potential for copper toxicosis. From a clinical perspective, copper overload most frequently presents in biliary atresia, biliary cirrhosis and in Wilson’s disease patients, and as such, individuals suffering from these conditions should avoid taking supplemental copper.
According to an analysis of data from the 2009–2012 National Health and Nutrition Survey (NHANES), 6% to 15% of adults aged 19 and older who do not take dietary supplements containing copper have copper intakes below the Estimated Average Requirement (average daily level of intake estimated to meet the requirements of 50% of healthy individuals; usually used to assess the nutrient intakes of groups of people and to plan nutritionally adequate diets for them; can also be used to assess the nutrient intakes of individuals) 132. In those who do use supplements, rates of adults with intakes below the copper Estimated Average Requirement (EAR) range from 2.2% to 7.2% 132.
Copper IUDs versus long-acting hormone injections and implants for contraception
Reversible, long-term contraception is relied on by millions of women to prevent unwanted pregnancy. Two very common methods of pregnancy prevention are the use of a copper-containing intrauterine device (IUD) or an injection of a progestogen hormone.
According to this (source 133) the effectiveness of copper-containing intra-uterine devices (coil) specifically the T-shaped IUDs with copper on the arms are the most effective, have the longest duration of action and are the IUDs of choice. TCu380A or TCu380S appear to be more effective than other IUDs. No IUD showed consistently lower removal rates for bleeding and pain in comparison to other IUDs. There is no evidence that any particular framed copper device is better suited to women who have not had children.
In the populations studied (studies that compared these two highly effective methods and found the IUD to be better at preventing pregnancy than depot medroxyprogesterone acetate) (source 134), the IUD was more effective than hormonal contraception with respect to pregnancy prevention.
The copper intrauterine device (copper IUD) is a highly-effective non-hormonal type of birth control, and is the most commonly used method in the world. However, use of the copper IUD is low in countries with relatively high rates of unintended pregnancy, such as the United Kingdom and United States. Since the copper IUD is one of the most effective reversible contraceptive methods, primary care and family planning and practitioners could consider adopting these interventions 135.
Can copper be harmful?
Yes, copper can be harmful if you get too much. Getting too much copper on a regular basis can cause liver damage, abdominal pain, cramps, nausea, diarrhea, and vomiting 26. More serious signs of acute copper toxicity include severe liver damage, kidney failure, coma, and death 5. Copper toxicity is rare in healthy individuals who do not have a hereditary copper homeostasis defect (e.g., Wilson’s disease, a rare genetic disorder). Wilson’s diseaseis a rare, autosomal recessive disease, which is caused by a mutation in ATP7B, leads to abnormally high tissue levels of copper as a result of defective copper clearance 136. People with Wilson’s disease can develop neurologic and liver damage that can result in cirrhosis 4. Patients can also develop acute hepatitis, hemolytic crisis, and liver failure. Lifelong copper chelation therapy or high doses of zinc can prevent permanent organ damage in these patients.
Copper toxicity has been reported in people who consume water containing high levels of copper as a result of stagnant water in copper-containing pipes and fixtures as well as copper alloys in water distribution systems and household plumbing that allow copper to leach into water 26, 137. The Environmental Protection Agency has established a recommended upper limit for copper in public water systems of 1.3 mg/L 137, 138 and by the World Health Organization (2 mg/liter) 139.
The daily upper limits (ULs) for copper include intakes from all sources—food, beverages, and supplements—and are listed below in micrograms (mcg). The Food and Nutrition Board has established Tolerable Upper Intake Level (maximum daily intake unlikely to cause adverse health effects) for copper from food and supplements for healthy individuals based on levels associated with liver damage 26. The Tolerable Upper Intake Levels (ULs) do not apply to individuals who are receiving supplemental copper under medical supervision.
Table 3. Tolerable Upper Intake Levels (ULs) for Copper
Age | Male | Female | Pregnancy | Lactation |
---|---|---|---|---|
Birth to 6 months | None established* | None established* | ||
7–12 months | None established* | None established* | ||
1–3 years | 1,000 mcg | 1,000 mcg | ||
4–8 years | 3,000 mcg | 3,000 mcg | ||
9–13 years | 5,000 mcg | 5,000 mcg | ||
14–18 years | 8,000 mcg | 8,000 mcg | 8,000 mcg | 8,000 mcg |
19+ years | 10,000 mcg | 10,000 mcg | 10,000 mcg | 10,000 mcg |
Footnote: * Breast milk, formula, and food should be the only sources of copper for infants.
[Source 6 ]Copper Toxicosis
The symptoms of acute copper intoxication include nausea, vomiting, diarrhea, abdominal pain, tachycardia and hemolytic anemia. The intoxication usually follows the ingestion of contaminated water or food 140.
The daily intake of copper determines the occurrence of copper toxicosis. High levels of copper in drinking water and food can cause a subacute to chronic syndrome of copper toxicosis 141. The current tolerable upper intake level is 10 mg/day 142. An oral supplement of copper 10 mg/day (supplementation with plain tap water between meals, consisting of two 5-mg copper gelatin capsules as copper sulfate) during two months induces a transient, but significant, elevation of aminotransferases 143. Drinking water from wells via copper pipes has been incriminated in copper toxicosis 144. The syndrome affects mainly young children. Their parents may be clinically healthy 144. The disorder can lead to death. In India, a micronodular cirrhosis has been reported 145. Copper toxicosis may be idiopathic, but these cases are very rare.
Rats exposed to copper in drinking water develop liver damage due to increased hepatocellular stores, with a significant increase of concentrations of the metal in the CNS and biochemical evidence of oxidative stress 146. The activity of superoxide dismutase (SOD) decreases, and the concentrations of malondialdehyde rise.
Copper and Drug interactions
Relatively little is known about the interaction of copper with drugs. Penicillamine is used to bind copper and enhance its elimination in Wilson disease, a genetic disorder resulting in hepatic copper overload. Because penicillamine dramatically increases the urinary excretion of copper, individuals taking the medication for reasons other than copper overload may have an increased dietary copper requirement. Additionally, antacids may interfere with copper absorption when used in very high amounts 38. Also, the anti-tuberculosis drug ethambutol may chelate copper in mitochondria and reduce cytochrome c oxidase activity specifically in optic nerve axons, possibly contributing to optic neuropathy which is a documented side-effect of this drug 147.
Am I getting enough copper?
Most people get enough copper from the foods they eat. However, certain groups of people are more likely than others to have trouble getting enough copper:
- People with celiac disease
- People with Menkes disease, a rare genetic disorder
- People taking high doses of zinc supplements, which can interfere with the ability to absorb copper and could lead to copper deficiency
What happens if I don’t get enough copper?
Copper deficiency is rare in the United States. Copper deficiency can cause extreme tiredness, lightened patches of skin, high levels of cholesterol in the blood, and connective tissue disorders affecting the ligaments and skin. Other effects of copper deficiency are weak and brittle bones, loss of balance and coordination, and increased risk of infection.
Copper deficiency
Copper deficiency or frank dietary copper deficiency is less common in healthy individuals because copper is a ubiquitous element and is present in almost all foods 148, 149, 18. Copper deficiency in the United States is believed to be relatively rare but has been described in the setting of zinc supplementation 150, myelodysplastic syndrome 151, use of total parenteral nutrition (TPN) 152 and chronic tube feeding 153 especially via jejunostomy 154 and in various malabsorption syndromes such as cystic fibrosis, celiac disease and in people who’ve had gastrectomy and roux-en-Y gastric bypass surgery for obesity and in infants exclusively fed cow-milk formulas 9. A rare X-linked genetic condition called Menkes kinky hair syndrome or Menkes disease is caused by a defect in the ATP7A gene 155, 156. The defect makes it hard for the body to properly distribute (transport) copper throughout the body. As a result, the brain and other parts of the body do not get enough copper, while it builds up in the small intestine and kidneys of affected infants. Menkes disease, which affects primarily males, is associated with seizures, delayed development, abnormal artery development in the brain, and unusual gray brittle kinky hair 156.
Based on studies in animals and humans, the effects of copper deficiency include anemia, hypopigmentation (a low amount of melanin in your skin), high blood cholesterol (hypercholesterolemia), connective tissue disorders, osteoporosis (condition in which bones become weak and brittle) and other bone defects, abnormal lipid metabolism, a lack of coordination or unsteadiness (ataxia), and increased risk of infection 4, 157, 158. Features of copper deficiency include hematologic abnormalities (anemia, leukopenia, neutropenia, and thrombocytopenia) and myeloneuropathy (spinal cord and peripheral nerves damage in the lower limbs); the latter is a rarer and often unrecognized complication of copper deficiency 159, 149, 9. A low copper level can affect the structure of bone, skin, hair, and blood vessels, and interfere with nerve function. Most importantly, the neurologic complications of copper deficiency may be irreversible, making early diagnosis and prompt treatment essential to successful outcomes.
One of the most common clinical signs of copper deficiency is an anemia that is unresponsive to iron therapy but is corrected by copper supplementation 5. It was hypothesized that this copper-deficiency anemia could result from defective iron mobilization due to decreased ceruloplasmin (CP) activity, yet individuals with inherited aceruloplasminemia (a rare inherited autosomal recessive genetic disorder in which a mutation in the ceruloplasmin (CP) gene leads to the absence or dysfunction of ceruloplasmin) do not always develop overt anemia 160. Ceruloplasmin (CP) plays a role in iron metabolism and carries more than 95% of the total copper in healthy human plasma 161. In copper-deficient pig, intestinal iron absorption is impaired but iron distribution among tissues or organs is normal 162, 163, 164. Low serum iron from reduced absorption is an unlikely cause of this anemia since intravenous provision of iron did not correct it 5. An alternative hypothesis is that copper-deficiency anemia is caused principally by impaired hemoglobin production and red blood cell proliferation, and a shortened red blood cell lifespan. These physiological processes are thus likely to require copper. Copper deficiency may also lead to neutropenia, which can increase susceptibility to infection. Copper depletion studies demonstrated that low copper might affect erythroid and myeloid cell lineages, supporting a role for copper in the regulation of blood cell proliferation and maturation 165, 166. More research is clearly needed to further define the mechanisms underlying copper deficiency-induced anemia and neutropenia 165, 33. Furthermore, osteoporosis and other abnormalities of bone development have been described in copper-deficient, low-birth-weight infants and young children. Less common features of copper deficiency may include impaired growth, depigmentation, and development of neurological pathologies 38, 37.
Copper is an essential trace mineral present in all body tissues that is essential for adequate use of iron and zinc, two other essential transition metals by your body 1. Copper is also a cofactor of proteins and enzymes called cuproenzymes (copper-dependent enzymes) that are involved in regulation of iron metabolism, formation of connective tissue, energy (ATP) production at the cellular level, oxygen transportation, formation of blood cells such as red blood cells, platelets, and white blood cells (hematopoiesis), the formation of new blood vessels (angiogenesis), neurohormone homeostasis, the production of melanin (the pigment that produces skin color), the function of the nervous system, brain development, aids in iron absorption, regulation of gene expression, immune system functioning, mitochondrial electron transport and free radical scavenging (redox reactions or oxidation-reduction reactions) 2, 3, 4, 5, 6. Furthermore, defense against oxidative damage depends mainly on the copper-containing superoxide dismutases 7, 8. Copper also helps keep the blood vessels, nerves, immune system, and bones healthy. Copper is vital to the normal function of the hematologic, vascular, skeletal, antioxidant, and neurologic systems 9, 5, 6. Copper is therefore essential for living cells. Both the accumulation of copper and copper deficiency are associated with brain dysfunction.
More recently, new evidence shows that copper is involved in the pathogenesis of neurodegenerative disorders. Copper is an essential transition metal. Copper participates in critical cuproenzymes preventing neurodegeneration and regulating neurotransmission. Via the ferroxidase activity of ceruloplasmin, copper is a metabolic regulator of the contents of iron in the CNS (central nervous system). An excess of free copper is directly involved to neurodegeneration. Wilson’s disease, Alzheimer’s disease and Parkinson’s disease are major neurodegenerative disorders associated with copper dyshomeostasis 10. Wilson disease is a rare inherited disorder that prevents your body from getting rid of extra copper, which can lead to excess storage of copper in the liver, brain, and other organs 11, 12. Copper excess (toxicity) can also occur when a person is exposed to and absorbs large amounts over a short period of time (acute exposure) or various amounts over a long period (chronic exposure).
Copper is found in many foods including nuts, chocolate, mushrooms, shellfish, whole grains, dried fruits, and liver. Drinking water may acquire copper as it flows through copper pipes, and food may acquire it when people cook or serve food in copper dishes. Normally, the body absorbs copper from food or liquids in the intestines, converts it to a non-toxic form by binding it to a protein, and transports it to the liver. The liver stores some of the copper and binds most of the rest to another protein called apoceruloplasmin to produce the enzyme ceruloplasmin. About 95% of the copper in the blood is bound to ceruloplasmin, and most of the rest is bound to other proteins such as albumin. Only a small amount is normally present in the blood in a free (unbound) state. The liver eliminates excess copper into the bile and it is removed from the body in the stool. Some copper is also eliminated in the urine.
The average daily intake of copper is approximately 1,400 mcg/day for men and 1,100 mcg/day for women, coming mainly from seeds, grains, shellfish, nuts, beans and liver 17, 4, 18, 19, 20, 21. The current recommended dietary intake of copper in the USA is 900 mcg/day 22, 23.
Copper is absorbed mainly from the duodenum and proximal jejunum, and partly from the stomach 24. Low dietary copper intake reduces immune response, which is not restored to normal levels even after several weeks of a high copper intake 25. Only small amounts of copper are typically stored in your body, and the average adult has a total body content of 50–120 mg copper 4, 18. Almost two-thirds of the body’s copper is located in the bone and muscle 4, 22.
Most copper is excreted in bile, and a small amount is excreted in urine. Total fecal losses of copper of biliary origin and nonabsorbed dietary copper are about 1 mg/day 4, 18. Copper levels in your body are homeostatically maintained by copper absorption from the intestine and copper release by the liver into bile to provide protection from copper deficiency and copper toxicity 22.
Copper status is not routinely assessed in clinical practice, and no biomarkers that accurately and reliably assess copper status have been identified 18. Human studies typically measure copper and cuproenzyme activity in plasma and blood cells because individuals with known copper deficiency often have low blood levels of copper and ceruloplasmin (an abundant cuproenzyme) 18. Serum copper and ceruloplasmin (CP) levels may fall to 30% of normal in cases of severe copper deficiency 5. However, plasma ceruloplasmin (CP) and copper levels can be influenced by other factors, such as estrogen status, pregnancy, infection, inflammation, and some cancers 18. Low serum copper level or hypocupremia is also observed in genetic disorders of copper metabolism, including Wilson disease and aceruloplasminemia (a rare inherited autosomal recessive genetic disorder in which a mutation in the ceruloplasmin (CP) gene leads to the absence or dysfunction of ceruloplasmin); however, neither disorder has been linked to low dietary copper intake 5.
Normal serum concentrations are 10–25 micromol/L (63.5–158.9 mcg/dL) for copper and 180–400 mg/L for ceruloplasmin (CP) 26.
The treatment for copper deficiency is not yet developed and depends on the underlying cause of the copper deficiency 159. However, it is easy to consume food containing high amounts of copper as a copper supplement. Commercially available pure cocoa contains approximately 4 mg copper per 100 g and is easy to administer to tube‐feeding patients because it is in powder form. Experts recommended other copper‐rich foods such as nuts, shellfish, or liver to patients with diabetes mellitus because almost all patients add sugar to suppress the bitterness of cocoa. Experts also used other copper‐rich foods for patients who could not continue oral cocoa intake due to diarrhea because cocoa contains fibers 167. For individuals with Menkes kinky hair syndrome or Menkes disease, there is no cure at this time, but treatment with parenteral copper histidinate (CuHis) can increase survival and lessen the neurological symptoms if initiated early, within approximately 28 days following birth 168.
Copper deficiency causes
Upper gastrointestinal surgery
Certain types of upper gastrointestinal surgery can reduce the effective absorption area for particular micronutrients. Deficiencies of iron and vitamin B12 are especially common 169, with a lack of intrinsic factor contributing to the latter. Whilst neurological complications of gastrectomy for peptic ulcer disease have long been recognized, the underlying aetiology often remained obscure 170. The association between a remote history of upper gastrointestinal surgery, copper deficiency and myelopathy was first described in 2001 171, and has since been increasingly reported following both non-bariatric 172 and bariatric intervention 173.
Partial gastrectomy has rarely been used to treat peptic ulcer disease since the advent of effective pharmacological therapy. The number of cases of gastrectomy-related copper deficiency is small relative to the number of past procedures. However, it is likely that many further cases will be diagnosed because undiagnosed hypocupraemia is common in patients with a history of gastrectomy 174, copper deficiency may manifest over 40 years after the operation 175 and awareness amongst physicians continues to increase.
The annual number of bariatric operations continues to grow, with the commonest procedure being a Roux-en-Y gastric bypass. Post-operatively, food bypasses most of the stomach and the entire duodenum. Copper deficiency tends to develop sooner after bariatric than after non-bariatric operations, probably due to the greater reduction in the effective absorption area for copper. Case numbers of copper deficiency will rise with the growing use of bariatric surgery and with the ageing of the present cohort.
Malabsorption
In half the cases of copper deficiency attributed to malabsorption, celiac disease was diagnosed; in the other half, no cause of malabsorption was established. In three cases of idiopathic copper malabsorption, no detailed investigation findings were reported 170. In the fourth case, malabsorption was not specific to copper: the patient was cachectic and exhibited concomitant deficiencies of iron, vitamin D and vitamin E. A D-xylose absorption test was compatible with proximal intestinal malabsorption, but upper gastrointestinal endoscopy, duodenal biopsies and faecal microbiology failed to identify an underlying cause 176.
Drug-induced copper deficiency
Rosa 177 reviewed a series of cases of infants born to women who received d-penicillamine during pregnancy for a variety of conditions, including connective-tissue abnormalities and rheumatoid arthritis. Abnormalities observed in the infants included lax skin, hyperflexibility of the joints, fragility of the veins, and numerous soft tissue abnormalities. It was suggested that the malformations were in part due to a drug-induced copper deficiency during embryonic or fetal development. Similar abnormalities were produced with d-penicillamine in rodent models 178, and the teratogenicity of the drug can be modulated by maternal dietary copper intake 179. The above suggest that in humans maternal copper status should be monitored when d-penicillamine is taken during pregnancy.
Little is known about the influence of other copper chelating drugs such as captopril, disulfiram, dimercaptosuccinic acid, and triethylenetetramine on human prenatal development. Given the significant teratogenic effects that can be associated with similar drugs in experimental animals, it is reasonable to suggest that these drugs can pose a substantial risk to the conceptus if maternal dietary copper intake is low.
A condition of severe copper deficiency can be rapidly induced in experimental animals through the use of a number of chelating drugs, including disulfiram, D-penicillamine, triethylenetetramine, and dimercaptosuccinic acid 180. Each of those drugs is known to be teratogenic. The abnormalities produced are reminiscent of those induced by dietary copper deficiency. Although the teratogenicity of D-penicillamine, triethylenetetramine, and dimercaptosuccinic acid can be modulated by the amount of copper in the mother’s diet (Cohen et al. 1983; Mark-Savage et al. 1983), it is important to note that drugs that bind copper typically also bind zinc. Thus, the teratogenic effects of those drugs might be due to a combination of copper and zinc deficiencies.
In contrast to the effects of zinc, drugs and chemicals that induce an acute-phase response in the mother do not necessarily influence copper uptake by the embryo or fetus, and in most cases, fetal copper concentrations are unaffected by chemicals that induce transitory acute-phase responses 181. That is not surprising, because maternal plasma copper concentrations are increased during an acute-phase response (due to the hepatic production and release of the cuproenzyme ceruloplasmin), and zinc concentrations are decreased. Ceruloplasmin has been postulated to be involved directly in copper transport to the embryo and fetus 182. It must be noted that the mechanisms underlying copper transport into the embryo and fetus is an area of active research.
Disease-induced copper deficiency
Copper deficiency can occur secondarily to such diseases as chronic diarrhea, diabetes, alcoholism, and hypertension 183. In the case of maternal diabetes and alcoholism, disease-induced deficiencies of copper in the embryo or fetus have been postulated to contribute to the teratogenesis 184. Mothers with diabetes are reported to have low concentrations of copper in erythrocytes 185. In addition, maternal hypocupremia is often noted in cases of spontaneous abortion or rupture of the fetal and placental membranes 186.
Copper-diet interactions
Zinc
Zinc intakes, well in excess of the amount normally found in the diet, can decrease copper absorption in adults 187. In one case report, an infant who was given 16 to 24 mg/day of zinc developed copper deficiency 188. Very high doses of zinc have been used to treat patients with Wilson’s disease, an inborn error of copper metabolism resulting in copper toxicity 189. This zinc-induced inhibition of copper absorption could be the result of competition for a common, apically oriented transporter or the induction of metallothionein in intestinal cells by zinc. Because this protein has a higher binding affinity for copper than for zinc, copper is retained within enterocytes and its absorption is reduced. This response has been used as a therapy to diminish copper absorption in patients with Wilson’s disease 190. The interaction could also be responsible for reducing copper absorption during consumption of zinc supplements. When zinc-to-copper ratios of 2:1, 5:1, and 15:1 were fed to humans, there were limited effects on copper absorption 191.
Iron
High iron intakes may interfere with copper absorption in infants. Infants fed a formula containing low concentrations of iron absorbed more copper than infants consuming the same formula with a higher iron concentration 192. Such an interaction has been reported to produce reduced copper status in infants 193.
Fructose
Studies in rats demonstrated that diets very high in fructose were associated with increased severity of copper deficiency in rats 194, but a similar effect was not observed in pigs 195, which have cardiovascular systems and gastrointestinal tracts more similar to those of humans. The effects were inconsistent in humans 196 but did not result in copper depletion, and the extremely high levels of fructose fed (20 percent of energy intake) suggest the effect would not be relevant to normal diets.
Gene-induced copper deficiency
There are at least two genetic defects that are expressed as copper-deficiency syndromes (Menkes disease and occipital horn syndrome). Both disorders are due to defects in a copper-transporting P-type ATPase. Infants with Menkes disease are characterized by progressive degeneration of the brain and spinal cord, hypothermia, connective-tissue abnormalities, and failure to thrive. Menkes disease has been recognized as a disorder of copper metabolism for over 20 years. The prognosis for infants with the disorder is poor and death typically occurs before 3 years of age 183. Similar to the blotchy mouse, the developmental abnormalities associated with Menkes disease are thought to be the consequence of low activity of numerous cuproenzymes during embryonic and fetal development. Those cuproenzymes include dopamine-B-monoxygenase, peptidylglycine a-amidating monooxygenase, cytochrome c oxidase, lysyl oxidase, and CuZnSOD 197. The aberrant pattern of the plasma and cerebrospinal fluid of Menkes patients has been attributed to low activity of dopamine-B-monoxygenase 198. Moreover, individuals with Menkes disease are characterized by low activity of ceruloplasmin and peptidylglycine a-amidating monooxygenase 199. Thus, low activity of numerous enzymes that rely on the amidation of peptides for their activity might occur as a secondary effect of low peptidylglycine a-amidating monooxygenase activity.
Groups at Risk of Copper Inadequacy
The following groups are most likely to have inadequate copper status.
People with Celiac disease
In a study of 200 adults and children with celiac disease, of which 69.9% claimed to maintain a gluten-free diet, 15% had copper deficiency (less than 70 mcg/dL in serum in men and girls younger than 12 years and less than 80 mcg/dL in women older than 12 years and/or ceruloplasmin (CP) less than 170 mg/L) as a result of intestinal malabsorption resulting from the intestinal lining alterations associated with celiac disease 200. In its 2009 clinical guidelines for celiac disease, the American College of Gastroenterology notes that people with celiac disease appear to have an increased risk of copper deficiency and that copper levels normalize within a month of adequate copper supplementation while eating a gluten-free diet 201.
People with Menkes disease
Menkes disease is a rare, X-linked, recessive disorder of copper homeostasis caused by ATP7A gene mutations, which encode a copper-transporting ATPase 4. In these individuals, intestinal absorption of dietary copper drops sharply, leading to signs of copper deficiency, including low serum copper and ceruloplasmin (CP) levels 4, 202. The typical manifestations of Menkes disease include failure to thrive, impaired cognitive development, aortic aneurysms, seizures, and unusually kinky hair 15. Most individuals with Menkes disease die by age 3 years if untreated, but subcutaneous injections of copper starting in the first few weeks after birth can reduce mortality risk and improve development 203.
People taking high doses of Zinc supplements
High dietary intakes of zinc can interfere with copper absorption, and excessive use of zinc supplements can lead to copper deficiency. Reductions in red blood cell copper-zinc superoxide dismutase, a marker of copper status, have been reported with even moderately high zinc intakes of approximately 60 mg/day for up to 10 weeks 22. People who regularly consume high doses of zinc from supplements or use excessive amounts of zinc-containing denture creams can develop copper deficiency because zinc can inhibit copper absorption. This is one reason the Food and Nutrition Board established the Tolerable Upper Intake Level (maximum daily intake unlikely to cause adverse health effects) for zinc at 40 mg/day for adults 22.
Copper deficiency signs and symptoms
Copper plays a role as a cofactor for various enzymes in the human body, and is indispensable for myeloid and neurological structure and function 204. Copper deficiency leads to functional disorders of hematopoiesis such as anemia and neutropenia, as well as neurological disorders such as myelopathy 205. Symptoms accompanying the copper deficiency included normocytic, hypochromic anemia, leukopenia, and neutropenia. Osteoporosis was observed in copper-deficient infants and growing children 206.
Acquired copper deficiency has long been recognised as a cause of anemia and, less commonly, other cytopenias 207. It was only in 2001 that an association with myelopathy was reported 171. The neurologic manifestations of copper deficiency may be similar to the myeloneuropathy observed with vitamin B12 deficiency 208. The clinical and radiological picture is usually indistinguishable from subacute combined degeneration due to vitamin B12 (cobalamin) deficiency 209 and the syndrome is thought to represent the human counterpart of swayback or enzootic ataxia, a copper deficiency myelopathy occurring in ruminants 210. Other, less frequently reported and less clearly causally related neurological associations of acquired copper deficiency include isolated peripheral neuropathy 211, motor neuron disease 212, myopathy 213, cerebral demyelination 214, cognitive dysfunction 215 and optic neuropathy 216.
Though copper deficiency is thought to be rare in developed countries, the neurologic symptoms can be profound and are frequently irreversible, making awareness and early diagnosis essential 217.
Copper deficiency test
Copper testing may be ordered when a person has signs and symptoms that may be due to a copper deficiency, such as:
- Abnormally low numbers of neutrophils, a type of white blood cell (neutropenia)
- Osteoporosis
- Anemia
- Less commonly, neurologic symptoms and delayed growth in children
One or more of the copper tests may be ordered periodically when monitoring is recommended.
A hepatic (liver) copper test may be ordered to further investigate copper storage when copper and ceruloplasmin results are abnormal or equivocal.
Copper testing is primarily used to help diagnose Wilson disease, a rare inherited disorder that can lead to excess storage of copper in the liver, brain, and other organs. Less commonly, a copper test may be used to detect copper excess due to another condition, to detect a copper deficiency, or to monitor treatment for one of these conditions.
Rarely, a copper test may be used to help diagnose Menkes kinky hair syndrome (Menkes disease), a rare inherited disorder of copper transport dysfunction.
Copper is an essential mineral but in excess, it can be toxic. In the blood from normal, healthy humans, more than 95% of the copper is incorporated into the enzyme ceruloplasmin and only a small amount is in a “free” or unbound state (loosely bound to albumin).
Typically, a total blood copper test is ordered along with a ceruloplasmin level. If the results from these tests are abnormal or unclear, then they may be followed by a 24-hour urine copper test to measure copper elimination and/or a copper test performed on a liver biopsy to evaluate copper storage in the liver.
Sometimes a free (unbound) blood copper test is also ordered. If Wilson disease is suspected, genetic testing may be performed to detect mutations in the ATP7B gene. However, these tests have limited availability and are usually performed in special reference or research laboratories.
Normal copper reference values
- 0-2 months: 0.40-1.40 mcg/mL
- 3-6 months: 0.40-1.60 mcg/mL
- 7-9 months: 0.40-1.70 mcg/mL
- 10-12 months: 0.80-1.70 mcg/mL
- 13 months-10 years: 0.80-1.80 mcg/mL
- > or =11 years: 0.75-1.45 mcg/mL
When is copper test ordered?
One or more copper tests are ordered along with ceruloplasmin when someone has signs and symptoms that a health practitioner suspects may be due to Wilson disease, excess copper storage, or copper poisoning. These signs and symptoms may include:
- Anemia
- Nausea, abdominal pain
- Jaundice
- Fatigue
- Behavioral changes
- Tremors
- Difficulty walking and/or swallowing
- Dystonia
Copper tests may also be ordered to aid in the diagnosis of:
- Primary biliary cirrhosis
- Primary sclerosing cholangitis
What does abnormal copper test result mean?
Copper test results must be evaluated in context and are usually compared to ceruloplasmin levels. Abnormal copper results are not diagnostic of a specific condition; they indicate the need for further investigation. Interpretation can be complicated by the fact that ceruloplasmin is an acute phase reactant – ceruloplasmin may be elevated whenever inflammation or severe infections are present. Both ceruloplasmin and copper are also increased during pregnancy and with estrogen and oral contraceptive use.
- Serum copper below the normal range is associated with Wilson disease, as well as a variety of other clinical situations (see below). Excess use of denture cream containing zinc can cause hypocupremia.
- Low blood copper concentrations along with increased urine copper levels, low ceruloplasmin levels, and increased hepatic copper are typically seen with Wilson disease.
- Serum concentrations above the normal range are seen in primary biliary cirrhosis and primary sclerosing cholangitis, as well as a variety of other clinical situations (see below).
- Increased blood and urine copper concentrations and normal or increased ceruloplasmin levels may indicate exposure to excess copper or may be associated with conditions that decrease copper excretion, such as chronic liver disease, or that release copper from tissues, such as acute hepatitis.
- Increased hepatic copper may be present with chronic conditions.
- Decreased blood and urine copper concentrations and decreased ceruloplasmin may indicate a copper deficiency.
- A normal hepatic copper test may indicate that copper metabolism is functioning properly or that the distribution of copper in the liver is uneven and the sample is not representative of the person’s condition.
- If a person is being treated for Wilson disease or copper toxicity with drugs that bind copper (chelators), then that person’s 24-hour urine copper levels may be high until body copper stores decrease. Eventually, blood copper and 24-hour urine copper concentrations should normalize.
- If someone is being treated for a condition related to copper deficiency and the person’s ceruloplasmin and total copper concentrations begin to rise, then the condition is likely responding to the treatment.
- Medications such as carbamazepine and phenobarbital can increase blood copper levels. They may also be elevated with rheumatoid arthritis and with some cancers and decreased with a variety of conditions associated with malabsorption, such as cystic fibrosis.
- Total serum copper concentrations are normally low at birth, rise over the next few years, peak, and then decline slightly to a relatively stable level.
Low serum copper, most often due to excess iron or zinc ingestion and infrequently due to dietary copper deficit, results in severe derangement in growth and impaired erythropoiesis. Low serum copper is also observed in hepatolenticular degeneration (Wilson disease) due to a decrease in the synthesis of ceruloplasmin and allelic variances in cellular metal ion transporters. In Wilson disease, the albumin-bound copper may actually be increased, but ceruloplasmin copper is low, resulting in low serum copper. However, during the acute phase of Wilson disease (fulminant hepatic failure), ceruloplasmin and copper may be normal; in this circumstance, hepatic inflammation causes increased release of ceruloplasmin. It is useful to relate the degree of liver inflammation to the ceruloplasmin and copper-see discussion on hypercupremia below. Significant hepatic inflammation with normal ceruloplasmin and copper suggest acute Wilson disease.
Other disorders associated with decreased serum copper concentrations include malnutrition, hypoproteinemia, malabsorption, nephrotic syndrome, Menkes disease, copper toxicity, and megadosing of zinc-containing vitamins (zinc interferes with normal copper absorption from the gastrointestinal tract).
Hypercupremia is found in primary biliary cirrhosis, primary sclerosing cholangitis, hemochromatosis, malignant diseases (including leukemia), thyrotoxicosis, and various infections. Serum copper concentrations are also elevated in patients taking contraceptives or estrogens and during pregnancy.
Since the gastrointestinal (GI) tract effectively excludes excess copper, it is the gastrointestinal tract that is most affected by copper ingestion. Increased serum concentration does not, by itself, indicate copper toxicity.
Table 4. Copper test results interpretation
Test | Wilson Disease | Copper Toxicity | Menkes Disease (Kinky Hair Syndrome) | Copper Deficiency |
---|---|---|---|---|
Copper, blood | Low but may be normal | High | Low | Low |
Copper, serum free | High | High | Low | Low |
Ceruloplasmin | Low but may be normal | High | Low | Low |
Copper, urine | Very high | High | Low | Low |
Copper, liver/hepatic* | Positive but, depending on the site sampled, may be negative | High or normal | Low | Low |
Footnote: *Excess copper in the liver is often unevenly distributed and may not be detected in a sample. Care must be taken, especially with a 24-hour urine sample, not to contaminate the sample with an external source of copper. Talk to the health practitioner and/or the laboratory that will perform the test about necessary precautions. If a urine or blood copper test result is higher than expected, the health practitioner may have the test repeated with a new sample to confirm the findings.
Should everyone’s copper metabolism be evaluated?
General screening for copper concentrations is not recommended or necessary. Many people with conditions not associated with copper, such as people with infections or inflammation, may have temporarily increased concentrations.
Can I choose either a blood (total or free) or urine copper test?
These tests provide complementary information and your healthcare provider will determine which tests are necessary to evaluate your condition.
Copper deficiency treatment
Treatment of copper deficiency consists of parenteral (IV) and oral copper replacement until normal copper levels in blood are achieved. The response to copper replacement is variable and likely depends on the degree and duration of copper depletion. The hematologic abnormalities have been reported to correct within four to six weeks; however, improvements in neurologic symptoms are modest and often difficult to demonstrate objectively. Paresthesias may resolve, but significant gait abnormalities may remain, consistent with clinical observations in reported cases 218. Despite malabsorption being the likely mechanism underlying some copper deficiency after roux-en-Y gastric bypass surgery, oral supplementation has been found to correct copper deficiency, suggesting that super-saturation of intestinal copper transport systems is a reliable way to maintain adequate serum levels 219.
Copper deficiency treatment dose
Typical daily doses were equivalent to 2–4 mg of elemental copper 220. In one case attributed to non-bariatric gastrointestinal surgery, administration of 2 mg of oral copper per day normalized blood parameters and improved neurological symptoms. However, a subsequent symptomatic and biochemical relapse necessitated that the dose be incremented to 4 mg and then 6 mg, which achieved sustained remission 221. Another patient with idiopathic zinc overload was poorly compliant with oral copper supplementation at 2 mg/day. His serum copper levels remained low and the neurological deficit progressed, though serum ceruloplasmin increased to a value at the low end of the normal range and the anaemia resolved. The copper dose was gradually escalated to 8 mg/day but compliance remained poor and he continued to deteriorate neurologically. Subsequently, the patient reported better compliance and his neurological status improved. However, his highest recorded serum copper level remained below the normal range, and it was unclear whether this reflected insufficient dosage, poor compliance or other factors. Copper doses of up to 9 mg per day have been reported 172.
- Eid C, Hémadi M, Ha-Duong NT, El Hage Chahine JM. Iron uptake and transfer from ceruloplasmin to transferrin. Biochim Biophys Acta. 2014 Jun;1840(6):1771-81. doi: 10.1016/j.bbagen.2014.01.011[↩][↩]
- Squitti, R.; Polimanti, R. Copper phenotype in Alzheimer’s disease: Dissecting the pathway. Am. J. Neurodegener. Dis. 2013, 2, 46–56[↩][↩]
- Festa RA, Thiele DJ. Copper: an essential metal in biology. Curr Biol. 2011 Nov 8;21(21):R877-83. doi: 10.1016/j.cub.2011.09.040[↩][↩]
- Collins JF. Copper. In: Ross AC, Caballero B, Cousins RJ, Tucker KL, Ziegler TR, eds. Modern Nutrition in Health and Disease. 11th ed. Baltimore, MD: Lippincott Williams & Wilkins; 2014:206-16.[↩][↩][↩][↩][↩][↩][↩][↩][↩][↩][↩][↩][↩][↩][↩][↩]
- Copper. https://lpi.oregonstate.edu/mic/minerals/copper[↩][↩][↩][↩][↩][↩][↩][↩][↩][↩][↩][↩][↩]
- Copper. https://ods.od.nih.gov/factsheets/Copper-HealthProfessional[↩][↩][↩][↩][↩][↩][↩][↩][↩][↩]
- Owen CAJ. Biochemical Aspects of Copper: Copper Proteins, Ceruloplasmin, and Copper Protein Binding. Park Ridge, NJ: Noyes Publications; 1982.[↩][↩]
- Allen KG, Klevay LM. Copper: an antioxidant nutrient for cardiovascular health. Curr Opin Lipidol. 1994 Feb;5(1):22-8. doi: 10.1097/00041433-199402000-00005[↩][↩]
- Griffith DP, Liff DA, Ziegler TR, Esper GJ, Winton EF. Acquired copper deficiency: a potentially serious and preventable complication following gastric bypass surgery. Obesity (Silver Spring). 2009 Apr;17(4):827-31. doi: 10.1038/oby.2008.614[↩][↩][↩][↩]
- Abnormal Copper Homeostasis: Mechanisms and Roles in Neurodegeneration. Toxics 2014, 2(2), 327-345; doi:10.3390/toxics2020327 https://www.mdpi.com/2305-6304/2/2/327/htm[↩][↩][↩][↩]
- Wilson Disease. https://medlineplus.gov/wilsondisease.html[↩][↩]
- Wilson Disease. https://rarediseases.org/rare-diseases/wilson-disease[↩][↩]
- Linder MC, Hazegh-Azam M. Copper biochemistry and molecular biology. Am J Clin Nutr. 1996 May;63(5):797S-811S. doi: 10.1093/ajcn/63.5.797[↩]
- Scheiber IF, Mercer JF, Dringen R. Metabolism and functions of copper in brain. Prog Neurobiol. 2014 May;116:33-57. doi: 10.1016/j.pneurobio.2014.01.002[↩]
- Hordyjewska A, Popiołek Ł, Kocot J. The many “faces” of copper in medicine and treatment. Biometals. 2014 Aug;27(4):611-21. doi: 10.1007/s10534-014-9736-5[↩][↩]
- Squitti R, Polimanti R. Copper phenotype in Alzheimer’s disease: dissecting the pathway. Am J Neurodegener Dis. 2013 Jun 21;2(2):46-56. https://www.ncbi.nlm.nih.gov/pmc/articles/PMC3703119[↩]
- U.S. Department of Agriculture, Agricultural Research Service. What We Eat in America, 2013-2014. https://www.ars.usda.gov/northeast-area/beltsville-md-bhnrc/beltsville-human-nutrition-research-center/food-surveys-research-group/docs/wweia-data-tables[↩][↩][↩]
- Prohaska JR. Copper. In: Erdman JW, Macdonald IA, Zeisel SH, eds. Present Knowledge in Nutrition. 10th ed. Washington, DC: Wiley-Blackwell; 2012:540-53.[↩][↩][↩][↩][↩][↩][↩][↩][↩][↩][↩][↩][↩][↩]
- Klevay LM. Copper. In: Coates PM, Betz JM, Blackman MR, et al., eds. Encyclopedia of Dietary Supplements. 2nd ed. London and New York: Informa Healthcare; 2010:604-11.[↩][↩][↩]
- Klevay LM. Is the Western diet adequate in copper? J Trace Elem Med Biol. 2011 Dec;25(4):204-12. doi: 10.1016/j.jtemb.2011.08.146[↩][↩]
- Tapiero H, Townsend DM, Tew KD. Trace elements in human physiology and pathology. Copper. Biomed Pharmacother. 2003 Nov;57(9):386-98. doi: 10.1016/s0753-3322(03)00012-x[↩][↩]
- Institute of Medicine (US) Panel on Micronutrients. Dietary Reference Intakes for Vitamin A, Vitamin K, Arsenic, Boron, Chromium, Copper, Iodine, Iron, Manganese, Molybdenum, Nickel, Silicon, Vanadium, and Zinc. Washington (DC): National Academies Press (US); 2001. Available from: https://www.ncbi.nlm.nih.gov/books/NBK222310[↩][↩][↩][↩][↩][↩][↩][↩][↩][↩]
- Chambers A, Krewski D, Birkett N, Plunkett L, Hertzberg R, Danzeisen R, Aggett PJ, Starr TB, Baker S, Dourson M, Jones P, Keen CL, Meek B, Schoeny R, Slob W. An exposure-response curve for copper excess and deficiency. J Toxicol Environ Health B Crit Rev. 2010 Oct;13(7-8):546-78. doi: 10.1080/10937404.2010.538657[↩][↩]
- Nishiwaki S, Iwashita M, Goto N, Hayashi M, Takada J, Asano T, Tagami A, Hatakeyama H, Hayashi T, Maeda T, Saito K. Predominant copper deficiency during prolonged enteral nutrition through a jejunostomy tube compared to that through a gastrostomy tube. Clin Nutr. 2011 Oct;30(5):585-9. doi: 10.1016/j.clnu.2011.04.008[↩][↩]
- Kelley DS, Daudu PA, Taylor PC, Mackey BE, Turnlund JR. Effects of low-copper diets on human immune response. Am J Clin Nutr. 1995 Aug;62(2):412-6. doi: 10.1093/ajcn/62.2.412[↩][↩][↩]
- Institute of Medicine (US) Standing Committee on the Scientific Evaluation of Dietary Reference Intakes and its Panel on Folate, Other B Vitamins, and Choline. Dietary Reference Intakes for Thiamin, Riboflavin, Niacin, Vitamin B6, Folate, Vitamin B12, Pantothenic Acid, Biotin, and Choline. Washington (DC): National Academies Press (US); 1998. Available from: https://www.ncbi.nlm.nih.gov/books/NBK114310[↩][↩][↩][↩][↩]
- Rae TD, Schmidt PJ, Pufahl RA, Culotta VC, O’Halloran TV. Undetectable intracellular free copper: the requirement of a copper chaperone for superoxide dismutase. Science. 1999 Apr 30;284(5415):805-8. doi: 10.1126/science.284.5415.805[↩]
- Hellman, N.E.; Gitlin, J.D. Ceruloplasmin metabolism and function. Annu. Rev. Nutr. 2002, 22, 439–458.[↩]
- Vashchenko, G.; MacGillivray, R.T. Multi-copper oxidases and human iron metabolism. Nutrients 2013, 5, 2289–2313.[↩]
- Eid, C.; Hémadi, M.; Ha-Duong, N.T.; El Hage Chahine, J.M. Iron uptake and transfer from ceruloplasmin to transferrin. Biochim. Biophys. Acta 2014, 1840, 1771–1781.[↩]
- Kono, S. Aceruloplasminemia: An update. Int. Rev. Neurobiol. 2013, 110, 125–151.[↩]
- Hare, D.; Ayton, S.; Bush, A.; Lei, P. A delicate balance: Iron metabolism and diseases of the brain. Front. Aging Neurosci. 2013, 5, 34.[↩]
- Prohaska JR. Impact of copper limitation on expression and function of multicopper oxidases (ferroxidases). Adv Nutr. 2011 Mar;2(2):89-95. doi: 10.3945/an.110.000208[↩][↩]
- Collins JF. Copper nutrition and biochemistry and human (patho)physiology. Adv Food Nutr Res. 2021;96:311-364. doi: 10.1016/bs.afnr.2021.01.005[↩]
- Collins JF. Copper. In: Ross AC, Caballero B, Cousins RJ, Tucker KL, Ziegler TR, eds. Modern Nutrition in Health and Disease. 11th ed. Philadelphia: Wolter Kluwer; Lippincott Williams & Wilkins; 2014:206-216.[↩]
- Collins JF. Copper. In: Marriott BP, Birt DF, Stalling VA, Yates AA, eds. Present Knowledge in Nutrition. 11th ed: Academic Press; 2020:409-427.[↩]
- Uauy R, Olivares M, Gonzalez M. Essentiality of copper in humans. Am J Clin Nutr. 1998 May;67(5 Suppl):952S-959S. doi: 10.1093/ajcn/67.5.952S[↩][↩]
- Turnlund JR. Copper. In: Shils ME, Shike M, Ross A, Caballero B, Cousins RA, eds. Modern Nutrition in Health and Disease. 10th ed. Baltimore: Lipincott Williams & Wilkins; 2006:289-299.[↩][↩][↩][↩][↩][↩]
- Vashchenko G, MacGillivray RT. Multi-copper oxidases and human iron metabolism. Nutrients. 2013 Jun 27;5(7):2289-313. doi: 10.3390/nu5072289[↩]
- Vasilyev VB. Looking for a partner: ceruloplasmin in protein-protein interactions. Biometals. 2019 Apr;32(2):195-210. doi: 10.1007/s10534-019-00189-1[↩]
- Meyer LA, Durley AP, Prohaska JR, Harris ZL. Copper transport and metabolism are normal in aceruloplasminemic mice. J Biol Chem. 2001 Sep 28;276(39):36857-61. doi: 10.1074/jbc.M105361200[↩]
- Harris ZL, Durley AP, Man TK, Gitlin JD. Targeted gene disruption reveals an essential role for ceruloplasmin in cellular iron efflux. Proc Natl Acad Sci U S A. 1999 Sep 14;96(19):10812-7. doi: 10.1073/pnas.96.19.10812[↩]
- Kono S. Aceruloplasminemia. Curr Drug Targets. 2012 Aug;13(9):1190-9. doi: 10.2174/138945012802002320[↩]
- Thackeray EW, Sanderson SO, Fox JC, Kumar N. Hepatic iron overload or cirrhosis may occur in acquired copper deficiency and is likely mediated by hypoceruloplasminemia. J Clin Gastroenterol. 2011 Feb;45(2):153-8. doi: 10.1097/MCG.0b013e3181dc25f7[↩]
- Harris E. Copper. In: O’Dell B, Sunde R, eds. Handbook of Nutritionally Essential Minerals. New York: Marcel Dekker, Inc.; 1997:231-273.[↩]
- Johnson MA, Fischer JG, Kays SE. Is copper an antioxidant nutrient? Crit Rev Food Sci Nutr. 1992;32(1):1-31. doi: 10.1080/10408399209527583[↩][↩]
- van den Berghe PV, Klomp LW. Posttranslational regulation of copper transporters. J Biol Inorg Chem. 2010 Jan;15(1):37-46. doi: 10.1007/s00775-009-0592-7[↩]
- Armendariz AD, Gonzalez M, Loguinov AV, Vulpe CD. Gene expression profiling in chronic copper overload reveals upregulation of Prnp and App. Physiol Genomics. 2004 Dec 15;20(1):45-54. doi: 10.1152/physiolgenomics.00196.2003[↩]
- Armendáriz AD, Olivares F, Pulgar R, Loguinov A, Cambiazo V, Vulpe CD, González M. Gene expression profiling in wild-type and metallothionein mutant fibroblast cell lines. Biol Res. 2006;39(1):125-42. doi: 10.4067/s0716-97602006000100015[↩]
- González M, Reyes-Jara A, Suazo M, Jo WJ, Vulpe C. Expression of copper-related genes in response to copper load. Am J Clin Nutr. 2008 Sep;88(3):830S-4S. doi: 10.1093/ajcn/88.3.830S[↩]
- Mattie MD, McElwee MK, Freedman JH. Mechanism of copper-activated transcription: activation of AP-1, and the JNK/SAPK and p38 signal transduction pathways. J Mol Biol. 2008 Nov 28;383(5):1008-18. doi: 10.1016/j.jmb.2008.08.080[↩]
- Gombart AF, Pierre A, Maggini S. A Review of Micronutrients and the Immune System-Working in Harmony to Reduce the Risk of Infection. Nutrients. 2020 Jan 16;12(1):236. doi: 10.3390/nu12010236[↩]
- Failla ML, Hopkins RG. Is low copper status immunosuppressive? Nutr Rev. 1998 Jan;56(1 Pt 2):S59-64. doi: 10.1111/j.1753-4887.1998.tb01646.x[↩]
- Percival SS. Copper and immunity. Am J Clin Nutr. 1998 May;67(5 Suppl):1064S-1068S. doi: 10.1093/ajcn/67.5.1064S[↩]
- Heresi G, Castillo-Duran C, Munoz C, Arevalo M, Schlesinger L. Phagocytosis and immunoglobulin levels in hypocupremic children. Nutr Res. 1985;5:1327-1334.[↩]
- Hodgkinson V, Petris MJ. Copper homeostasis at the host-pathogen interface. J Biol Chem. 2012 Apr 20;287(17):13549-55. doi: 10.1074/jbc.R111.316406[↩]
- Govind V, Bharadwaj S, Sai Ganesh MR, Vishnu J, Shankar KV, Shankar B, Rajesh R. Antiviral properties of copper and its alloys to inactivate covid-19 virus: a review. Biometals. 2021 Dec;34(6):1217-1235. doi: 10.1007/s10534-021-00339-4[↩]
- Ford ES. Serum copper concentration and coronary heart disease among US adults. Am J Epidemiol. 2000 Jun 15;151(12):1182-8. doi: 10.1093/oxfordjournals.aje.a010168[↩]
- Cabral M, Kuxhaus O, Eichelmann F, Kopp JF, Alker W, Hackler J, Kipp AP, Schwerdtle T, Haase H, Schomburg L, Schulze MB. Trace element profile and incidence of type 2 diabetes, cardiovascular disease and colorectal cancer: results from the EPIC-Potsdam cohort study. Eur J Nutr. 2021 Sep;60(6):3267-3278. doi: 10.1007/s00394-021-02494-3[↩]
- Malek F, Jiresova E, Dohnalova A, Koprivova H, Spacek R. Serum copper as a marker of inflammation in prediction of short term outcome in high risk patients with chronic heart failure. Int J Cardiol. 2006 Nov 10;113(2):e51-3. doi: 10.1016/j.ijcard.2006.05.022[↩]
- Kunutsor SK, Voutilainen A, Kurl S, Laukkanen JA. Serum copper-to-zinc ratio is associated with heart failure and improves risk prediction in middle-aged and older Caucasian men: A prospective study. Nutr Metab Cardiovasc Dis. 2022 Aug;32(8):1924-1935. doi: 10.1016/j.numecd.2022.05.005[↩]
- Leone N, Courbon D, Ducimetiere P, Zureik M. Zinc, copper, and magnesium and risks for all-cause, cancer, and cardiovascular mortality. Epidemiology. 2006 May;17(3):308-14. doi: 10.1097/01.ede.0000209454.41466.b7[↩]
- Kosar F, Sahin I, Acikgöz N, Aksoy Y, Kucukbay Z, Cehreli S. Significance of serum trace element status in patients with rheumatic heart disease: a prospective study. Biol Trace Elem Res. 2005 Oct;107(1):1-10. doi: 10.1385/BTER:107:1:001[↩]
- Liu N, Lo LS, Askary SH, Jones L, Kidane TZ, Trang T, Nguyen M, Goforth J, Chu YH, Vivas E, Tsai M, Westbrook T, Linder MC. Transcuprein is a macroglobulin regulated by copper and iron availability. J Nutr Biochem. 2007 Sep;18(9):597-608. doi: 10.1016/j.jnutbio.2006.11.005[↩]
- Moriya M, Ho YH, Grana A, Nguyen L, Alvarez A, Jamil R, Ackland ML, Michalczyk A, Hamer P, Ramos D, Kim S, Mercer JF, Linder MC. Copper is taken up efficiently from albumin and alpha2-macroglobulin by cultured human cells by more than one mechanism. Am J Physiol Cell Physiol. 2008 Sep;295(3):C708-21. doi: 10.1152/ajpcell.00029.2008[↩]
- Bertinato J, Zouzoulas A. Considerations in the development of biomarkers of copper status. J AOAC Int. 2009 Sep-Oct;92(5):1541-50.[↩]
- Klevay LM. Cardiovascular disease from copper deficiency–a history. J Nutr. 2000 Feb;130(2S Suppl):489S-492S. doi: 10.1093/jn/130.2.489S[↩]
- Mielcarz G, Howard AN, Mielcarz B, Williams NR, Rajput-Williams J, Nigdigar SV, Stone DL. Leucocyte copper, a marker of copper body status is low in coronary artery disease. J Trace Elem Med Biol. 2001;15(1):31-5. doi: 10.1016/S0946-672X(01)80023-7[↩]
- Kinsman GD, Howard AN, Stone DL, Mullins PA. Studies in copper status and atherosclerosis. Biochem Soc Trans. 1990 Dec;18(6):1186-8. doi: 10.1042/bst0181186[↩]
- Wang XL, Adachi T, Sim AS, Wilcken DE. Plasma extracellular superoxide dismutase levels in an Australian population with coronary artery disease. Arterioscler Thromb Vasc Biol. 1998 Dec;18(12):1915-21. doi: 10.1161/01.atv.18.12.1915[↩]
- Eshak ES, Iso H, Yamagishi K, Maruyama K, Umesawa M, Tamakoshi A. Associations between copper and zinc intakes from diet and mortality from cardiovascular disease in a large population-based prospective cohort study. J Nutr Biochem. 2018 Jun;56:126-132. doi: 10.1016/j.jnutbio.2018.02.008[↩][↩]
- Mansoor MA, Bergmark C, Haswell SJ, Savage IF, Evans PH, Berge RK, Svardal AM, Kristensen O. Correlation between plasma total homocysteine and copper in patients with peripheral vascular disease. Clin Chem. 2000 Mar;46(3):385-91. Erratum in: Clin Chem 2001 Aug;47(8):1505.[↩]
- Celik C, Bastu E, Abali R, Alpsoy S, Guzel EC, Aydemir B, Yeh J. The relationship between copper, homocysteine and early vascular disease in lean women with polycystic ovary syndrome. Gynecol Endocrinol. 2013 May;29(5):488-91. doi: 10.3109/09513590.2013.774361[↩]
- Gupta M, Meehan-Atrash J, Strongin RM. Identifying a role for the interaction of homocysteine and copper in promoting cardiovascular-related damage. Amino Acids. 2021 May;53(5):739-744. doi: 10.1007/s00726-021-02979-9[↩]
- Barter PJ, Rye KA. Homocysteine and cardiovascular disease: is HDL the link? Circ Res. 2006 Sep 15;99(6):565-6. doi: 10.1161/01.RES.0000243583.39694.1f[↩]
- Emsley AM, Jeremy JY, Gomes GN, Angelini GD, Plane F. Investigation of the inhibitory effects of homocysteine and copper on nitric oxide-mediated relaxation of rat isolated aorta. Br J Pharmacol. 1999 Feb;126(4):1034-40. doi: 10.1038/sj.bjp.0702374[↩][↩]
- Shukla N, Angelini GD, Jeremy JY. Interactive effects of homocysteine and copper on angiogenesis in porcine isolated saphenous vein. Ann Thorac Surg. 2007 Jul;84(1):43-9. doi: 10.1016/j.athoracsur.2007.03.087[↩]
- Uthus EO, Reeves PG, Saari JT. Copper deficiency decreases plasma homocysteine in rats. J Nutr. 2007 Jun;137(6):1370-4. doi: 10.1093/jn/137.6.1370[↩]
- Wei H, Zhang WJ, McMillen TS, Leboeuf RC, Frei B. Copper chelation by tetrathiomolybdate inhibits vascular inflammation and atherosclerotic lesion development in apolipoprotein E-deficient mice. Atherosclerosis. 2012 Aug;223(2):306-13. doi: 10.1016/j.atherosclerosis.2012.06.013[↩]
- Tsikas D. Homocysteine and copper ions: is their interaction responsible for cardiovascular-related damage? Amino Acids. 2021 Aug;53(8):1297-1298. doi: 10.1007/s00726-021-03010-x[↩]
- DiSilvestro RA, Joseph EL, Zhang W, Raimo AE, Kim YM. A randomized trial of copper supplementation effects on blood copper enzyme activities and parameters related to cardiovascular health. Metabolism. 2012 Sep;61(9):1242-6. doi: 10.1016/j.metabol.2012.02.002[↩]
- Bügel S, Harper A, Rock E, O’Connor JM, Bonham MP, Strain JJ. Effect of copper supplementation on indices of copper status and certain CVD risk markers in young healthy women. Br J Nutr. 2005 Aug;94(2):231-6. doi: 10.1079/bjn20051470[↩]
- Klevay LM. Alzheimer’s disease as copper deficiency. Med Hypotheses. 2008;70(4):802-7. doi: 10.1016/j.mehy.2007.04.051[↩]
- Siotto M, Simonelli I, Pasqualetti P, Mariani S, Caprara D, Bucossi S, Ventriglia M, Molinario R, Antenucci M, Rongioletti M, Rossini PM, Squitti R. Association Between Serum Ceruloplasmin Specific Activity and Risk of Alzheimer’s Disease. J Alzheimers Dis. 2016;50(4):1181-9. doi: 10.3233/JAD-150611[↩]
- Lanza V, Milardi D, Di Natale G, Pappalardo G. Repurposing of Copper(II)-chelating Drugs for the Treatment of Neurodegenerative Diseases. Curr Med Chem. 2018 Feb 12;25(4):525-539. doi: 10.2174/0929867324666170518094404[↩]
- Rembach A, Doecke JD, Roberts BR, Watt AD, Faux NG, Volitakis I, Pertile KK, Rumble RL, Trounson BO, Fowler CJ, Wilson W, Ellis KA, Martins RN, Rowe CC, Villemagne VL, Ames D, Masters CL, AIBL research group, Bush AI. Longitudinal analysis of serum copper and ceruloplasmin in Alzheimer’s disease. J Alzheimers Dis. 2013;34(1):171-82. doi: 10.3233/JAD-121474[↩][↩][↩]
- Morris MC, Evans DA, Tangney CC, Bienias JL, Schneider JA, Wilson RS, Scherr PA. Dietary copper and high saturated and trans fat intakes associated with cognitive decline. Arch Neurol. 2006 Aug;63(8):1085-8. doi: 10.1001/archneur.63.8.1085[↩][↩][↩]
- Bucossi S, Ventriglia M, Panetta V, Salustri C, Pasqualetti P, Mariani S, Siotto M, Rossini PM, Squitti R. Copper in Alzheimer’s disease: a meta-analysis of serum,plasma, and cerebrospinal fluid studies. J Alzheimers Dis. 2011;24(1):175-85. doi: 10.3233/JAD-2010-101473. Corrected and republished in: J Alzheimers Dis. 2012;30(4):981-4[↩]
- Squitti R, Simonelli I, Ventriglia M, Siotto M, Pasqualetti P, Rembach A, Doecke J, Bush AI. Meta-analysis of serum non-ceruloplasmin copper in Alzheimer’s disease. J Alzheimers Dis. 2014;38(4):809-22. doi: 10.3233/JAD-131247[↩]
- Squitti R, Ventriglia M, Simonelli I, Bonvicini C, Costa A, Perini G, Binetti G, Benussi L, Ghidoni R, Koch G, Borroni B, Albanese A, Sensi SL, Rongioletti M. Copper Imbalance in Alzheimer’s Disease: Meta-Analysis of Serum, Plasma, and Brain Specimens, and Replication Study Evaluating ATP7B Gene Variants. Biomolecules. 2021 Jun 29;11(7):960. doi: 10.3390/biom11070960[↩]
- Li DD, Zhang W, Wang ZY, Zhao P. Serum Copper, Zinc, and Iron Levels in Patients with Alzheimer’s Disease: A Meta-Analysis of Case-Control Studies. Front Aging Neurosci. 2017 Sep 15;9:300. doi: 10.3389/fnagi.2017.00300[↩]
- Squitti R, Polimanti R. Copper hypothesis in the missing hereditability of sporadic Alzheimer’s disease: ATP7B gene as potential harbor of rare variants. J Alzheimers Dis. 2012;29(3):493-501. doi: 10.3233/JAD-2011-111991[↩]
- Kessler H, Bayer TA, Bach D, Schneider-Axmann T, Supprian T, Herrmann W, Haber M, Multhaup G, Falkai P, Pajonk FG. Intake of copper has no effect on cognition in patients with mild Alzheimer’s disease: a pilot phase 2 clinical trial. J Neural Transm (Vienna). 2008 Aug;115(8):1181-7. doi: 10.1007/s00702-008-0080-1[↩][↩]
- Barnard ND, Bush AI, Ceccarelli A, Cooper J, de Jager CA, Erickson KI, Fraser G, Kesler S, Levin SM, Lucey B, Morris MC, Squitti R. Dietary and lifestyle guidelines for the prevention of Alzheimer’s disease. Neurobiol Aging. 2014 Sep;35 Suppl 2:S74-8. doi: 10.1016/j.neurobiolaging.2014.03.033[↩]
- Sparks DL, Schreurs BG. Trace amounts of copper in water induce beta-amyloid plaques and learning deficits in a rabbit model of Alzheimer’s disease. Proc Natl Acad Sci U S A. 2003 Sep 16;100(19):11065-9. doi: 10.1073/pnas.1832769100. Erratum in: Proc Natl Acad Sci U S A. 2003 Sep 30;100(20):11816.[↩][↩]
- Kitazawa M, Cheng D, Laferla FM. Chronic copper exposure exacerbates both amyloid and tau pathology and selectively dysregulates cdk5 in a mouse model of AD. J Neurochem. 2009 Mar;108(6):1550-60. doi: 10.1111/j.1471-4159.2009.05901.x[↩]
- Kessler H, Pajonk FG, Bach D, Schneider-Axmann T, Falkai P, Herrmann W, Multhaup G, Wiltfang J, Schäfer S, Wirths O, Bayer TA. Effect of copper intake on CSF parameters in patients with mild Alzheimer’s disease: a pilot phase 2 clinical trial. J Neural Transm (Vienna). 2008 Dec;115(12):1651-9. doi: 10.1007/s00702-008-0136-2[↩]
- Brewer GJ. Copper excess, zinc deficiency, and cognition loss in Alzheimer’s disease. Biofactors. 2012 Mar-Apr;38(2):107-13. doi: 10.1002/biof.1005[↩]
- Skjørringe T, Møller LB, Moos T. Impairment of interrelated iron- and copper homeostatic mechanisms in brain contributes to the pathogenesis of neurodegenerative disorders. Front Pharmacol. 2012 Sep 25;3:169. doi: 10.3389/fphar.2012.00169[↩]
- Bisaglia M, Bubacco L. Copper Ions and Parkinson’s Disease: Why Is Homeostasis So Relevant? Biomolecules. 2020 Jan 29;10(2):195. doi: 10.3390/biom10020195[↩]
- Akatsu H, Hori A, Yamamoto T, Yoshida M, Mimuro M, Hashizume Y, Tooyama I, Yezdimer EM. Transition metal abnormalities in progressive dementias. Biometals. 2012 Apr;25(2):337-50. doi: 10.1007/s10534-011-9504-8[↩]
- Davies KM, Bohic S, Carmona A, Ortega R, Cottam V, Hare DJ, Finberg JP, Reyes S, Halliday GM, Mercer JF, Double KL. Copper pathology in vulnerable brain regions in Parkinson’s disease. Neurobiol Aging. 2014 Apr;35(4):858-66. doi: 10.1016/j.neurobiolaging.2013.09.034[↩]
- Kim MJ, Oh SB, Kim J, Kim K, Ryu HS, Kim MS, Ayton S, Bush AI, Lee JY, Chung SJ. Association of metals with the risk and clinical characteristics of Parkinson’s disease. Parkinsonism Relat Disord. 2018 Oct;55:117-121. doi: 10.1016/j.parkreldis.2018.05.022[↩]
- Ilyechova EY, Miliukhina IV, Orlov IA, Muruzheva ZM, Puchkova LV, Karpenko MN. A low blood copper concentration is a co-morbidity burden factor in Parkinson’s disease development. Neurosci Res. 2018 Oct;135:54-62. doi: 10.1016/j.neures.2017.11.011[↩]
- Younes-Mhenni S, Aissi M, Mokni N, Boughammoura-Bouatay A, Chebel S, Frih-Ayed M, Kerkeni A, Bost M, Chazot G, Sfar MT, Sfar MH. Serum copper, zinc and selenium levels in Tunisian patients with Parkinson’s disease. Tunis Med. 2013 Jun;91(6):402-5.[↩]
- Miyake Y, Tanaka K, Fukushima W, Sasaki S, Kiyohara C, Tsuboi Y, Yamada T, Oeda T, Miki T, Kawamura N, Sakae N, Fukuyama H, Hirota Y, Nagai M; Fukuoka Kinki Parkinson’s Disease Study Group. Dietary intake of metals and risk of Parkinson’s disease: a case-control study in Japan. J Neurol Sci. 2011 Jul 15;306(1-2):98-102. doi: 10.1016/j.jns.2011.03.035[↩]
- Powers KM, Smith-Weller T, Franklin GM, Longstreth WT Jr, Swanson PD, Checkoway H. Parkinson’s disease risks associated with dietary iron, manganese, and other nutrient intakes. Neurology. 2003 Jun 10;60(11):1761-6. doi: 10.1212/01.wnl.0000068021.13945.7f[↩]
- Looker AC, Melton LJ 3rd, Harris TB, Borrud LG, Shepherd JA. Prevalence and trends in low femur bone density among older US adults: NHANES 2005-2006 compared with NHANES III. J Bone Miner Res. 2010 Jan;25(1):64-71. doi: 10.1359/jbmr.090706[↩]
- Tiidus PM, Lowe DA, Brown M. Estrogen replacement and skeletal muscle: mechanisms and population health. J Appl Physiol (1985). 2013 Sep 1;115(5):569-78. doi: 10.1152/japplphysiol.00629.2013[↩]
- Cauley JA. Public health impact of osteoporosis. J Gerontol A Biol Sci Med Sci. 2013 Oct;68(10):1243-51. doi: 10.1093/gerona/glt093[↩]
- Kanumakala S, Boneh A, Zacharin M. Pamidronate treatment improves bone mineral density in children with Menkes disease. J Inherit Metab Dis. 2002 Sep;25(5):391-8. doi: 10.1023/a:1020103901969[↩]
- Marquardt ML, Done SL, Sandrock M, Berdon WE, Feldman KW. Copper deficiency presenting as metabolic bone disease in extremely low birth weight, short-gut infants. Pediatrics. 2012 Sep;130(3):e695-8. doi: 10.1542/peds.2011-1295[↩]
- Baker A, Harvey L, Majask-Newman G, Fairweather-Tait S, Flynn A, Cashman K. Effect of dietary copper intakes on biochemical markers of bone metabolism in healthy adult males. Eur J Clin Nutr. 1999 May;53(5):408-12. doi: 10.1038/sj.ejcn.1600763[↩]
- Baker A, Turley E, Bonham MP, O’Connor JM, Strain JJ, Flynn A, Cashman KD. No effect of copper supplementation on biochemical markers of bone metabolism in healthy adults. Br J Nutr. 1999 Oct;82(4):283-90.[↩]
- Cashman KD, Baker A, Ginty F, Flynn A, Strain JJ, Bonham MP, O’Connor JM, Bügel S, Sandström B. No effect of copper supplementation on biochemical markers of bone metabolism in healthy young adult females despite apparently improved copper status. Eur J Clin Nutr. 2001 Jul;55(7):525-31. doi: 10.1038/sj.ejcn.1601177[↩]
- Eaton-Evans J, Mellwrath EM, Jackson WE, McCartney H, Strain JJ. Copper supplementation and the maintenance of bone mineral density in middle-aged women. J Trace Elem Exp Med. 1996;9:87-94.[↩]
- Strause L, Saltman P, Smith KT, Bracker M, Andon MB. Spinal bone loss in postmenopausal women supplemented with calcium and trace minerals. J Nutr. 1994 Jul;124(7):1060-4. doi: 10.1093/jn/124.7.1060[↩][↩]
- Nielsen FH, Lukaski HC, Johnson LK, Roughead ZK. Reported zinc, but not copper, intakes influence whole-body bone density, mineral content and T score responses to zinc and copper supplementation in healthy postmenopausal women. Br J Nutr. 2011 Dec;106(12):1872-9. doi: 10.1017/S0007114511002352[↩]
- Sidiropoulou-Chatzigiannis S, Kourtidou M, Tsalikis L. The effect of osteoporosis on periodontal status, alveolar bone and orthodontic tooth movement. A literature review. J Int Acad Periodontol. 2007 Jul;9(3):77-84.[↩]
- Darcey J, Horner K, Walsh T, Southern H, Marjanovic EJ, Devlin H. Tooth loss and osteoporosis: to assess the association between osteoporosis status and tooth number. Br Dent J. 2013 Feb;214(4):E10. doi: 10.1038/sj.bdj.2013.165[↩]
- Sierpinska T, Konstantynowicz J, Orywal K, Golebiewska M, Szmitkowski M. Copper deficit as a potential pathogenic factor of reduced bone mineral density and severe tooth wear. Osteoporos Int. 2014 Feb;25(2):447-54. doi: 10.1007/s00198-013-2410-x[↩]
- Heffern MC, Park HM, Au-Yeung HY, Van de Bittner GC, Ackerman CM, Stahl A, Chang CJ. In vivo bioluminescence imaging reveals copper deficiency in a murine model of nonalcoholic fatty liver disease. Proc Natl Acad Sci U S A. 2016 Dec 13;113(50):14219-14224. doi: 10.1073/pnas.1613628113[↩]
- Aigner E, Theurl I, Haufe H, Seifert M, Hohla F, Scharinger L, Stickel F, Mourlane F, Weiss G, Datz C. Copper availability contributes to iron perturbations in human nonalcoholic fatty liver disease. Gastroenterology. 2008 Aug;135(2):680-8. doi: 10.1053/j.gastro.2008.04.007[↩]
- Lan Y, Wu S, Wang Y, Chen S, Liao W, Zhang X, Pan L, Jiang X, Zhang Y, Wang L. Association between blood copper and nonalcoholic fatty liver disease according to sex. Clin Nutr. 2021 Apr;40(4):2045-2052. doi: 10.1016/j.clnu.2020.09.026[↩]
- Aigner E, Strasser M, Haufe H, Sonnweber T, Hohla F, Stadlmayr A, Solioz M, Tilg H, Patsch W, Weiss G, Stickel F, Datz C. A role for low hepatic copper concentrations in nonalcoholic Fatty liver disease. Am J Gastroenterol. 2010 Sep;105(9):1978-85. doi: 10.1038/ajg.2010.170[↩][↩]
- Stättermayer AF, Traussnigg S, Aigner E, Kienbacher C, Huber-Schönauer U, Steindl-Munda P, Stadlmayr A, Wrba F, Trauner M, Datz C, Ferenci P. Low hepatic copper content and PNPLA3 polymorphism in non-alcoholic fatty liver disease in patients without metabolic syndrome. J Trace Elem Med Biol. 2017 Jan;39:100-107. doi: 10.1016/j.jtemb.2016.08.006[↩][↩]
- US Department of Agriculture, Agricultural Research Service. 2022. Nutrient Intakes from Food and Beverages: Mean Amounts Consumed per Individual, by Gender and Age, What We Eat in America, NHANES 2017-March 2020 Prepandemic. https://www.ars.usda.gov/ARSUserFiles/80400530/pdf/1720/Table_25_SNK_GEN_1720.pdf[↩]
- Copper. https://ods.od.nih.gov/factsheets/Copper-Consumer[↩]
- Food Labeling: Revision of the Nutrition and Supplement Facts Labels. https://www.federalregister.gov/documents/2016/05/27/2016-11867/food-labeling-revision-of-the-nutrition-and-supplement-facts-labels[↩]
- Rosado JL. Zinc and copper: proposed fortification levels and recommended zinc compounds. J Nutr. 2003 Sep;133(9):2985S-9S. doi: 10.1093/jn/133.9.2985S[↩]
- Pratt WB, Omdahl JL, Sorenson JR. Lack of effects of copper gluconate supplementation. Am J Clin Nutr. 1985 Oct;42(4):681-2. doi: 10.1093/ajcn/42.4.681[↩]
- Blumberg JB, Frei B, Fulgoni VL, Weaver CM, Zeisel SH. Contribution of Dietary Supplements to Nutritional Adequacy in Various Adult Age Groups. Nutrients. 2017 Dec 6;9(12):1325. doi: 10.3390/nu9121325[↩][↩]
- Cochrane Review 17 October 2007 – Effectiveness of Copper-containing intra-uterine devices (coil) – http://www.cochrane.org/CD005347/FERTILREG_effectiveness-of-copper-containing-intra-uterine-devices-coil[↩]
- Cochrane Review 16 June 2010 – Copper IUDs versus long-acting hormone injections and implants for contraception – http://www.cochrane.org/CD007043/FERTILREG_copper-iuds-versus-long-acting-hormone-injections-and-implants-for-contraception[↩]
- Cochrane Review 14 March 2012 – Ways to increase use of non-hormonal long-acting birth control – http://www.cochrane.org/CD008896/FERTILREG_ways-to-increase-use-of-non-hormonal-long-acting-birth-control[↩]
- Trocello JM, Broussolle E, Girardot-Tinant N, Pelosse M, Lachaux A, Lloyd C, Woimant F. Wilson’s disease, 100 years later…. Rev Neurol (Paris). 2013 Dec;169(12):936-43. doi: 10.1016/j.neurol.2013.05.002[↩]
- National Research Council Committee on Copper in Drinking Water. Copper in Drinking Water. Washington, DC: National Academies Press; 2000.[↩][↩]
- Environmental Protection Agency. Electronic Code of Federal Regulations, Title 40, Part 141. https://www.ecfr.gov/current/title-40/chapter-I/subchapter-D/part-141/subpart-I[↩]
- Fitzgerald DJ. Safety guidelines for copper in water. Am J Clin Nutr. 1998 May;67(5 Suppl):1098S-1102S. doi: 10.1093/ajcn/67.5.1098S[↩]
- Puig, S.; Thiele, D. Molecular mechanism of copper uptake and distribution. Curr. Opin. Chem. Biol. 2002, 6, 171–180.[↩]
- Brewer, G.J. Copper in medicine. Curr. Opin. Chem. Biol. 2003, 7, 207–212.[↩]
- Chambers, A.; Krewski, D.; Birkett, N.; Plunkett, L.; Hertzberg, R.; Danzeisen, R.; Aggett, P.J.; Starr, T.B.; Baker, S.; Dourson, M.; et al. An exposure-response curve for copper excess and deficiency. J. Toxicol. Environ. Health B 2010, 13, 546–578.[↩]
- Araya, M.; Olivares, M.; Pizarro, F.; Méndez, M.A.; González, M.; Uauy, R. Supplementing copper at the upper level of the adult dietary recommended intake induces detectable but transient changes in healthy adults. J. Nutr. 2005, 135, 2367–2371.[↩]
- Schramel, P.; Müller-Höcker, J.; Meyer, U.; Weiss, M.; Eife, R. Nutritional copper intoxication in three German infants with severe liver cell damage (features of Indian childhood cirrhosis). J. Trace Elem. Electrolytes Health Dis. 1988, 2, 85–89.[↩][↩]
- Baker, A.; Gormally, S.; Saxena, R.; Baldwin, D.; Drumm, B.; Bonham, J.; Portmann, B.; Mowat, A.P. Copper-associated liver disease in childhood. J. Hepatol. 1995, 23, 538–543.[↩]
- Ozcelik, D.; Uzun, H. Copper intoxication, antioxidant defenses and oxidative damage in rat brain. Biol. Trace Elem. Res. 2009, 127, 45–52.[↩]
- Kozak SF, Inderlied CB, Hsu HY, Heller KB, Sadun AA. The role of copper on ethambutol’s antimicrobial action and implications for ethambutol-induced optic neuropathy. Diagn Microbiol Infect Dis. 1998 Feb;30(2):83-7. doi: 10.1016/s0732-8893(97)00217-4[↩]
- Gabreyes AA, Abbasi HN, Forbes KP, McQuaker G, Duncan A, Morrison I. Hypocupremia associated cytopenia and myelopathy: a national retrospective review. Eur J Haematol. 2013 Jan;90(1):1-9. doi: 10.1111/ejh.12020[↩]
- Halfdanarson TR, Kumar N, Li CY, Phyliky RL, Hogan WJ. Hematological manifestations of copper deficiency: a retrospective review. Eur J Haematol. 2008 Jun;80(6):523-31. doi: 10.1111/j.1600-0609.2008.01050.x[↩][↩]
- Prodan CI, Holland NR, Wisdom PJ, Burstein SA, Bottomley SS. CNS demyelination associated with copper deficiency and hyperzincemia. Neurology. 2002 Nov 12;59(9):1453-6. doi: 10.1212/01.wnl.0000032497.30439.f6[↩]
- Angotti LB, Post GR, Robinson NS, Lewis JA, Hudspeth MP, Lazarchick J. Pancytopenia with myelodysplasia due to copper deficiency. Pediatr Blood Cancer. 2008 Nov;51(5):693-5. doi: 10.1002/pbc.21661[↩]
- Forbes GM, Forbes A. Micronutrient status in patients receiving home parenteral nutrition. Nutrition. 1997 Nov-Dec;13(11-12):941-4. doi: 10.1016/s0899-9007(97)00334-1[↩]
- Chen CC, Takeshima F, Miyazaki T, Murase K, Ohtani H, Isomoto H, Shikuwa S, Omagari K, Mizuta Y, Ozono Y, Kohno S. Clinicopathological analysis of hematological disorders in tube-fed patients with copper deficiency. Intern Med. 2007;46(12):839-44. doi: 10.2169/internalmedicine.46.6264[↩]
- Jayakumar S, Micallef-Eynaud PD, Lyon TD, Cramb R, Jilaihawi AN, Prakash D. Acquired copper deficiency following prolonged jejunostomy feeds. Ann Clin Biochem. 2005 May;42(Pt 3):227-31. doi: 10.1258/0004563053857879[↩]
- Tümer Z, Møller LB. Menkes disease. Eur J Hum Genet. 2010 May;18(5):511-8. doi: 10.1038/ejhg.2009.187[↩]
- Menkes disease. https://medlineplus.gov/ency/article/001160.htm[↩][↩]
- Fairweather-Tait SJ, Harvey LJ, Collings R. Risk-benefit analysis of mineral intakes: case studies on copper and iron. Proc Nutr Soc. 2011 Feb;70(1):1-9. doi: 10.1017/S0029665110003873[↩]
- Prohaska JR. Impact of copper deficiency in humans. Ann N Y Acad Sci. 2014 May;1314:1-5. doi: 10.1111/nyas.12354[↩]
- Uchino K, Quang LV, Enomoto M, Nakano Y, Yamada S, Matsumura S, Kanasugi J, Takasugi S, Nakamura A, Horio T, Murakami S, Goto M, Mizuno S, Yamamoto H, Watarai M, Hanamura I, Takami A. Cytopenia associated with copper deficiency. EJHaem. 2021 Aug 26;2(4):729-737. doi: 10.1002/jha2.278[↩][↩]
- Harris ZL, Klomp LW, Gitlin JD. Aceruloplasminemia: an inherited neurodegenerative disease with impairment of iron homeostasis. Am J Clin Nutr. 1998 May;67(5 Suppl):972S-977S. doi: 10.1093/ajcn/67.5.972S[↩]
- Hellman NE, Gitlin JD. Ceruloplasmin metabolism and function. Annu Rev Nutr. 2002;22:439-58. doi: 10.1146/annurev.nutr.22.012502.114457[↩]
- Lahey ME, Gubler CJ, Chase MS, Cartwright GE, Wintrobe MM. Studies on copper metabolism. II. Hematologic manifestations of copper deficiency in swine. Blood. 1952;7(11):1053-1074. https://doi.org/10.1182/blood.V7.11.1053.1053[↩]
- Wintrobe MM, Cartwright GE, Lahey ME, Gubler CJ. The role of copper in hemopoiesis. Trans Assoc Am Physicians. 1951;64:310-315.[↩]
- Cartwright GE, Gubler CJ, Bush JA, Wintrobe MM. Studies of copper metabolism. XVII. Further observations on the anemia of copper deficiency in swine. Blood. 1956;11(2):143-153. https://doi.org/10.1182/blood.V11.2.143.143[↩]
- Bustos RI, Jensen EL, Ruiz LM, Rivera S, Ruiz S, Simon F, Riedel C, Ferrick D, Elorza AA. Copper deficiency alters cell bioenergetics and induces mitochondrial fusion through up-regulation of MFN2 and OPA1 in erythropoietic cells. Biochem Biophys Res Commun. 2013 Aug 2;437(3):426-32. doi: 10.1016/j.bbrc.2013.06.095[↩][↩]
- Peled T, Landau E, Prus E, Treves AJ, Nagler A, Fibach E. Cellular copper content modulates differentiation and self-renewal in cultures of cord blood-derived CD34+ cells. Br J Haematol. 2002 Mar;116(3):655-61. doi: 10.1046/j.0007-1048.2001.03316.x. Erratum in: Br J Haematol 2002 May;117(2):485.[↩]
- Lecumberri E, Goya L, Mateos R, Alía M, Ramos S, Izquierdo-Pulido M, Bravo L. A diet rich in dietary fiber from cocoa improves lipid profile and reduces malondialdehyde in hypercholesterolemic rats. Nutrition. 2007 Apr;23(4):332-41. doi: 10.1016/j.nut.2007.01.013[↩]
- Menkes Disease. https://rarediseases.org/rare-diseases/menkes-disease[↩]
- Fujioka K. Follow-up of nutritional and metabolic problems after bariatric surgery. Diabetes Care. 2005;28:481–484. doi: 10.2337/diacare.28.2.481[↩]
- Kumar N. Copper deficiency myelopathy (human swayback) Mayo Clin Proc. 2006;81:1371–1384. doi: 10.4065/81.10.1371[↩][↩]
- Schleper B, Stuerenburg HJ. Copper deficiency-associated myelopathy in a 46-year-old woman. J Neurol. 2001;248:705–706. doi: 10.1007/s004150170118[↩][↩]
- Bartner R, Will M, Conrad J, Engelhardt A, Schwarz-Eywill M. Pancytopenia, arthralgia and myeloneuropathy due to copper deficiency. Med Klin (Munich) 2005;100:497–501. doi: 10.1007/s00063-005-1072-7[↩][↩]
- Goldberg ME, Laczek J, Napierkowski JJ. Copper deficiency: a rare cause of ataxia following gastric bypass surgery. Am J Gastroenterol. 2008;103:1318–1319. doi: 10.1111/j.1572-0241.2007.01782_12.x[↩]
- Prodan CI, Bottomley SS, Vincent AS, Cowan LD, Greenwood-Van Meerveld B, Holland NR, Lind SE. Copper deficiency after gastric surgery: a reason for caution. Am J Med Sci. 2009;337:256–258. doi: 10.1097/MAJ.0b013e31818ad0ff[↩]
- Jaiser SR, Duddy M. Copper deficiency masquerading as subacute combined degeneration of the cord and myelodysplastic syndrome. Adv Clin Neurosci Rehabil. 2007;7:20–21.[↩]
- Jung A, Marziniak M. Copper deficiency as a treatable cause of myelopathy. Nervenarzt. 2008;79:421–425. doi: 10.1007/s00115-007-2397-7[↩]
- Rosa, F.W. 1986. Teratogen update: Penacillamine. Teratology 33(1):127–31.[↩]
- Keen, C.L., P. Mark-Savage, B. Lonnerdal, L.S. Hurley. 1983. b. Teratogenic effects of D-penicillamine in rats: relation to copper deficiency. Drug Nutr. Interact. 2(1):17–34.[↩]
- Mark-Savage, P., C.L. Keen, and L.S. Hurley. 1983. Reduction by copper supplementation of teratogenic effects of D-penicillamine. J. Nutr. 113(3):501–510.[↩]
- Jasim, S., B.R. Danielsson, H. Tjalve, and L. Dencker. 1985. Distribution of Cu in foetal and adult tissues in mice: influence of sodium diethyldithiocarbamate. Acta Pharmacol. Toxicol. (Copenhagen) 57(4): 262–270.[↩]
- Keen, C.L. 1996. Teratogenic effects of essential trace metals: deficiencies and excesses. Pp. 977–1001 in Toxicology of Metals, L.W. Chang, editor; , L. Magos, editor; , and T. Suzuki, editor. , eds. New York: CRC Press.[↩]
- Lee S.H., R. Lancey, A. Montaser, N. Madani, M.C. Linder. 1993. Ceruloplasmin and copper transport during the latter part of gestation in the rat. Proc. Soc. Exp. Biol. Med. 203(4):428–39.[↩]
- Turnlund, J.P. 1994. Copper. Pp. 231–241 in Modern Nutrition in Health and Disease, Vol. I., 8th Ed., M.E. Shils, editor; , J.A. Olson, editor; , and M. Shike, editor. , eds. Philadelphia: Lea and Febiger.[↩][↩]
- Dubick, M.A., C.L. Keen, R.A. DiSilvestro, C.D. Eskelson, J. Ireton, G.C. Hunter. 1999. Antioxidant enzyme activity in human abdominal aortic aneurysmal and occlusive disease. Proc. Soc. Exp. Biol. Med. 220(1): 39–45.[↩]
- Speich, M., A. Murat, J.L. Auget, B. Bousquet, and P. Arnaud. 1992. Magnesium, total calcium, phosphorous, copper and Zn in plasma and erythrocytes of venous cord blood from infants of diabetic mothers: Comparison with a reference group by logistic discriminant analysis. Clin Chem. 38(10):2002–2007.[↩]
- Kiilholma, P., M. Gronroos, R. Erkkola, P. Pakarinen, and V. Nanto. 1984. The role of calcium, copper, iron and zinc in preterm delivery and premature rupture of fetal membranes. Gynecol. Obstet. Invest. 17(4):194–201.[↩]
- Turnlund JR. 1999. Copper. In: Shils ME, editor; , Olson JA, editor; , Shike M, editor; , Ross AC, editor. , eds. Modern Nutrition in Health and Disease , 9th ed. Baltimore: Williams & Wilkins. Pp.241–252.[↩]
- Botash AS, Nasca J, Dubowy R, Weinberger HL, Oliphant M. 1992. Zinc-induced copper deficiency in an infant. Am J Dis Child 146:709–711[↩]
- Brewer GJ, Hill GM, Prasad AS, Cossack ZT, Rabbani P. 1983. Oral zinc therapy for Wilson’s disease. Ann Intern Med 99:314–319.[↩]
- Yuzbasiyan-Gurkan V, Grider A, Nostrant T, Cousins RJ, Brewer GJ. 1992. Treatment of Wilson’s disease with zinc: X. Intestinal metallothionein induction. J Lab Clin Med 120:380–386.[↩]
- August D, Janghorbani M, Young VR. 1989. Determination of zinc and copper absorption at three dietary Zn-Cu ratios by using stable isotopic methods in young adult and elderly subjects. Am J Clin Nutr 50:1457–1463.[↩]
- Haschke F, Ziegler EE, Edwards BB, Foman SJ. 1986. Effect of iron fortification of infant formula on trace mineral absorption. J Pediatr Gastroenterol Nutr 5:768–773.[↩]
- Lonnerdal B, Hernell O. 1994. Iron, zinc, copper and selenium status of breast-fed infants and infants fed trace element fortified milk-based infant formula. Acta Paediatr 83:367–373.[↩]
- Fields M, Ferretti RJ, Smith JC, Reiser S. 1984. The interaction of type of dietary carbohydrates with copper deficiency. Am J Clin Nutr 39:289–295.[↩]
- Schoenemann HM, Failla ML, Steele NC. 1990. Consequences of severe copper deficiency are independent of dietary carbohydrate in young pigs. Am J Clin Nutr 52:147–154.[↩]
- Reiser S, Smith JC, Mertz W, Holbrook JT, Scholfield DJ, Powell AS, Canfield WK, Canary JJ. 1985. Indices of copper status in humans consuming a typical American diet containing either fructose or starch. Am J Clin Nutr 42:242–251.[↩]
- Mercer, J.F. 1998. Menkes syndrome and animal models. Am. J. Clin. Nutr. 67(5 Suppl.):1022S–1028S.[↩]
- Kaler, S.G. 1998. Diagnosis and therapy of Menkes syndrome, a genetic form of copper deficiency. Am. J. Clin. Nutr. 67(5 Suppl.):1029S–1034S[↩]
- Prohaska, J.R., T. Tamura, A.K. Percy, and J.R. Turnlund. 1997. In vitro copper stimulation of plasma peptidylglycine a-amidating monooxygenase in Menkes disease variant with occipital horns. Pediatr. Res. 42(6):862–865.[↩]
- Botero-López JE, Araya M, Parada A, Méndez MA, Pizarro F, Espinosa N, Canales P, Alarcón T. Micronutrient deficiencies in patients with typical and atypical celiac disease. J Pediatr Gastroenterol Nutr. 2011 Sep;53(3):265-70. doi: 10.1097/MPG.0b013e3181f988fc[↩]
- Rubio-Tapia A, Hill ID, Kelly CP, Calderwood AH, Murray JA; American College of Gastroenterology. ACG clinical guidelines: diagnosis and management of celiac disease. Am J Gastroenterol. 2013 May;108(5):656-76; quiz 677. doi: 10.1038/ajg.2013.7[↩]
- Costa LS, Pegler SP, Lellis RF, Krebs VL, Robertson S, Morgan T, Honjo RS, Bertola DR, Kim CA. Menkes disease: importance of diagnosis with molecular analysis in the neonatal period. Rev Assoc Med Bras (1992). 2015 Sep-Oct;61(5):407-10. doi: 10.1590/1806-9282.61.05.407[↩]
- Kaler SG. Neurodevelopment and brain growth in classic Menkes disease is influenced by age and symptomatology at initiation of copper treatment. J Trace Elem Med Biol. 2014 Oct;28(4):427-30. doi: 10.1016/j.jtemb.2014.08.008[↩]
- Kim BE, Nevitt T, Thiele DJ. Mechanisms for copper acquisition, distribution and regulation. Nat Chem Biol. 2008 Mar;4(3):176-85. doi: 10.1038/nchembio.72[↩]
- Jaiser SR, Winston GP. Copper deficiency myelopathy. J Neurol. 2010 Jun;257(6):869-81. doi: 10.1007/s00415-010-5511-x[↩]
- Institute of Medicine (US) Panel on Micronutrients. Dietary Reference Intakes for Vitamin A, Vitamin K, Arsenic, Boron, Chromium, Copper, Iodine, Iron, Manganese, Molybdenum, Nickel, Silicon, Vanadium, and Zinc. Washington (DC): National Academies Press (US); 2001. 7, Copper. Available from: https://www.ncbi.nlm.nih.gov/books/NBK222312[↩]
- Wasa M, Satani M, Tanano H, Nezu R, Takagi Y, Okada A. Copper deficiency with pancytopenia during total parenteral nutrition. JPEN J Parenter Enteral Nutr. 1994;18:190–192. doi: 10.1177/0148607194018002190[↩]
- Angotti LB, Post GR, Robinson NS, Lewis JA, Hudspeth MP, Lazarchick J. Pancytopenia with myelodysplasia due to copper deficiency. Pediatr Blood Cancer. 2008 Jul 11[↩]
- Kumar N, Ahlskog JE, Klein CJ, Port JD. Imaging features of copper deficiency myelopathy: a study of 25 cases. Neuroradiology. 2006;48:78–83. doi: 10.1007/s00234-005-0016-5[↩]
- Bennetts HW, Chapman FE. Copper deficiency in sheep in Western Australia: a preliminary account of the aetiology of enzootic ataxia of lambs and anaemia of ewes. Aust Vet J. 1937;13:138–149. doi: 10.1111/j.1751-0813.1937.tb04108.x[↩]
- Gregg XT, Reddy V, Prchal JT. Copper deficiency masquerading as myelodysplastic syndrome. Blood. 2002;100:1493–1495. doi: 10.1182/blood-2002-01-0256[↩]
- Weihl CC, Lopate G. Motor neuron disease associated with copper deficiency. Muscle Nerve. 2006;34:789–793. doi: 10.1002/mus.20631[↩]
- Kumar N, Low PA. Myeloneuropathy and anemia due to copper malabsorption. J Neurol. 2004;251:747–749. doi: 10.1007/s00415-004-0428-x[↩]
- Prodan CI, Holland NR, Wisdom PJ, Burstein SA, Bottomley SS. CNS demyelination associated with copper deficiency and hyperzincemia. Neurology. 2002;59:1453–1456. doi: 10.1001/archneur.59.9.1453[↩]
- Nations SP, Boyer PJ, Love LA, Burritt MF, Butz JA, Wolfe GI, Hynan LS, Reisch J, Trivedi JR. Denture cream: an unusual source of excess zinc, leading to hypocupremia and neurologic disease. Neurology. 2008;71:639–643. doi: 10.1212/01.wnl.0000312375.79881.94[↩]
- Spinazzi M, De Lazzari F, Tavolato B, Angelini C, Manara R, Armani M. Myelo-optico-neuropathy in copper deficiency occurring after partial gastrectomy. Do small bowel bacterial overgrowth syndrome and occult zinc ingestion tip the balance? J Neurol. 2007;254:1012–1017. doi: 10.1007/s00415-006-0479-2[↩]
- Prodan CI, Holland NR, Wisdom PJ, Burstein SA, et al. CNS demyelination associated with copper deficiency and hyperzincemia. Neurology. 2002;59:1453–1456[↩]
- Prodan CI, Holland NR, Wisdom PJ, Burstein SA, et al. CNS demyelination associated with copper deficiency and hyperzincemia. Neurology. 2002;59:1453–1456.[↩]
- Bertinato J, Abbe MRL. Maintaining copper homeostasis: regulation of copper-traficking proteins in response to copper deficiency or overload. J Nutri Biochem. 2004;15:316–322.[↩]
- Jaiser SR, Winston GP. Copper deficiency myelopathy. J Neurol. 2010;257(6):869-81. https://www.ncbi.nlm.nih.gov/pmc/articles/PMC3691478/[↩]
- Prodan CI, Bottomley SS, Holland NR, Lind SE. Relapsing hypocupraemic myelopathy requiring high-dose oral copper replacement. J Neurol Neurosurg Psychiatry. 2006;77:1092–1093. doi: 10.1136/jnnp.2006.096883[↩]