Contents
- Spinal cord injury
- What happens when the spinal cord is injured ?
How does the spinal cord work ?
To understand what can happen as the result of a spinal cord injury, it is important to understand the anatomy of the spinal cord and its normal functions.
The spinal cord is a tight bundle of neural cells (neurons and glia) and nerve pathways (axons) that extend from the base of the brain to the lower back. It is the primary information highway that receives sensory information from the skin, joints, internal organs, and muscles of the trunk, arms, and legs, which is then relayed upward to the brain. It also carries messages downward from the brain to other body systems. Millions of nerve cells situated in the spinal cord itself also coordinate complex patterns of movements such as rhythmic breathing and walking. Together, the spinal cord and brain make up the central nervous system (CNS), which controls most functions of the body.
Figure 1. Spinal cord anatomy
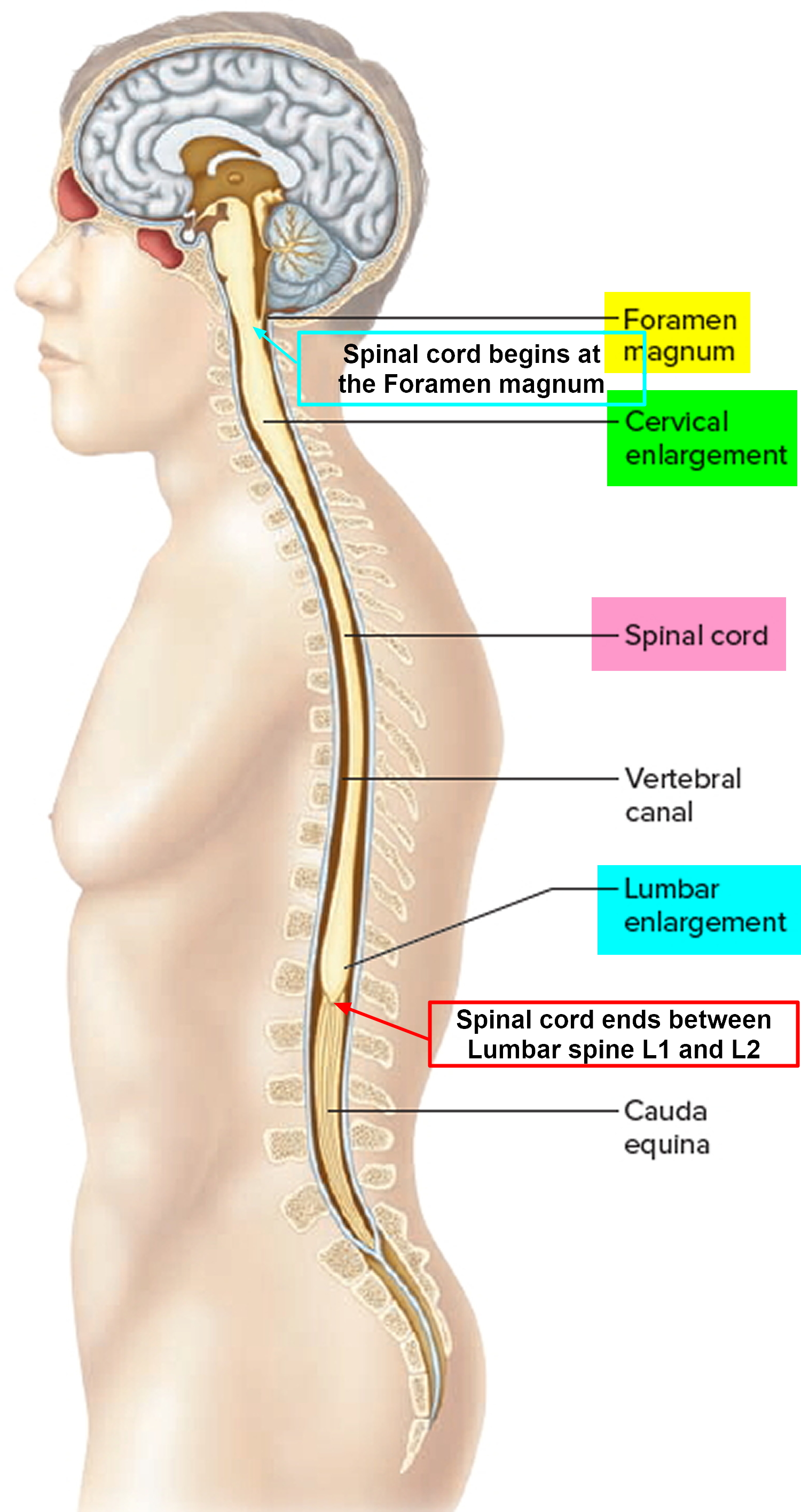
The spinal cord is made up of neurons, glia, and blood vessels. The neurons and their dendrites (branching projections that receive input from axons of other neurons) reside in an H-shaped or butterfly-shaped region called gray matter. The gray matter of the cord contains lower motor neurons, which branch out from the cord to muscles, internal organs, and tissue in other parts of the body and transmit information commands to start and stop muscle movement that is under voluntary control. Upper motor neurons are located in the brain and send their long processes (axons) to the spinal cord neurons. Other types of nerve cells found in dense clumps of cells that sit just outside the spinal cord (called sensory ganglia) relay information such as temperature, touch, pain, vibration, and joint position back to the brain.
The axons carry signals up and down the spinal cord and to the rest of the body. Thousands of axons are bundled into pairs of spinal nerves that link the spinal cord to the muscles and the rest of the body. The function of these nerves reflects their location along the spinal cord.
- Cervical spinal nerves (C1 to C8) emerge from the spinal cord in the neck and control signals to the back of the head, the neck and shoulders, the arms and hands, and the diaphragm.
- Thoracic spinal nerves (T1 to T12) emerge from the spinal cord in the upper mid-back and control signals to the chest muscles, some muscles of the back, and many organ systems, including parts of the abdomen.
- Lumbar spinal nerves (L1 to L5) emerge from the spinal cord in the low back and control signals to the lower parts of the abdomen and the back, the buttocks, some parts of the external genital organs, and parts of the leg.
- Sacral spinal nerves (S1 to S5) emerge from the spinal cord in the low back and control signals to the thighs and lower parts of the legs, the feet, most of the external genital organs, and the area around the anus.
Figure 2. Spinal cord segments
The outcome of any injury to the spinal cord depends upon the level at which the injury occurs in the neck or back and how many and which axons and cells are damaged; the more axons and cells that survive in the injured region, the greater the amount of function recovery. Loss of neurologic function occurs below the level of the injury, so the higher the spinal injury, the greater the loss of function.
Each spinal nerve except C1 receives sensory input from a specific area of skin called a dermatome. A dermatome map is a diagram of the cutaneous regions innervated by each spinal nerve (see Figure 3). Such a map is oversimplified, however, because the dermatomes overlap at their edges by as much as 50%. Therefore, severance of one sensory nerve root does not entirely deaden sensation from a dermatome. It is necessary to sever or anesthetize three sequential spinal nerves to produce a total loss of sensation from one dermatome. Spinal nerve damage is assessed by testing the dermatomes with pinpricks and noting areas in which the patient has no sensation.
Figure 3. Dermatome (spinal nerves sensory innvervation)
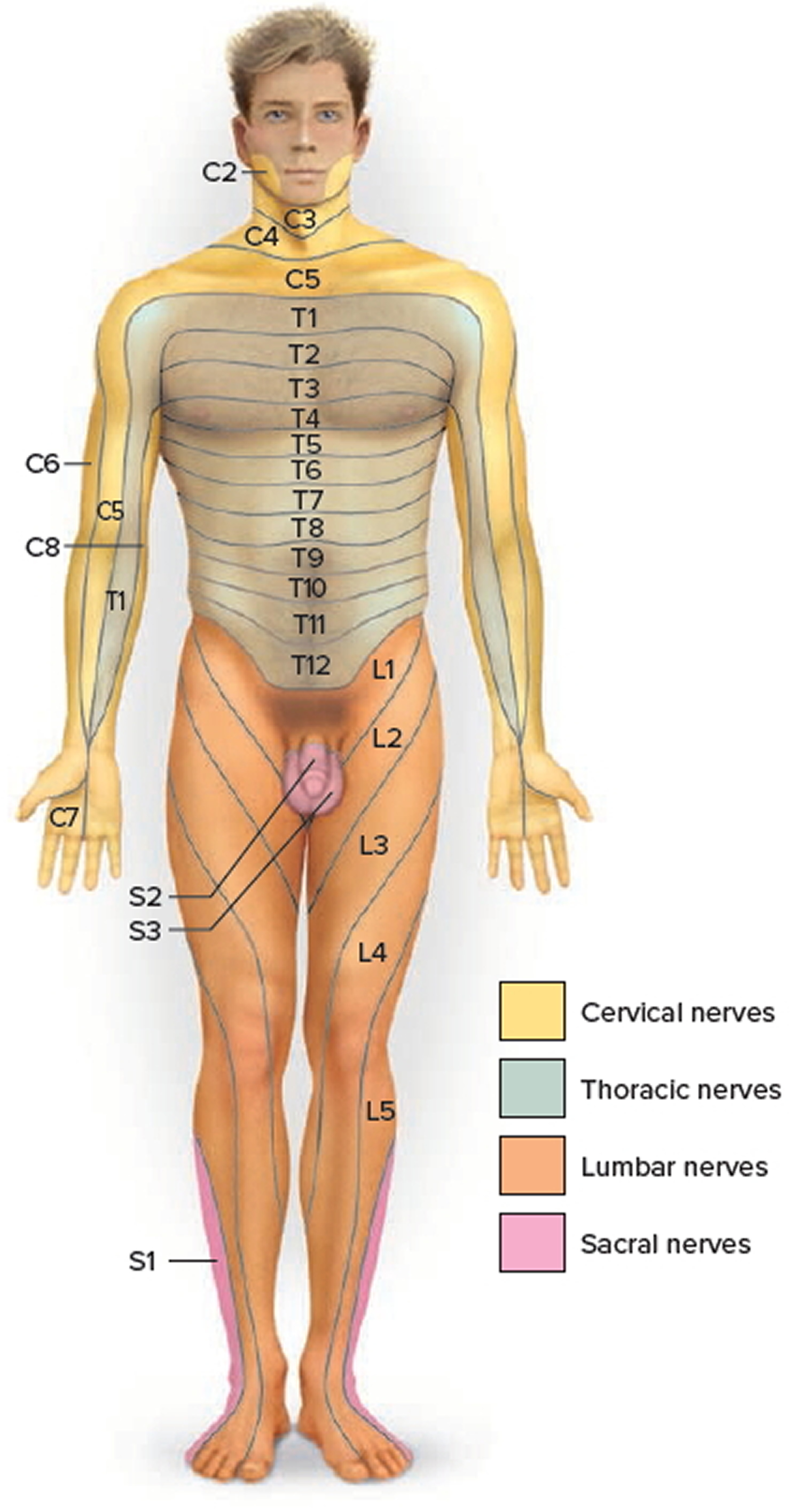
Cross-Sectional Anatomy of Spinal Cord
The spinal cord, like the brain, consists of two kinds of nervous tissue called gray and white matter. Gray matter has a relatively dull color because it contains little myelin. It contains the somas, dendrites, and proximal parts of the axons of neurons. It is the site of synaptic contact between neurons, and therefore the site of all neural integration in the spinal cord. White matter, by contrast, is whitish containing a mixture of proteins and fat-like substances called myelin covering the axons and allows electrical signals to flow quickly and freely. Myelin is much like the insulation around electrical wires. The white matter is composed of bundles of axons, called tracts, that carry signals from one level of the central nervous system (CNS) to another. It is formed by axon-insulating cells called oligodendrocytes. Because of its whitish color, the outer section of the spinal cord—which is formed by bundles of myelinated axons—is called white matter. Both gray and white matter also have an abundance of glial cells.
Figure 4. Spinal cord cross section
Spinal Cord Tracts
Knowledge of the locations and functions of the spinal tracts is essential in diagnosing and managing spinal cord injuries.
Ascending tracts carry sensory information up the cord, and descending tracts conduct motor impulses down. All nerve fibers in a given tract have a similar origin, destination, and function. Many of these fibers have their origin or destination in a region called the brainstem. Described more fully in the human brain article.
Several of these tracts undergo decussation as they pass up or down the brainstem and spinal cord—meaning that they cross over from the left side of the body to the right, or vice versa. As a result, the left side of the brain receives sensory information from the right side of the body and sends motor commands to that side, while the right side of the brain senses and controls the left side of the body. Therefore, a stroke that damages motor centers of the right side of the brain can cause paralysis of the left limbs and vice versa.
When the origin and destination of a tract are on opposite sides of the body, anatomists say they are contralateral to each other. When a tract does not decussate, its origin and destination are on the same side of the body and anatomists say they are ipsilateral. Bear in mind that each tract is repeated on the right and left sides of the spinal cord.
Figure 5. Spinal cord tracts
Figure 6. Processing of sensory input and motor output by the spinal cord
Note: Sensory input is conveyed from sensory receptors to the posterior gray horns of the spinal cord, and motor output is conveyed from the anterior and lateral gray horns of the spinal cord to effectors (muscles and glands).
Ascending Tracts
Ascending tracts carry sensory signals up the spinal cord. Sensory signals typically travel across three neurons from their origin in the receptors to their destination in the brain: a first-order neuron that detects a stimulus and transmits a signal to the spinal cord or brainstem; a second-order neuron that continues as far as a “gateway” called the thalamus at the upper end of the brainstem; and a third-order neuron that carries the signal the rest of the way to the cerebral cortex. The axons of these neurons are called the first- through third-order nerve fibers.
Figure 7. Spinal cord ascending tracts to the brain
Descending Tracts
Descending tracts carry motor signals down the brainstem and spinal cord. A descending motor pathway typically involves two neurons called the upper and lower motor neurons. The upper motor neuron begins with a soma in the cerebral cortex or brainstem and has an axon that terminates on a lower motor
neuron in the brainstem or spinal cord. The axon of the lower motor neuron then leads the rest of the way to the muscle or other target organ. The names of most descending tracts consist of a word root denoting the point of origin in the brain, followed by the suffix -spinal.
Figure 8. Spinal cord descending tracts from the brain
Protective Structures of the spinal cord
The nervous tissue of the central nervous system (CNS) is very delicate and does not respond well to injury or damage. Accordingly, nervous tissue requires considerable protection. The first layer of protection for the central nervous system is the hard bony skull and vertebral column. The skull encases the brain and the vertebral column surrounds the spinal cord, providing strong protective defenses against damaging blows or bumps. The spinal cord is located within the vertebral canal of the vertebral column. The surrounding vertebrae provide a sturdy shelter for the enclosed spinal cord. The vertebral ligaments, meninges, and cerebrospinal fluid provide additional protection. The second protective layer is the meninges, three membranes that lie between the bony encasement and the nervous tissue in both the brain and spinal cord. Finally, a space between two of the meningeal membranes contains cerebrospinal fluid, a buoyant liquid that suspends the central nervous tissue in a weightless environment while surrounding it with a shock-absorbing, hydraulic cushion.
Figure 9. Vertebral column
Figure 10. Spinal cord anatomy
The spinal cord, like the brain, is enclosed in three membrane layers called meninges: the dura mater (the tougher, most protective, outermost layer); the arachnoid (middle layer); and the pia mater (innermost and very delicate). The soft, gel-like spinal cord is protected by 33 rings of bone called vertebrae, which form the spinal column. Each vertebra has a circular hole, so when the rung-like bones are stacked one on top of the other there is a long hollow channel, with the spinal cord inside that channel. The vertebrae are named and numbered from top to bottom according to their location along the backbone: seven cervical vertebrae (C1-C7) are in the neck; twelve thoracic vertebrae (T1-T12) attach to the ribs; five lumbar vertebrae (L1-L5) are in the lower back; and, below them, five sacral vertebrae (S1-S5) that connect to the pelvis. The adult spinal cord is shorter than the spinal column and generally ends at the L1-L2 vertebral body level. A thick set of nerves from the lumbar and sacral cord form the “cauda equina” in the spinal canal below the cord.
The spinal column is not all bone. Between the vertebrae are discs of semi-rigid cartilage and narrow spaces called foramen that act as passages through which the spinal nerves travel to and from the rest of the body. These are places where the spinal cord is particularly vulnerable to direct injury.
Figure 11. Spinal nerve
Figure 12. Spinal nerve fiber anatomy
Spinal cord blood supply
The arterial supply to the spinal cord comes from two sources. It consists of:
- The longitudinal vessels.
- Segmental spinal arteries.
The longitudinal vessels consist of:
- A single anterior spinal artery, which originates within the cranial cavity as the union of two vessels that arise from the vertebral arteries-the resulting single anterior spinal artery passes inferiorly, approximately parallel to the anterior median fissure, along the surface of the spinal cord; and
- Two posterior spinal arteries, which also originate in the cranial cavity, usually arising directly from a terminal branch of each vertebral artery (the posterior inferior cerebellar artery)-the right and left posterior spinal arteries descend along the spinal cord, each as two branches that bracket the posterolateral sulcus and the connection of posterior roots with the spinal cord.
The anterior and posterior spinal arteries are reinforced along their length by eight to ten segmental medullary arteries. The largest of these is the arteria radicularis magna or the artery of Adamkiewicz. This vessel arises in the lower thoracic or upper lumbar region, usually on the left side, and reinforces the arterial supply to the lower portion of the spinal cord, including the lumbar enlargement.
Segmental spinal arteries
Segmental spinal arteries arise predominantly from the vertebral and deep cervical arteries in the neck, the posterior intercostal arteries in the thorax, and the lumbar arteries in the abdomen.
After entering an intervertebral foramen, the segmental spinal arteries give rise to anterior and posterior radicular arteries. This occurs at every vertebral level. The radicular arteries follow, and supply, the anterior and posterior roots. At various vertebral levels, the segmental spinal arteries also give off segmental medullary arteries. These vessels pass directly to the longitudinally oriented vessels, reinforcing these.
Figure 13. Spinal cord blood supply
Spinal cord injury
As mentioned earlier, the vertebrae normally protect the soft tissues of the spinal cord, but they can be broken or dislocated in a variety of ways that puts harmful pressure on the spinal cord. Injuries can occur at any level of the spinal cord. The segment of the cord that is injured, and the severity of the damage to the nervous tissue, will determine which body functions are compromised or lost. An injury to a part of the spinal cord causes physiological consequences to parts of the body controlled by nerves at and below the level of the injury.
Spinal cord injuries are classified as either complete or incomplete. An incomplete injury means that the ability of the spinal cord to convey messages to or from the brain is not completely lost. People with incomplete injuries retain some motor or sensory function below the injury. A complete injury is indicated by a total lack of sensory and motor function below the level of injury. People who survive a spinal cord injury will most likely have medical complications such as chronic pain and bladder and bowel dysfunction, along with an increased susceptibility to respiratory and heart problems. Successful recovery depends upon how well these chronic conditions are handled day to day.
Your ability to control your limbs after spinal cord injury depends on two factors:
- the place of the injury along your spinal cord and
- the severity of injury to the spinal cord.
The lowest part of your spinal cord that functions normally after injury is referred to as the neurological level of your injury. The severity of the injury is often called “the completeness” and is classified as either of the following:
- Complete. If almost all feeling (sensory) and all ability to control movement (motor function) are lost below the spinal cord injury, your injury is called complete.
- Incomplete. If you have some motor or sensory function below the affected area, your injury is called incomplete. There are varying degrees of incomplete injury.
Additionally, paralysis from a spinal cord injury may be referred to as:
- Tetraplegia. Also known as quadriplegia, this means your arms, hands, trunk, legs and pelvic organs are all affected by your spinal cord injury.
- Paraplegia. This paralysis affects all or part of the trunk, legs and pelvic organs.
Spinal cord injuries of any kind may result in one or more of the following signs and symptoms:
- Loss of movement
- Loss of sensation, including the ability to feel heat, cold and touch
- Loss of bowel or bladder control
- Exaggerated reflex activities or spasms
- Changes in sexual function, sexual sensitivity and fertility
- Pain or an intense stinging sensation caused by damage to the nerve fibers in your spinal cord
- Difficulty breathing, coughing or clearing secretions from your lungs.
Emergency signs and symptoms of spinal cord injury after an accident may include 1:
- Extreme back pain or pressure in your neck, head or back
- Weakness, incoordination or paralysis in any part of your body
- Numbness, tingling or loss of sensation in your hands, fingers, feet or toes
- Loss of bladder or bowel control
- Difficulty with balance and walking
- Impaired breathing after injury
- An oddly positioned or twisted neck or back.
Motor vehicle accidents and catastrophic falls are the most common causes of physical trauma that breaks, crushes, or presses on the vertebrae and can cause irreversible damage at the corresponding level of the spinal cord and below. Severe trauma to the cervical cord results in paralysis of most of the body, including the arms and legs, and is called tetraplegia 2. Trauma to the thoracic nerves in the upper, middle, or lower back results in paralysis of the trunk and lower extremities, called paraplegia.
Penetrating injuries, such as gunshot or knife wounds, damage the spinal cord; however, most traumatic injuries do not completely sever the spinal cord. Instead, an injury is more likely to cause fractures and compression of the vertebrae, which then crush and destroy the axons that carry signals up and down the spinal cord 2. A spinal cord injury can damage a few, many, or almost all of the axons that cross the site of injury. A variety of cells located in and around the injury site may also die. Some injuries in which there is little or no nerve cell death but only pressure-induced blockage of nerve signaling or only demyelination without axonal damage will allow almost complete recovery. Others in which there is complete cell death across even a thin horizontal level of the spinal cord will result in complete paralysis.
Surgery to relieve compression of the spinal tissue by surrounding bones broken or dislocated by the injury is often necessary, through timing of such surgery may vary widely. A recent prospective multicenter trial called STASCIS is exploring whether performing decompression surgery early (less than 24 hours following injury) can improve outcomes for patients with bone fragments or other tissues pressing on the spinal cord.
Today, improved emergency care for people with spinal cord injuries, antibiotics to treat infections, and aggressive rehabilitation can minimize damage to the nervous system and restore function to varying degrees 2. Advances in research are giving doctors and people living with spinal cord injury hope that spinal cord injuries will eventually be repairable. With new surgical techniques and developments in spinal nerve regeneration, cell replacement, neuroprotection, and neurorehabilitation, the future for spinal cord injury survivors looks brighter than ever.
When to see a doctor ?
Anyone who experiences significant trauma to his or her head or neck needs immediate medical evaluation for the possibility of a spinal injury. In fact, it’s safest to assume that trauma victims have a spinal injury until proven otherwise because:
- A serious spinal injury isn’t always immediately obvious. If it isn’t recognized, more severe injury may occur.
- Numbness or paralysis may develop immediately or come on gradually as bleeding or swelling occurs in or around the spinal cord.
- The time between injury and treatment can be critical in determining the extent of complications and the amount of recovery.
If you suspect that someone has a back or neck injury:
- Don’t move the injured person — permanent paralysis and other serious complications may result
- Call your local emergency medical assistance number
- Keep the person still
- Place heavy towels on both sides of the neck or hold the head and neck to prevent them from moving until emergency care arrives
- Provide basic first aid, such as stopping any bleeding and making the person comfortable, without moving the head or neck
Spinal cord injuries result from damage to the vertebrae, ligaments or disks of the spinal column or to the spinal cord itself.
A traumatic spinal cord injury may stem from a sudden, traumatic blow to your spine that fractures, dislocates, crushes, or compresses one or more of your vertebrae. It also may result from a gunshot or knife wound that penetrates and cuts your spinal cord.
Additional damage usually occurs over days or weeks because of bleeding, swelling, inflammation and fluid accumulation in and around your spinal cord.
A nontraumatic spinal cord injury may be caused by arthritis, cancer, inflammation, infections or disk degeneration of the spine.
Facts and Figures about Spinal Cord Injury 2:
- There are an estimated 12,000 spinal cord injuries every year in the United States alone.
- More than a quarter of a million Americans are currently living with spinal cord injuries.
- The cost of managing the care of spinal cord injury patients is $3 billion each year.
- The largest proportion of spinal cord injuries (36.5 percent) occurs during car accidents; more than a quarter are the result of falls; and the rest are due to acts of violence (primarily gunshot wounds), sporting accidents, and other less common causes.
- The average age at injury has risen and is now 42.6 years.
- 80 percent of spinal cord injury patients are men.
Common causes of spinal cord injury
The most common causes of spinal cord injuries in the United States are:
- Motor vehicle accidents. Auto and motorcycle accidents are the leading cause of spinal cord injuries, accounting for more than 35 percent of new spinal cord injuries each year.
- Falls. Spinal cord injury after age 65 is most often caused by a fall. Overall, falls cause more than one-quarter of spinal cord injuries.
- Acts of violence. Around 15 percent of spinal cord injuries result from violent encounters, often involving gunshot and knife wounds, according to the National Spinal Cord Injury Statistical Center.
- Sports and recreation injuries. Athletic activities, such as impact sports and diving in shallow water, cause about 9 percent of spinal cord injuries.
- Alcohol. Alcohol use is a factor in about 1 out of every 4 spinal cord injuries.
- Diseases. Cancer, arthritis, osteoporosis and inflammation of the spinal cord also can cause spinal cord injuries.
Although a spinal cord injury is usually the result of an accident and can happen to anyone, certain factors may predispose you to a higher risk of sustaining a spinal cord injury, including:
- Being male. Spinal cord injuries affect a disproportionate amount of men. In fact, females account for only about 20 percent of traumatic spinal cord injuries in the United States.
- Being between the ages of 16 and 30. You’re most likely to suffer a traumatic spinal cord injury if you’re between the ages of 16 and 30.
- Being older than 65. Falls cause most injuries in older adults.
- Engaging in risky behavior. Diving into too-shallow water or playing sports without wearing the proper safety gear or taking proper precautions can lead to spinal cord injuries. Motor vehicle crashes are the leading cause of spinal cord injuries for people under 65.
- Having a bone or joint disorder. A relatively minor injury can cause a spinal cord injury if you have another disorder that affects your bones or joints, such as arthritis or osteoporosis.
Following this advice may reduce your risk of a spinal cord injury:
- Drive safely. Car crashes are one of the most common causes of spinal cord injuries. Wear a seat belt every time you drive or ride in a car.
- Make sure that your children wear a seat belt or use an age- and weight-appropriate child safety seat. To protect them from air bag injuries, children under age 12 should always ride in the back seat.
- Check water depth before diving. To make sure you don’t dive into shallow water, don’t dive into a pool unless it’s 9 feet (about 3 meters) or deeper, don’t dive into an aboveground pool and don’t dive into any water of which you don’t know the depth.
- Prevent falls. Use a step stool with a grab bar to reach objects in high places. Add handrails along stairways. Put nonslip mats on tile floors and in the tub or shower. For young children, use safety gates to block stairs and consider installing window guards.
- Take precautions when playing sports. Always wear recommended safety gear. Avoid leading with your head in sports. For example, don’t slide headfirst in baseball, and don’t tackle using the top of your helmet in football. Use a spotter for new moves in gymnastics.
- Don’t drink and drive. Don’t drive while intoxicated or under the influence of drugs. Don’t ride with a driver who’s been drinking.
What happens when the spinal cord is injured ?
Traumatic spinal cord injury usually begins with a sudden, mechanical blow or rupture to the spine that fractures or dislocates vertebrae. The damage begins at the moment of primary injury, when the cord is stretched or displaced by bone fragments or disc material. Nerve signaling stops immediately but may not return rapidly even if there is no structural damage to the cord. In severe injury, axons are cut or damaged beyond repair, and neural cell membranes are broken. Blood vessels may rupture and cause bleeding into the spinal cord’s central tissue, or bleeding can occur outside the cord, causing pressure by the blood clot on the cord.
Within minutes, the spinal cord near the site of severe injury swells within the spinal canal. This may increase pressure on the cord and cut blood flow to spinal cord tissue. Blood pressure can drop, sometimes dramatically, as the body loses its ability to self-regulate. All these changes can cause a condition known as spinal shock that can last from several hours to several days 2.
There is some controversy among neurologists about the extent and impact of spinal shock, and even its definition in terms of physiological characteristics 2. It appears to occur in approximately half of the cases of spinal cord injury and is usually directly related to the size and severity of the injury. During spinal shock, the entire spinal cord below the lesion becomes temporarily disabled, causing complete paralysis, loss of all reflexes, and loss of sensation below the affected cord level.
The primary injury initiates processes that continue for days or weeks. It sets off a cascade of biochemical and cellular events that kills neurons, strips axons of their protective myelin covering, and triggers an inflammatory immune system response. This is the beginning of the secondary injury process 2. Days, or sometimes even weeks later, after this second wave of damage has passed, the area of destruction has increased—sometimes to several segments above and below the original injury.
Changes in blood flow cause ongoing damage. The major reduction in blood flow to the site following the initial injury can last for as long as 24 hours and become progressively worse if there is continued compression of the cord due to swelling or bleeding. Because of the greater blood flow needs of gray matter, the impact is greater on the central cord than on the outlying white matter. Blood vessels in the gray matter also become leaky, sometimes as early as 5 minutes after injury, which initiates spinal cord swelling. Cells that line the still-intact blood vessels in the spinal cord also begin to swell, and this further reduces blood flow to the injured area. The combination of leaking, swelling, and sluggish blood flow prevents the normal delivery of oxygen and nutrients to neurons, causing many of them to die 2.
Excessive release of neurotransmitters kills nerve cells 2. After the injury, an excessive release of neurotransmitters (chemicals that allow neurons to signal each other) can cause additional damage by over-stimulating nerve cells. The neurotransmitter glutamate is commonly used by axons in the spinal cord to stimulate activity in other neurons. But when spinal cells are injured, their axons flood the area with glutamate and trigger additional nerve cell damage. This process kills neurons near the injury site and the myelin-forming oligodendrocytes at and beyond the injured area.
An invasion of immune system cells creates inflammation. Under normal conditions, the blood-brain barrier keeps potentially destructive immune system cells from entering the brain or spinal cord. This barrier is a naturally-occurring result of closely spaced cells along the blood vessels that prevent many substances from leaving the blood and entering brain tissues. But when the blood-brain barrier breaks down, immune system cells—primarily white blood cells—can invade the spinal cord tissue and trigger an inflammatory response. This inflammatory response can cause additional damage to some neurons and may kill others.
Free radicals attack nerve cells. Another consequence of inflammation is the increased production of highly reactive forms of oxygen molecules called free radicals—chemicals that modify the chemical structure of other molecules in damaging ways, for example, damaging cell membranes. Free radicals are produced naturally as a by-product of normal oxygen metabolism in small enough amounts that they cause no harm. But injury to the spinal cord causes cells to overproduce free radicals, which destroy critical molecules of the cell.
Nerve cells self-destruct. For reasons that are still unclear, spinal cord injury sets off apoptosis—a normal process of cell death that helps the body get rid of old and unhealthy cells. Apoptosis kills oligodendrocytes in damaged areas of the spinal cord days to weeks after the injury. Apoptosis can strip myelin from intact axons in adjacent ascending and descending pathways, causing the axons to become dysfunctional and disrupting the spinal cord’s ability to communicate with the brain.
Scarring occurs. Following a spinal cord injury, astrocytes (star-shaped glial cells that support the brain and spinal cord) wall off the injury site by forming a scar, which creates a physical and chemical barrier to any axons which could potentially regenerate and reconnect. Even if some intact myelinated axons remain, there may not be enough to convey any meaningful information to or from the brain.
Researchers are especially interested in studying the mechanisms of this wave of secondary damage because finding ways to stop it could save spinal cord tissue and thereby enable greater functional recovery.
How are spinal cord injuries diagnosed ?
The emergency room physician will test the individual to see if there is any movement or sensation at or below the level of injury. Methods to assess autonomic function also have been established (American Spinal Injury Association).
Figure 14. International Standards for Neurological Classification of Spinal Cord Injury
[Source 3]Emergency medical tests for a spinal cord injury include:
Magnetic resonance imaging (MRI), which uses computer-generated radio waves and a powerful magnetic field to produce detailed three-dimensional images of body structures, including tissues, organs, bones, and nerves. It can document brain and spinal trauma from injury, as well as aid in diagnosing brain and spinal cord tumors, herniated disks, vascular (blood vessel) irregularities, bleeding and inflammation that might compress the spine and spinal cord, and injury to the ligaments that support the cervical spine.
Computerized tomography (CT) provides rapid, clear two-dimensional x-ray images of organs, bones, and tissues. Neurological CT scans are used to view the brain and spine. CT is excellent in detecting bone fractures, bleeding, and spinal stenosis (narrowing of the spinal canal), but CT has less ability to image the spinal cord or identify ligament injury associated with an unstable spine than MRI.
Plane x-rays (which demonstrate the planes of bone on bone) of the person’s chest and skull are often taken as part of a neurological work-up. X-rays can be used to view most parts of the body, such as a joint or major organ system. In a conventional x-ray, a concentrated burst of low-dose ionized radiation is passed through the body and onto a photographic plate. Since calcium in bones absorbs x-rays more easily than soft tissue or muscle, the bony structure appears white on the film. Vertebral misalignment or fracture can be seen within minutes. X-rays taken in different neck positions (i.e., flexion and extension views) detect instability of the cervical spine. Tissue masses such as injured ligaments or a bulging disc are not visible on conventional x-rays.
Types of spinal cord injury
Once the swelling from within and around the spinal cord has eased a bit—usually within a week to 10 days—physicians will conduct a complete neurological exam to classify the injury as complete or incomplete. An incomplete injury means that the ability of the spinal cord to convey messages to or from the brain is not completely lost. People with incomplete injuries retain some sensory function and may have voluntary motor activity below the injury site. A complete injury prevents nerve communications from the brain and spinal cord to parts of the body below the injury site. There is a total lack of sensory and motor function below the level of injury, even if the spinal cord was not completely severed. Studies have shown that people with incomplete injuries have a greater chance of recovering some function in the affected limbs than those with a complete injury.
Physicians use the International Standards of Neurologic Classification of Spinal Cord Injury to measure the extent of neurologic injury following a spinal cord injury. The American Spinal Injury Association Impairment Scale (AIS) is used to categorize the degrees of injury into different groups.
Table 1. American Spinal Injury Association Impairment Scale
Classification | Description | |
---|---|---|
A | Complete: no motor or sensory function is preserved below the level of injury, including the sacral segments S4-S5 | |
B | Incomplete: sensory, but not motor, function is preserved below the neurologic level and some sensation in the sacral segments S4-S5 | |
C | Incomplete: motor function is preserved below the neurologic level, however, more than half of key muscles below the neurologic level have a muscle grade less than 3 (i.e., not strong enough to move against gravity) | |
D | Incomplete: motor function is preserved below the neurologic level, and at least half of key muscles below the neurologic level have a muscle grade of 3 or more (i.e., joints can be moved against gravity) | |
E | Normal: motor and sensory functions are normal |
How does a spinal cord injury affect the rest of the body and how is it treated ?
People who survive a spinal cord injury often have medical complications resulting in bladder, bowel, and sexual dysfunction. They may also develop chronic pain, autonomic dysfunction, and spasticity (increased tone in and contractions of muscles of the arms and legs) , but this is highly variable and poorly understood. Higher levels of injury may have an increased susceptibility to respiratory and heart problems.
Breathing. A spinal cord injury high in the neck can affect the nerves and muscles in the neck and chest that are involved with breathing. Respiratory complications are often an indication of the severity of spinal cord injury. About one-third of those with injury to the neck area will need help with breathing and require respiratory support via intubation, which involves inserting a tube connected to a machine that pushes oxygen into the lungs and removes carbon dioxide) through the nose or throat and into the airway. This may be temporary or permanent depending upon the severity and location of injury. Any injury to the spinal cord between the C1-C4 segments, which supply the phrenic nerves leading to the diaphragm, can stop breathing.
The phrenic nerves originate from C3, C4, and C5 and supply the diaphragm and cause the diaphragm to move and the lungs to expand. Complete severing of the spinal cord above the origin of the phrenic nerves (C3, C4,and C5) causes respiratory arrest. In injuries to the phrenic nerves, breathing stops because the phrenic nerves no longer send nerve impulses to the diaphragm. People with these injuries need immediate ventilatory support. People with high cervical cord injury may have trouble coughing and clearing secretions from their lungs. Special training regarding breathing and swallowing may be needed.
Figure 15. Nerves from the cervical spines (Cervical Plexus) including the phrenic nerve
Pneumonia. Respiratory complications are the leading cause of death in people with spinal cord injury, commonly as a result of pneumonia. Intubation increases the risk of developing ventilator-associated pneumonia; individuals with spinal cord injury who are intubated have to be carefully monitored and treated with antibiotics if symptoms of pneumonia appear. Attention to clearing secretions and preventing aspiration of mouth contents into the lungs can prevent pneumonia.
Circulatory problems. Spinal cord injuries can cause a variety of changes in circulation, including blood pressure instability, abnormal heart rhythms (arrhythmias) that may appear days after the injury, and blood clots. Because the brain’s control of the cardiac nerves is cut off, the heart can beat at a dangerously slow pace, or it can pound rapidly and irregularly. Arrhythmias are more common and severe in the most serious injuries. Low blood pressure also often occurs due to changes in nervous system control of blood vessels, which then widen, causing blood to pool in the small arteries far away from the heart. Blood pressure needs to be closely monitored to keep blood and oxygen flowing through the spinal cord tissue, with the understanding that baseline blood pressure can be significantly lower than usual in people living with spinal cord injuries. Since muscle movement contributes to moving blood back to the heart, people with spinal cord injuries are at triple the usual risk for blood clots due to stagnation of blood flow in the large veins in the legs. Treatment includes anticoagulant drugs and compression stockings to increase blood flow in the lower legs and feet.
Spasticity and muscle tone. When the spinal cord is damaged, information from the brain can no longer regulate reflex activity. Reflexes may become exaggerated over time, causing muscle spasticity. Muscles may waste away or diminish due to underuse. If spasms become severe enough, they may require medical treatment. For some, spasms can be as much of a help as they are a hindrance, since spasms can tone muscles that would otherwise waste away. Some people can even learn to use the increased tone in their legs to help them turn over in bed, propel them into and out of a wheelchair, or stand.
Autonomic dysreflexia. The autonomic nervous system controls involuntary actions such as blood pressure, heartbeat, and bladder and bowel function. Autonomic dysreflexia is a life-threatening reflex action that primarily affects those with injuries to the neck or upper back. It happens when there is an irritation, pain, or stimulus to the nervous system below the level of injury. The irritated area tries to send a sensory signal to the brain, but the signal may be misdirected, causing a runaway reflex action in the spinal cord that has been disconnected from the brain’s regulation. Unlike spasms that affect muscles, autonomic dysreflexia affects blood vessels and organ systems controlled by the sympathetic nervous system. Anything that causes pain or irritation can set off autonomic dysreflexia, including a full bladder, constipation, cuts, burns, bruises, sunburn, pressure of any kind on the body, or tight clothing. Symptoms of its onset may include flushing or sweating, a pounding headache, anxiety, sudden increase in blood pressure, vision changes, or goose bumps on the arms and legs. Emptying the bladder or bowels and removing or loosening tight clothing are just a few of the possibilities that should be tried to relieve whatever is causing the irritation. If possible, the person should be kept in a sitting position, rather than lying flat, to keep blood flowing to the lower extremities and help reduce blood pressure.
Pressure sores (or pressure ulcers). Pressure sores are areas of skin tissue that have broken down because of continuous pressure on the skin and reduced blood flow to the area. People with paraplegia and tetraplegia are susceptible to pressure sores because they may lose all or part of skin sensations and cannot shift their weight. As a result, individuals must be shifted periodically by a caregiver if they cannot shift positions themselves. Good nutrition and hygiene can also help prevent pressure sores by encouraging healthy skin. Special motorized rotating beds may be used to prevent and treat sores.
Pain. Some people who have spinal cord nerve are paralyzed often develop neurogenic pain—pain or an intense burning or stinging sensation may be unremitting due to hypersensitivity in some parts of the body. It can either be spontaneous or triggered by a variety of factors and can occur even in parts of the body that have lost normal sensation. Almost all people with spinal cord injury are prone to normal musculoskeletal pain as well, such as shoulder pain due to overuse of the shoulder joint from using a wheelchair. Treatments for chronic pain include medications, acupuncture, spinal or brain electrical stimulation, and surgery. However, none of these treatments are completely effective at relieving neurogenic pain.
Bladder and bowel problems. Most spinal cord injuries affect bladder and bowel functions because the nerves that control the involved organs originate in the segments near the lower end of the spinal cord and lose normal brain input. Although the kidneys continue to produce urine, bladder control may be lost and the risk of bladder and urinary tract infections increases. Some people may need to use a catheter to empty their bladders. The digestive system may be unaffected, but people recovering from a spinal cord injury may need to learn ways to empty their bowels. A change in diet may be needed to help with control.
Sexual function. Depending on the level of injury and recovery from the trauma, sexual function and fertility may be affected. A urologist and other specialists can suggest different options for sexual functioning and health.
Depression. Many people living with a spinal cord injury may develop depression as a result of lifestyle changes. Therapy and medicines may help treat depression.
Once someone has survived the injury and begins to cope psychologically and emotionally, the next concern is how to live with disabilities. Doctors are now able to predict with reasonable accuracy the likely long-term outcome of spinal cord injuries. This helps people experiencing SCI set achievable goals for themselves, and gives families and loved ones a realistic set of expectations for the future.
Spinal cord injury treatment
Injury to the spine isn’t always obvious. Any injury that involves the head and neck, pelvic fractures, penetrating injuries in the area of the spine, or injuries that result from falling from heights should raise concerns regarding an unstable spinal column. Until imaging of the spine is done at an emergency or trauma center, people who might have spine injury should be cared for as if any significant movement of the neck or back could cause further damage.
At the accident scene, emergency personnel will immobilize the head and neck to prevent movement, put a rigid collar around the neck, and carefully place the person on a rigid backboard to prevent further damage to the spinal cord. Sedation may be given to relax the person and prevent movement. A breathing tube may be inserted if the injury is to the high cervical cord and the individual is at risk of respiratory arrest.
At the hospital or trauma center, realigning the spine using a rigid brace or axial traction (using a mechanical force to stretch the spine and relieve pressure on the spinal cord) is usually done as soon as possible to stabilize the spine and prevent additional damage. Fractured vertebrae, bone fragments, herniated discs, or other objects compressing the spinal column may need to be surgically removed. Spinal decompression surgery to relieve pressure within the spinal column also may be necessary in the days after injury. Results of a neurosurgical study show that, in some cases, earlier surgery is associated with better functional recovery.
Emergency actions
Urgent medical attention is critical to minimize the effects of any head or neck trauma. That’s why treatment for a spinal cord injury often begins at the scene of the accident.
Emergency personnel typically immobilize the spine as gently and quickly as possible using a rigid neck collar and a rigid carrying board, which they’ll use to transport you to the hospital.
Early (acute) stages of treatment
In the emergency room, doctors focus on:
- Maintaining your ability to breathe
- Preventing shock
- Immobilizing your neck to prevent further spinal cord damage
- Avoiding possible complications, such as stool or urine retention, respiratory or cardiovascular difficulty and formation of deep vein blood clots in the extremities
You may be sedated so that you don’t move and sustain more damage while undergoing diagnostic tests for spinal cord injury.
If you do have a spinal cord injury, you’ll usually be admitted to the intensive care unit for treatment. You may even be transferred to a regional spine injury center that has a team of neurosurgeons, orthopedic surgeons, spinal cord medicine specialists, psychologists, nurses, therapists and social workers with expertise in spinal cord injury.
Medications. Intravenous (IV) methylprednisolone (A-Methapred, Solu-Medrol) is a treatment option for an acute spinal cord injury. If methylprednisolone is given within eight hours of injury, some people experience mild improvement.
It appears to work by reducing damage to nerve cells and decreasing inflammation near the site of injury. However, it’s not a cure for a spinal cord injury.
Immobilization. You may need traction to stabilize your spine, to bring the spine into proper alignment or both. In some cases, a rigid neck collar may work. A special bed also may help immobilize your body.
Surgery. Often surgery is necessary to remove fragments of bones, foreign objects, herniated disks or fractured vertebrae that appear to be compressing the spine. Surgery may also be needed to stabilize the spine to prevent future pain or deformity.
Experimental treatments. Scientists are trying to figure out ways to stop cell death, control inflammation and promote nerve regeneration. Ask your doctor about the availability of such treatments.
How does rehabilitation help people recover from spinal cord injuries ?
No two people will experience the same emotions after surviving a spinal cord injury, but almost everyone will feel frightened, anxious, or confused about what has happened. It’s common for people to have very mixed feelings: relief that they are still alive, but disbelief at the nature of their disabilities.
Rehabilitation programs combine physical therapies with skill-building activities and counseling to provide social and emotional support. The education and active involvement of the newly injured person and his or her family and friends is crucial.
A rehabilitation team is usually led by a doctor specializing in physical medicine and rehabilitation (called a physiatrist), and often includes social workers, physical and occupational therapists, recreational therapists, rehabilitation nurses, rehabilitation psychologists, vocational counselors, nutritionists, a case worker, and other specialists.
In the initial phase of rehabilitation, therapists emphasize regaining communication skills and leg and arm strength. For some individuals, mobility will only be possible with the assistance of devices such as a walker, leg braces, or a wheelchair. Communication skills such as writing, typing, and using the telephone may also require adaptive devices for some people with tetraplegia.
Physical therapy includes exercise programs geared toward muscle strengthening. Occupational therapy helps redevelop fine motor skills, particularly those needed to perform activities of daily living such as getting in and out of a bed, self-grooming, and eating. Bladder and bowel management programs teach basic toileting routines. People acquire coping strategies for recurring episodes of spasticity, autonomic dysreflexia, and neurogenic pain.
Vocational rehabilitation includes identifying the person’s basic work skills and physical and cognitive capabilities to determine the likelihood for employment; identifying potential work places and any assistive equipment that will be needed; and arranging for a user-friendly workplace. If necessary, educational training is provided to develop skills for a new line of work that may be less dependent upon physical abilities and more dependent upon computer or communication skills. Individuals with disabilities that prevent them from returning to the workforce are encouraged to maintain productivity by participating in activities that provide a sense of satisfaction and self-esteem, such as educational classes, hobbies, memberships in special interest groups, and participation in family and community events.
Recreation therapy encourages people with spinal cord injury to participate in recreational sports or activities at their level of mobility, as well as achieve a more balanced and normal lifestyle that provides opportunities for socialization and self-expression.
Adaptive devices also may help people with spinal cord injury to regain independence and improve mobility and quality of life. Such devices may include a wheelchair, electronic stimulators, assisted gait training, neural prostheses, computer adaptations, and other computer-assisted technology.
What research is being done ?
Scientists continue to investigate new ways to better understand and treat spinal cord injuries.
Many hospitals have developed specialized centers for spinal cord injury care. Many of these bring together spinal cord injury researchers from a variety of disciplines for partnerships regarding basic and clinical research, clinical care, and knowledge translation.
Current research is focused on advancing our understanding of four key principles of spinal cord repair:
- Neuroprotection—protecting surviving nerve cells from further damage
- Regeneration—stimulating the regrowth of axons and targeting their connections appropriately
- Cell replacement—replacing damaged nerve or glial cells
- Retraining CNS circuits and plasticity to restore body functions
A spinal cord injury is complex. Repairing it has to take into account all of the different kinds of damage that occur during and after the injury. Because the molecular and cellular environment of the spinal cord is constantly changing from the moment of injury until several weeks or even months later, combination therapies will have to be designed to address specific types of damage at different stages of the injury.
Neuroprotection
Strategies involving neuroprotection are aimed at preventing cell death, limiting or reducing inflammation, and stopping over-excitability of certain cells and their functions. Investigators are looking at ways to reduce inflammation within or near the injured spinal cord, which can restrict blood flow, affect nerve signal transmission, and increase cell death. One approach is using steroid drugs to reduce nerve cell damage and suppress activities of immune cells. One clinical trial identified slight improvement in motor function among some individuals who were given a steroid within 8 hours after injury. However, other trials showed the drug’s modest benefit did not outweigh serious side effects. Steroid therapy has not been approved by the U.S. Food and Drug Administration (FDA) for the treatment of acute spinal cord injury.
Antibiotics, which can cross the protective blood-brain barrier, have been shown to improve motor function, restoration, decrease lesion size, and reduce cell death in animal models of spinal cord injury.
The kidney hormone erythropoietin promotes the growth of new red blood cells and increases oxygen levels in the blood. Studies in animal models have shown that erythropoietin can reduce inflammation in the brain, improve blood flow to the brain, and reduce nerve cell death following brain injury. It also aids in the recovery of motor function. However, other trials in animal models show conflicting results regarding the drug’s usefulness in preventing inflammation and cell death. Researchers continue to study the drug in preclinical models.
Therapeutic hypothermia (controlled lowering of the body’s core temperature) can protect cells from damage following cardiac arrest, stroke, and traumatic brain injury. Therapeutic hypothermia has been shown to reduce the swelling and inflammation that presses on the spinal cord following injury in animal models and in small, limited human studies. It also can reduce damage to susceptible neurons following the primary injury, reduce damage to spinal cord microvasculature, and improve functional outcome. Researchers are studying the safety and effectiveness of different durations of hypothermia following spinal cord injury.
Researchers are trying to manipulate macrophages—a type of white blood cell that travels to the injury site during the inflammatory response—to promote nerve cell growth without causing further tissue damage. Following a spinal cord injury, macrophages at the site of injury begin to remove cellular debris and receive signals that help them promote the growth of axons. But, within a few days post-injury, the collection of macrophages increases inflammation, scarring, and toxicity, which can worsen the damage. Scientists hope to learn how to signal macrophages to continue their restorative function while turning off their damaging consequences.
The buildup of sodium and glutamate in cells following a spinal cord injury can lead to cell damage and impaired or blocked cell signaling. The drug riluzole, which slows the progression of the disease amyotrophic lateral sclerosis, has shown in animal models to improve motor function and reduce cell death loss caused by decreased blood flow following spinal cord injury. The experimental drug HP 184 blocks the entry of sodium into cells (which can impair nerve function) and may enhance cell signaling in surviving axons that have had their protective myelin cover either destroyed or damaged following spinal cord injury. Other scientists are examining drugs that target glutamate, whose release is greatly increased following a spinal injury. Excess glutamate leads to cell death and blocked transmission of signals across nerve synapses. Researchers are studying different drugs that may reduce glutamate binding among cells following spinal cord injury, which could reduce secondary cell death, improve motor function outcome, and reduce long-term hypersensitive pain post-injury.
Regeneration
Neurons have a limited capacity to regenerate. As nerve cells are either damaged or destroyed by injury, and as others die naturally during development, the number of chemical interactions between adjacent nerve cells (synapses)decreases. Nerve cells can die when they do not make enough synapses, leaving large numbers of supporting glial cells in the area of damage. Glial cells are thought to support tissue after injury to the spinal cord but also inhibit the growth of axons.
Approaches to repairing damaged axons through remyelination and new growth include:
Some anti-inflammatory drugs have been shown to encourage axonal regeneration by stimulating CNS nerve axons to grow and by inhibiting amino acid toxicity and cell death that occurs after the initial injury. Two such nonsteroidal anti-inflammatory drugs are ibuprofen and indomethacin. The drug rolipram was shown to encourage axonal regeneration in an animal model of spinal cord injury. Preclinical studies are examining the effectiveness of rolipram in combination with other drugs given at different delivery times post-injury.
Antibodies are proteins made by immune cells and are designed to attach themselves to specific foreign proteins (called antigens) and disable them. Therapeutically, antibodies can be made that target specific proteins that inhibit repair of the body after injury. Monoclonal antibodies, produced in a laboratory, may promote nerve fiber regeneration by blocking proteins in the myelin debris that inhibit regeneration after spinal cord injury. Scientists are testing monoclonal antibodies at the site of the spinal cord injury in animal models in an attempt to block anti-regeneration activity of cells in the damaged central nervous system and improve nerve function and recovery. For example, researchers are using a Nogo-A monoclonal antibody to block proteins that inhibit the sprouting and regeneration of axons following a spinal injury. Other antibodies, called anti-MAG (myelin associated glycoproteins), are designed to counteract protein-sugar molecules on myelin-forming debris at the site of injury.
A number of other targets are being tested to overcome inhibition to regeneration. Cethrin, a recombinant protein that blocks activation of rho (a protein that inhibits axon regeneration), has been tested clinically. A drug that targets Nogo-receptors is being developed to promote regeneration after spinal cord injury and stroke. Recent animal studies showing that inhibition of the tumor-suppressing gene PTEN can promote growth of upper motor neurons has led to active research into strategies to promote regeneration after spinal cord and optic nerve injuries. Finally, the histone deacetylases (HDAC) are a class of compounds that are involved in the regulation of gene expression and negatively affect cell structure following a traumatic spinal cord injury. Preclinical studies are examining ways to inhibit HDAC activity as a way to protect and allow axons to overcome inhibition in regions of a spinal injury.
To get past the glial scar that forms after a spinal cord injury and be able to transmit signals from the cell body, an axon has to advance between the tangles of long, branching molecules made up of inhibitory proteins and sugars that surround the cells. Experiments have successfully used a bacterial enzyme, chondroitinase ABC, to clear away the tangles so that axons could grow in animal models of injury. Researchers are looking at ways to combine chondroitinase ABC with other treatments, such as cell transplants, to increase functional recovery.
Other researchers are using a tissue-engineered highway-like matrix that is implanted onto the spinal cord to help axons “bridge” the lesion that forms at the injury site and to rebuild neural circuits. They also will look at using the matrix as a way of delivering growth factors that can promote nerve cell survival and inhibit proteins associated with the glial scar.
Cell Replacement
Controversy exists over potential benefits and possible harmful consequences of cell replacement and cell transplants. The potential of several cell types, including stem cells and glial cells, to treat spinal cord injury is being investigated eagerly, but there are many things about stem cells that researchers still need to understand. For example, researchers know there are many different kinds of chemical signals that tell a stem cell what to do. Some of these are internal to the stem cell, but many others are external—within the cellular environment—and will have to be recreated in the transplant region to encourage proper growth and differentiation. Because of the complexities involved in stem cell treatment, researchers expect these kinds of therapies to be possible only after much more research is done. Preclinical research results are limited but show cell transplants can regenerate neuronal growth and create new connections between neurons.
Scientists are experimenting with a variety of cells for effectiveness and safety in increasing connectivity and restoring function following a spinal cord injury:
Human oligodendrocyte progenitor cells have been shown to reduce secondary damage following a spinal cord injury and promote functional recovery and remyelination. Researchers are expanding trials to better determine optimal cell delivery windows and evaluate potential risks of transplants, such as the formation of tumors and inflammatory reactions.
Schwann cells surround and insulate peripheral nerves, and often grow into the spinal cord after injury. Schwann cells that have been transplanted at the site of the spinal cord injury can produce growth factors and reinsulate damaged nerve axons. They are not stem cells in that their fate as Schwann cells is determined before transplantation. Schwann cells can be taken from the individual’s own body, which reduces the need for immune-suppressing drugs and the risk of tumor formation, but this requires several weeks after injury before grafting can be done. Preclinical studies are examining their potential in safely treating both acute and chronic spinal cord injury.
Bone marrow stromal cells, taken from the tissue found inside bones, have been shown in some studies to increase recovery from an injury to the spinal cord. Scientists are studying the injection of these cells into the cerebrospinal fluid (fluid that bathes the brain and spinal cord) to promote proteins needed to grow and maintain nerves and increase nerve signaling across the glial scarring that forms post-injury.
Nasal olfactory ensheathing cells, taken from the lining of the nose, are special types of glial cells that have been shown to promote axon regeneration and remyelination at the injury site. The transplanted cells have been shown to permit regrowth of axons in both the peripheral and central nervous systems, and improve functional outcome in animal models of spinal cord injury. Early-stage trials in humans are being conducted overseas.
Retraining CNS circuits and plasticity
Recovery from a spinal cord injury may occur for quite some time after the initial injury, as part of the brain’s ability to reorganize or form new nerve connections and pathways following injury or cell death (called neuroplasticity).
Active rehabilitation and exercise
Specific training can improve function, coordination of fine muscle movements, and overall strength and health. Scientists are comparing the relative effectiveness of an intensive task-specific motor training program added to standard rehabilitation compared with standard rehabilitation alone for improving hand function and clinical outcomes in people with recent tetraplegia.
Much as in the general population, cardiovascular disease is a leading cause of death in persons with spinal cord injury. After the injury, the opportunity to actively exercise large muscles affected by paralysis is limited or may require assistive technologies. Researchers have recently shown that such injuries increase the risk of cardiovascular disease, along with other muscle atrophy and effects of deconditioning. Since cardiovascular disease occurs earlier in life for those with a spinal cord injury, researchers are assessing the impact of acute exercise, conditioning, and dietary supplementation on measures of fitness, function, and cardiovascular disease risk factors/modifiers in individuals with tetraplegia. Other scientists are examining an add-on group exercise program to the usual physical therapy delivered in the hospital to see if it improves the participant’s overall outlook on exercise and recovery, and to promote physical activity as part of a healthy lifestyle.
Epidural Stimulation
In May 2011, scientists funded in part by the National Institutes of Health reported that after intensive physical therapy and electrical stimulation to the spine, a man with a paralyzing spinal cord injury from the chest down had recovered the ability to stand and move paralyzed muscles at will when the stimulator is active. The man participated in a pilot study that combined epidermal stimulation and locomotor training—involving his being suspended for hours a day in a harness, walking on a treadmill while physical therapists moved his legs in stepping motions. Two years of locomotor training following his injury did not improve his ability to walk or stand. But he did improve after December 2009, when electrodes were surgically implanted over the paralyzed area of his spinal cord and began sending rhythmic electrical bursts to neurons in the spinal cord during the locomotor training sessions. This epidural simulation imitates the brain’s sending signals to the spinal cord to begin movement. He gradually became able to stand and fully bear his own weight for a few minutes at a time. Although he can’t walk without assistance, he can bend one leg at the knee and flex his ankle when the stimulator is active. Locomotor training without any epidural stimulation is routinely used as a rehabilitative technique for people with spinal cord injuries—which allows some individuals to retain the ability to move and feel below the injury. Meanwhile, a form of epidural stimulation is used to relieve pain for some individuals. The researchers and NIH scientists caution that this finding was in only one person, and further study is required to confirm these early, promising results and to understand exactly how the stimulation is working.
Functional Electric Stimulation
Exciting and promising results in restoring or assisting function in individuals with chronic spinal cord injury have been shown in studies using functional electric stimulation (FES). FES devices use a computer system and electrodes to deliver small bursts of a low-level electrical current to paralyzed muscles, to generate muscle contractions. Researchers are working to improve the electrode and computer interfaces so they can produce more natural yet complex movements. FES is being used to restore breathing without a ventilator, cough unassisted, enhance bladder and bowel control, increase hand movement and grasping, and improve blood flow to the skin.
Several studies continue to investigate the use of FES in restoring or improving function following a spinal cord injury. Researchers are using FES to help individuals with tetraplegia move paralyzed muscles to restore voluntary grasping function. Others are attempting to stimulate recovery with the goal of being able to grasp objects without stimulation once the treatment program is completed. This approach has been shown to be useful for people who have had a stroke.
One study is using a surgically implanted FES to facilitate exercise, standing, stepping, and/or balance in people with various degrees of paralysis. A related study is using a surgically implanted FES to facilitate stability of the trunk and hips, and to evaluate how stabilizing and stiffening the trunk can change the way spinal cord injured volunteers sit, breathe, reach, push a wheelchair, or roll in bed.
Transcranial Direct Current Stimulation (tDCS) is another form of electric stimulation that is being tested experimentally to improve patient outcomes after a spinal cord injury. tDCS is a noninvasive procedure that delivers continuous low electrical current to areas of the brain involved with movement via small electrodes placed on the scalp. Researchers are investigating if combining tDCS with exercise therapy for the affected hand will increase performance over exercise therapy alone. Other researchers hope to determine if tDCS can decrease chronic pain in people with spinal cord injury.
Robotic-assisted therapy
Most recovery following SCI takes place within six months after injury. Substantial recovery after 12 months is unusual, but researchers continue to test ways to restore function in persons with chronic paralysis. In one very small study involving patients one year after injury, scientists are testing the safety and efficacy of a type of robotic therapy device known as the AMES device. The aim of this study is to investigate the use of assisted movement and enhanced sensation (AMES) technology in the rehabilitation of the legs of participants with incomplete spinal cord injury. The AMES device rotates the ankle over a range of 30 degrees while vibrators stimulate the tendons attached to muscles that move the leg. The subject’s task is to assist the motion of the device.
In another study, researchers will determine the effect of using body-weight supported treadmill training and a robotic gait trainer on functional movement from place to place (ambulation) in people with an incomplete spinal cord injury. The person is suspended in a harness and onto a treadmill, and the robotic legs are strapped to the person. A computer controls the pace of the walking. The effect of the therapy will be evaluated by analyzing changes in ambulation and gait patterns during walking.
Brain-computer interfaces (BCI)
The goal of brain-computer interface technology is to bypass the damaged nerve circuits in the spinal cord and establish a direct link between the brain and an assistive implanted device which may restore an individual’s control of voluntary muscle movement and coordination of paralyzed muscles.
Most individuals with tetraplegia have intact brain function but are unable to move due to injury or disease affecting the spinal cord. Brain-computer interface technology is based on the finding that with intact brain function, neural signals for movement are generated in the brain and can be used to control computer-assisted devices. By implanting electrodes in the brain, individuals can be trained to practice thoughts and thereby generate neural signals that are interpreted by a computer and translated to movement which can then be used to control a variety of devices or computer displays.
Researchers are working to develop BCI technology to offer individuals with upper limb paralysis a natural and rich control signal for prosthetic arms or FES device to re-animate paralyzed arms. The study uses electrocorticographic (ECoG) electrodes that interface with neurons in the brain’s cortex (the part of the brain that is responsible for higher thought and motor control) to measure brain activity. Participants will learn to control computer cursors, virtual reality environments, and assistive devices such as hand orthotics and FES devices using neural activity recorded with the ECoG sensor.
Another preliminary study is assessing the safety and effectiveness of a brain-computer interface to give people with tetraplegia the ability to control a computer cursor and other assistive devices with their thoughts. A small electrode array is inserted into the part of the brain’s surface that controls movements. Using the very precise signals this device records, researchers are working to improve the computer’s ability to interpret the person’s intent and transfer that to the display screen.
- Spinal cord injury. Mayo Clinic. http://www.mayoclinic.org/diseases-conditions/spinal-cord-injury/basics/definition/CON-20023837p=1?p=1[↩]
- Spinal Cord Injury: Hope Through Research. National Institute of Neurological Disorders and Stroke. https://www.ninds.nih.gov/Disorders/Patient-Caregiver-Education/Hope-Through-Research/Spinal-Cord-Injury-Hope-Through-Research[↩][↩][↩][↩][↩][↩][↩][↩][↩]
- International Standards for Neurological Classification of Spinal Cord Injury. American Spinal Injury Association. http://asia-spinalinjury.org/wp-content/uploads/2016/02/International_Stds_Diagram_Worksheet.pdf[↩][↩]