Contents
What is glycine ?
Glycine is a non-essential amino acid, with just an amino group, a carboxyl group and two hydrogen atoms all bound to one carbon atom (Figure 1) 1. Glycine molecular weight is 75.067 g/mol 1. Glycine is found primarily in gelatin and silk fibroin and used therapeutically as a nutrient. Glycine is also a fast inhibitory neurotransmitter. Glycine has 4 well known roles within the human body and brain.
- Role in metabolism 2. Glycine is known to attenuate the increase in fatty acids in rats fed on a high sucrose diet 3.
- Inhibitory neurotransmitter in the adult central nervous system (preferentially in the brainstem and spinal cord) 4.
- Cytoprotective agent in against ischaemia–reperfusion injury 5. Glycine has anti-inflammatory effects during ischemia, injury, and transplantation 6.
- Glycine subjectively and objectively improved sleep quality in humans who suffered from repeated sleep complaints 7, 8.
Figure 1. Glycine molecule
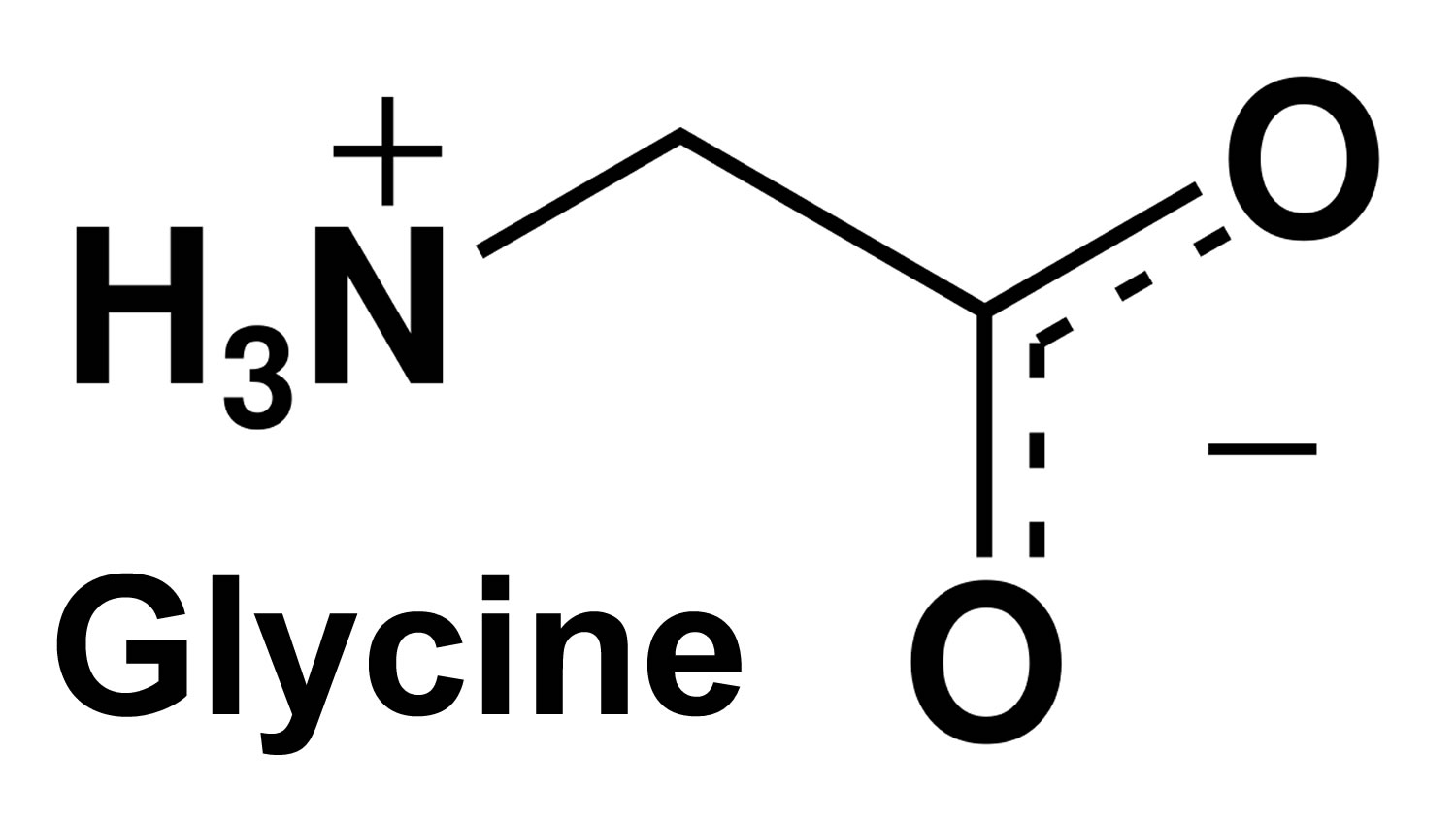
Glycine as an Inhibitory Neurotransmitter
It is approximately 40 years since Aprison & Werman in 1965 9 noted that the concentration of glycine in the spinal cord tissue is far higher than elsewhere in the brain and because of this, it may have a role as a neurotransmitter. The levels of glycine were highest in the ventral horn, the location for spinal interneuronal terminals and the destruction of interneurones by anoxia correlated with a fall in tissue glycine levels, suggesting that the interneurones may be the repository of stored glycine. To add to the growing evidence of glycine as a neurotransmitter molecule, Shank & Aprison in 1970 10 demonstrated that glycine could be synthesized by neurones and later on Hopkin & Neal also in the 1970 11 were the first to demonstrate the release of this amino acid after applying stimulation. Once released, and having dissociated from the postsynaptic glycine receptors, glycine was sequestered and taken back into cells by Na+-dependent high-affinity transporters (usually referred to as uptake systems or carriers at that time).
Glycine is now readily accepted as a vital spinal cord and brainstem inhibitory neurotransmitter 2. Glycine is an important component and precursor for many macromolecules in the cells. Binding of glycine to the glycine receptor (a ligand-gated chloride channel) triggers chloride influx, membrane hyperpolarization, and thereby creating an inhibitory post-synaptic potential (Figure 2). In contrast, glycine also acts as a co-agonist, along with glutamate, facilitating an excitatory potential at the glutaminergic N-methyl-D-aspartic acid (NMDA) receptors. That way glycine is, for example, involved in the generation of reflex responses, in the processing of sensorial inputs and in the sensation of pain. The alkaloid strychnine is a potent competitive inhibitor of the glycine receptor acting already at micromolar concentrations. Structurally, the neuronal glycine receptor is a pentamer presumably being composed of two α and three β subunits. There are four different α subunits (α1 to α4) but only one β subunit, encoded by separate genes. The scaffolding protein gephyrin binds to the β subunits and connects the glycine receptor with filaments of the submembraneous cytoskeleton, mediating, among others, clustering of the receptor. In addition to its function as an inhibitory neurotransmitter, glycine facilitates neurotransmission mediated by glutamate, the major excitatory neurotransmitter in the CNS. The NMDA (glutamate) receptor does not only have a specific recognition site for glutamate but also a second one for glycine 12. To activate the receptor, both recognition sites need to be occupied. The specific binding site for glycine within the NMDA receptor cannot be blocked by strychnine 13, and an impaired function of NMDA receptors has been proposed to contribute to psychiatric disorders such as schizophrenia 14.
Glycine receptors and disease
Despite the lack of any useful therapeutic ligands for the glycine receptor, there is a considerable amount of evidence suggesting that mutations in the glycine receptor play pivotal roles in a number of diseases concerning motor control (see Figure 3. Glycine receptor). These disorders are often characterized by intense muscle contraction/tone (hypertonia) and normally associated with an exaggerated startle reflex, which leads to the hypertonia. Three naturally occurring mutations in the glycine receptors give rise to the phenotypes known as spastic, spasmodic and oscillator 15, 16. The spastic mice seemingly lack a sufficient number of cell surface functional glycine receptors due to disruption in the production of β subunit mRNA. By contrast, the spasmodic mice demonstrate a phenotype that is characterized by a startle reflex to sudden acoustic stimuli. A single mutation (A52S) is sufficient to cause this effect and effectively lowers the potency of glycine at these receptors, making them less efficient as inhibitory receptors. The third mutation, known as oscillator, exhibits rapid, repetitive shaking, eventually leading to a form of rigor. This phenotype has been associated with the drastic loss of adult glycine receptors following the deletion of the large intracellular domain in α1 subunits between TM3 and TM4 and also the loss of TM4 itself.
With regard to humans, the rare but potentially fatal disorder of hyperekplexia or human startle disease also involves the mutation of glycine receptors 17. Essentially, the disorder presents as a severe muscle rigidity which is initiated by abrupt stimuli that can take various forms, including light, sound or physical contact. The muscle contraction that results impedes ambulation and can also lead to postural instability. Molecular genetics studies have enabled many mutations in the α1 subunit TM1–TM2 linker, TM2 itself and the TM2–TM3 linker to be implicated as the underlying causes of hyperekplexia 17. Curiously, no hyperekplexia mutations have been detected in human α2 or α3 subunits to date. The mutations have various effects that range from reducing the sensitivity of the receptor to glycine, to reducing the single-channel current or the number of functional receptors in the cell membrane. Sometimes single mutations may cause no phenotype, but when they are combined in one individual they reduce receptor expression 16. A combined mutation in the β subunit has also been reported that reduces the α1β glycine receptor sensitivity to glycine, giving rise to a hyperekplexia phenotype 18. Generally, taking all these observations into consideration, the reduced sensitivity to glycine and the reduced numbers of glycine receptors all contribute to the main effect of decreasing the amplitude of glycine-activated currents. Therefore, the dysfunction of other proteins associated with the glycinergic synapse, which impact on the concentration of glycine in the synapse, could have consequences for the expression of hyperekplexia. It is therefore not surprising that mutations in gephyrin can cause hyperekplexia 19 and the ablation of the glycine transporter (GlyT2) also produces a hyperekplexia phenotype 20 because this transporter is required to sequester glycine for future release at inhibitory synapses.
Figure 2. Glycine an inhibitory neurotransmitter and an inhibitor of the activation of immune cells
Note: Glycine, an inhibitory neurotransmitter and an inhibitor of the activation of immune cells. Activation of GlyR by glycine results in influx of chloride ions, membrane hyperpolarization and thus, in neurons, in inhibition of the response to excitatory neurotransmitters. In immune cells, hyperpolarization inhibits opening of voltage-operated calcium channels (VOCs), thus influx of calcium ions and activation of these cells, resulting among others in attenuation of the formation of TNF-α, NO and ROS.
[Source 2]Figure 3. Glycine receptor
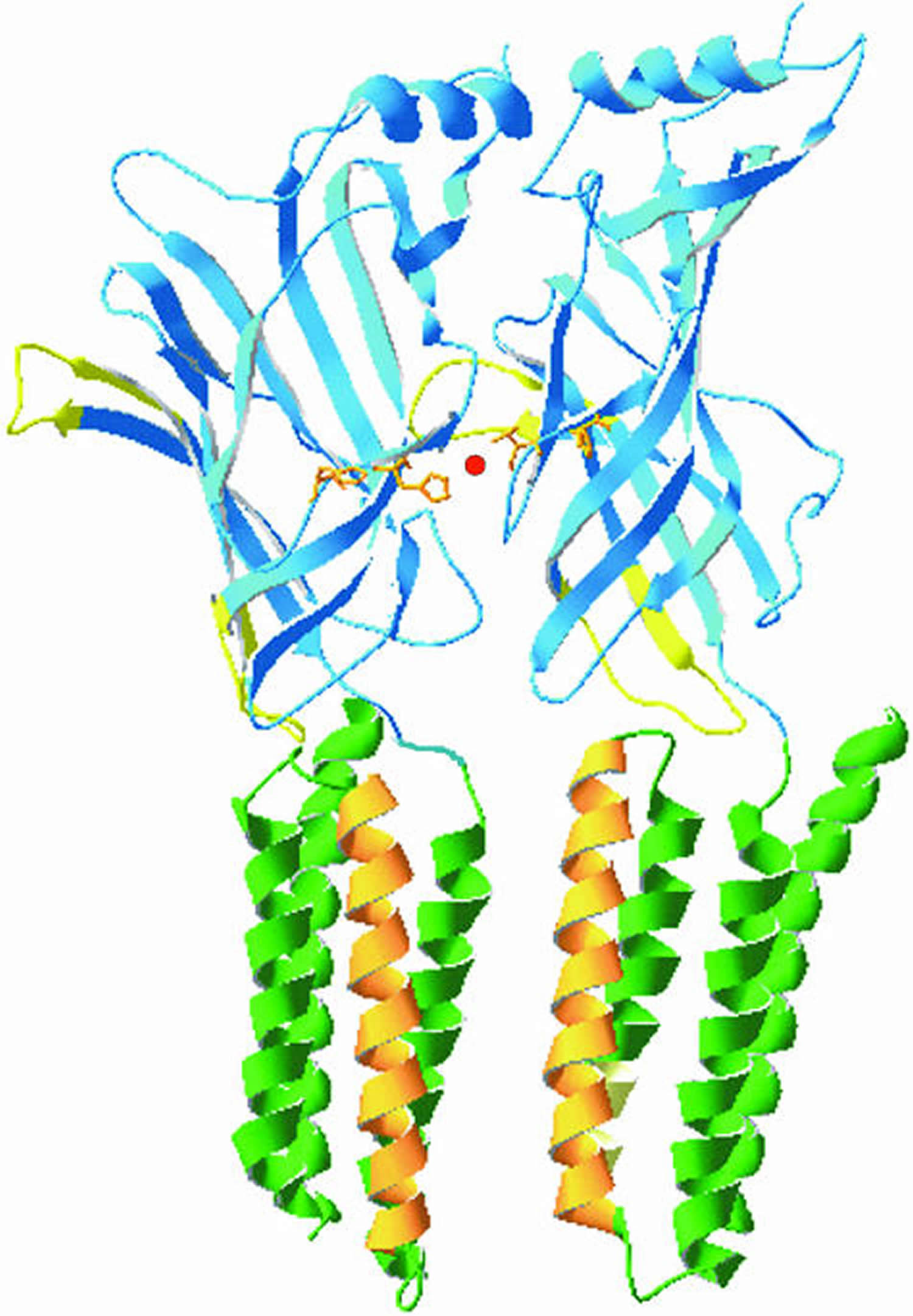
Note: The glycine receptor. This diagram shows two juxtaposed α1 subunits of the glycine receptor based on a homology model with the acetylcholine-binding protein and the TM domains taken from the nicotinic acetylcholine receptor. The N-terminal domains are shown in blue with the Cys loops depicted in yellow. The TM (transmembrane) domains TM1, 3 and 4 are shown in green and the ion channel lining TM2 is shown in orange. The model illustrates the position of histidines 107 and 109 (orange) and their ability to coordinate a Zn2+ ion (red) at the interface between two adjacent α1 subunits.
[Source 21]Figure 4. The glycinergic synapse
Note: Schematic representation of a typical glycinergic synapse. The glycine receptors are shown as pentamers of stoichiometry 3α : 2β and also the more recent preferred stoichiometry of 2α : 3β. The receptors are anchored via the β subunits to gephyrin and thus to the microfilaments and microtubules. Presynaptic glycine is packaged into vesicles via the vesicular inhibitory amino-acid transporter (VIAAT) before release. After dissociation from the receptor, either of two discretely localized glycine transporters (GlyT1 or 2) sequester the glycine, which can then be re-packaged into synaptic vesicles or hydrolysed via the glycine cleavage system (GCS). The diversity of glycinergic synapses is further demonstrated by the fact that some synapses can corelease both glycine and GABA from the same axon terminals, causing the activation of the appropriate glycine and GABAA receptors.
[Source 21]Glycine and sleep quality in humans who suffered from insomnia
Approximately 30% of the general population suffer from symptoms of insomnia 22. Insomnia-induced problems include cognitive inefficiency, sleepiness, mood disruptions 23, impaired attention, and memory deficits 24. Thus, treatment of insomnia is critical. To treat the symptoms, hypnotics are often used by those suffering from insomnia. Moreover, some natural herb extracts, such as chamomile 25 and valerian 26 have been used to cure insomnia.
In urban populations, most individuals experience restricted sleep. This is especially true for office workers in large cities, who are prone to restricted sleep periods during the week. During partial sleep restriction, daytime sleepiness and fatigue have been shown to increase, and daytime performance has been shown to be impaired 27, 28, 29; these parameters can also be indicative of sleep quality. Taken together, improving sleep quality during sleep-restricted periods would ameliorate daytime sleepiness and fatigue.
Recently, researchers found that glycine subjectively and objectively improved sleep quality in humans who suffered from repeated sleep complaints 7, 8. Glycine is a non-essential amino acid, and mammals can produce sufficient amounts of it via de novo synthesis. In humans, approximately 45 g of endogenous glycine is synthesized 30 and 3–5 g of glycine is ingested from the daily diet. In both the peripheral and central nervous system, glycine acts as an inhibitory neurotransmitter via glycine receptors 31. Conversely, this amino acid also acts as an excitatory co-agonist via the N-methyl-d-aspartate subtype of glutamate receptors (NMDA receptors) 32, 33.
To investigate the mechanisms by which glycine improves sleep, researchers assessed the permeability of the blood–brain barrier to glycine in a rat model 34. The results indicated that ingested glycine passively diffuses across the blood–brain barrier by non-specific transportation. Furthermore, glycine improves sleep in rats by decreasing core body temperature and increasing vasodilatation 35, 36. The suprachiasmatic nucleus is necessary for glycine-induced hypothermia and vasodilatation because ingested glycine primarily acts on the NMDA Receptors in the suprachiasmatic nucleus. This mechanism may result in an improvement in sleep quality. Although glycine has been shown to improve sleep quality in humans and rats 7, 8, 35, 36, the relationship between glycine ingestion and the circadian clock remains unknown. The relationship between the clock gene and sleep are well known. For example, Per2 mutation results in advanced sleep phase syndrome 37, 38 and Dec2 mutation causes short sleep phenotypes in humans 39. Melatonin is secreted by the pineal gland and circulates throughout the body. Melatonin concentrations are high during the dark period in all species, regardless of diurnal or nocturnal activity 40, 41. Therefore, the timing of melatonin secretion is associated with circadian rhythms. The suprachiasmatic nucleus, where many types of neuropeptides and clock genes are localized, is known as the circadian pacemaker site 42. Plasma melatonin concentration has been shown to be high during the nocturnal period, and ingestion of melatonin is known to induce sleep 40, 41.
This study 43 showed that the ingestion of 3 gram of glycine at bedtime occasionally improves the impairments in subjective alertness and neurobehavioral functions induced by acute and modest sleep restriction. The same researchers found, that the melatonin levels were not significantly changed by glycine administration in either the diurnal and nocturnal period, suggesting that melatonin is not related to glycine administration 43. In addition, they measured the mRNA expression of known clock genes and neuropeptides in the suprachiasmatic nucleus. Bmal1 and Per2 are the clock genes expressed in the suprachiasmatic nucleus 44. The expression of these genes was not significantly changed by glycine administration, suggesting that glycine does not primarily affect the circadian rhythms. The expression levels of arginine vasopressin (AVP) and vasoactive intestinal peptide (VIP) in the suprachiasmatic nucleus, however, were significantly increased during the light period. Glycine increased arginine vasopressin (AVP) and vasoactive intestinal peptide (VIP) expression 4.2- and 3.2-fold, respectively, during the light period. Thus, the researchers believe that glycine has significant effects on the expression levels of these neuropeptides during the light period, suggesting that glycine may modulate suprachiasmatic nucleus function and neurotransmission to the mPOA via the regulation of arginine vasopressin (AVP) and vasoactive intestinal peptide (VIP) expression 43. The suprachiasmatic nucleus has an afferent projection to the medial preoptic area 45, an area known to be involved in thermoregulation 46. In conclusion, although no alterations in the circadian clock itself were observed, the results indicated that glycine modulated suprachiasmatic nucleus function. Therefore, glycine modulates certain neuropeptides in the suprachiasmatic nucleus and this phenomenon may indirectly contribute to improving the occasional sleepiness and fatigue induced by sleep restriction.
Glycine role in metabolism
Glycine is a simple, nonessential amino acid, although experimental animals show reduced growth on low-glycine diets. The average adult ingests 3 to 5 grams of glycine daily 47. Glycine is involved in the body’s production of DNA, phospholipids and collagen, and in release of energy. Glycine levels are effectively measured in plasma in both normal patients and those with inborn errors of glycine metabolism – glycine encephalopathy, also known as nonketotic hyperglycinemia – is an autosomal recessive condition caused by deficient enzyme activity of the glycine cleavage enzyme system and as a consequence, accumulation of large quantities of glycine in all body tissues including the brain 48. At conception, each sibling of an affected individual has a 25% chance of being affected, a 50% chance of being an asymptomatic carrier, and a 25% chance of being unaffected and not a carrier 48. De novo pathogenic variants occur in approximately 1% of individuals with glycine encephalopathy; thus, carrier status in parents should be confirmed by molecular genetic testing rather than be assumed or inferred. Most individuals with glycine encephalopathy do not reproduce. Carrier testing for at-risk relatives and prenatal testing for pregnancies at increased risk are possible if the pathogenic variants in the family are known. The majority of glycine encephalopathy presents in the neonatal period (85% as the neonatal severe form and 15% as the neonatal attenuated form). Of those presenting in infancy, 50% have the infantile attenuated form and 50% have the infantile severe form. Overall, 20% of all children presenting as either neonates or infants have a less severe outcome, defined as developmental quotient greater than 20. A minority of patients have mild or atypical forms of glycine encephalopathy. The neonatal form manifests in the first hours to days of life with progressive lethargy, hypotonia, and myoclonic jerks leading to apnea and often death. Surviving infants have profound intellectual disability and intractable seizures. The infantile form is characterized by hypotonia, developmental delay, and seizures. The atypical forms range from milder disease, with onset from late infancy to adulthood, to rapidly progressing and severe disease with late onset. The glycine cleavage enzyme system comprises four proteins: P-, T-, H- and L-proteins. Mutations have been described in the GLDC, AMT and GCSH genes encoding the P-, T-, and H-proteins respectively. The glycine cleavage system catalyses the oxidative conversion of glycine into carbon dioxide and ammonia, with the remaining one-carbon unit transferred to folate as methylenetetrahydrofolate. It is the main catabolic pathway for glycine and it also contributes to one-carbon metabolism. Patients with a deficiency of this enzyme system have increased glycine in plasma, urine and cerebrospinal fluid with an increased cerebrospinal fluid: plasma glycine ratio. No effective treatment exists for severe glycine encephalopathy; preliminary evidence suggests that children with pathogenic variants associated with residual glycine cleavage system enzyme activity treated aggressively in the first two years of life with sodium benzoate to reduce plasma concentration of glycine and N-methyl D-aspartate (NMDA) receptor site antagonists have improved outcome compared to late-treated or untreated controls. Other: antiepileptic drugs and/or ketogenic diet for seizure control; gastrostomy tube for feeding problems; therapy for gastroesophageal reflux; physical therapy 48. Valproate an anti-epileptic drug is avoided, as it increases blood and cerebrospinal fluid glycine concentrations and may increase seizure frequency and even result in coma in attenuated glycine encephalopathy.
Glycine is taken up by cells via a variety of glycine transporters; typically by a secondary active transport coupled to hydrogen, sodium and/or chloride ion uptake 49. Due to its small size, glycine is sterically extremely adaptable and the preferred candidate for an interior position in proteins. Besides being a decisive building block in many proteins, glycine is also a component of the tripeptide glutathione and of the bile acid glycocholic acid (Figure 5. Metabolism of glycine. THF, tetrahydrofolate). In addition, it is an essential substrate for the synthesis of a variety of biomolecules such as creatine, porphyrins and purine nucleotides. Within cells, glycine is oxidatively (NAD+-dependently) degraded by the mitochondrial glycine cleavage system to CO2, NH4+, NADH and a methylene group, which is accepted by tetrahydrofolate, thus forming N5,N10-methylenetetrahydrofolate 50, 51. Via methylenetetrahydrofolate, glycine is decisively involved in the metabolism of 1-carbon units and by that in further synthetic pathways. Using methylenetetrahydrofolate, mitochondrial and cytosolic serine hydroxymethyl transferase catalyses the formation of serine from glycine 50. The reactions catalysed by the glycine cleavage system and by serine hydroxymethyl transferase are reversible and thus can also be used for the synthesis of glycine. Glycine is also formed from glyoxylate, a reaction catalysed by alanine : glyoxylate aminotransferase, an enzyme which is predominantly located in peroxisomes 52.
Figure 5. Metabolism of glycine. THF, tetrahydrofolate
[Source 2]Glycine as as a cytoprotective agent against ischemia–reperfusion injury
Since its first description as a cytoprotective agent in 1987 5, glycine has been shown to protect against ischaemia–reperfusion injury [including related injuries due to anoxia, hypoxia, reactive oxygen species, chemically induced energy depletion, and mere resupply of oxygen (reoxygenation)] in a great variety of experimental models. There are already profound reviews covering specific aspects of this protective action such as protection of the liver in human liver transplant experiments 53, 54 and mechanisms of cytoprotection and inhibition of inflammation 6, 13, 55. In addition to protection from ischaemia–reperfusion injury, substantial experimental evidence has been presented that glycine may also protect against other injurious processes such as liver fibrosis and alcoholic liver disease, gastric ulcer, cyclosporine A-induced nephrotoxicity and arthritis 6, 6. Even inhibition of tumour growth in rats and mice has been reported 56.
Figure 6. Cell protective effects of glycine following ischaemia–reperfusion injury
Note: Cell injury, inflammatory response and regeneration during or following ischaemia–reperfusion. Protective effects of glycine. At concentrations around 1 mM and above, glycine decreases hypoxic cell injury by direct cytoprotection and inhibits the inflammatory response. A direct cytoprotective effect of glycine on other forms of cell injury occurring during reperfusion, such as those mediated by ROS, remains a matter of debate. The mechanism of protection by glycine at low-dose treatment regimens is unknown. The same is true for the effect of glycine on regeneration of the tissue injured by ischaemia–reperfusion.
[Source 2]As compared with the large number of experimental studies, there have been only a few clinical trials performed with glycine to protect against ischaemia–reperfusion injury.
In 1999, Arora et al. reported that glycine rinse decreases injury of the transplanted liver 57. In their study with a total number of 50 patients, livers were cold-stored in UW solution. After completion of both vena cava anastomoses (i.e. immediately before reperfusion), the hepatic artery was flushed with 150 mL, and the portal vein with 350 mL of either an electrolyte solution or the same solution supplemented with 2 mM glycine. Glycine rinse decreased postoperative transaminase elevations and the occurrence of bile duct strictures, and none of the patients in the glycine group required retransplantation as compared with three patients in the no-glycine group.
Using a somewhat different approach, the effect of glycine on reperfusion injury of the liver following liver transplantation was also studied by Schemmer et al. 58. In their study, liver recipients were infused with glycine (5.6 g) 1 h prior to reperfusion of the transplanted organ and subsequently daily during the following week, resulting in a fivefold increase in the serum glycine concentration. In preliminary results from seven patients, serum transaminases were markedly lower compared with matched historic control patients. Based on these data, a prospective double-blinded multicenter clinical trial was started in 2005, using a comparable glycine treatment regimen but a daily glycine dose of 11 g 59. As a preliminary result, decreased transaminases have been reported 60. The final results, however, have not been published yet.
In a double-blinded, placebo-controlled trial, the efficacy of sublingual glycine treatment was studied in 200 patients with acute ischaemic stroke (0.5, 1.0 or 2.0 g·day−1 for 5 days). One and 2.0 g glycine per day significantly improved clinical (functional) outcome and tended to decrease the 30 day mortality (Gusev et al., 2000).
High-risk cardiac patients scheduled to undergo cardiac surgery with the use of extracorporal circulation were treated preoperatively with oral immune-enhancing nutrition supplement for at least 5 days; glycine (around 32 gram per day) was added to the liquid supplement to prevent ischaemia–reperfusion injury 61. Glycine inclusion failed to improve organ function and postoperative recovery or to decrease postoperative infectious morbidity.
In summary, glycine has a great potential to protect from ischaemia–reperfusion injury. This is clearly suggested by numerous studies with cells, isolated perfused or cold-stored organs and experimental animals. However, protection by glycine from ischaemia–reperfusion injury does not apply to all organs and under all conditions. For certain organs such as the liver and the small intestine, several reports demonstrating protection have been published, while for other organs, especially the heart, very limited information on protection by glycine is available. Protection of the kidney in vivo appears to be unlikely. The brain deserves special attention due to the fact that glycine acts as a neurotransmitter in the central nervous system.
Glycine may prevent ischaemia–reperfusion injury by direct cytoprotection, presumably by inhibition of the formation of plasma membrane pores and by inhibition of the inflammatory response. However, the relevance of both protective mechanisms in detail remains unclear. Direct cytoprotection is most likely responsible for and limited to the protection in cells and perfused/cold-stored organs in the absence of blood or plasma. In contrast, under in vivo conditions, inhibition of the inflammatory response is presumably involved in the protective action of glycine against ischaemia–reperfusion injury; this, however, only at glycine concentrations several times higher than the physiological values (i.e. close to 1 mM or above). The mechanism of protection achieved by low-dose glycine treatment regimens is unknown.
- National Center for Biotechnology Information. PubChem. Glycine. https://pubchem.ncbi.nlm.nih.gov/compound/glycine[↩][↩]
- Petrat F, Boengler K, Schulz R, de Groot H. Glycine, a simple physiological compound protecting by yet puzzling mechanism(s) against ischaemia–reperfusion injury: current knowledge. British Journal of Pharmacology. 2012;165(7):2059-2072. doi:10.1111/j.1476-5381.2011.01711.x. https://www.ncbi.nlm.nih.gov/pmc/articles/PMC3413844/[↩][↩][↩][↩][↩]
- Glycine intake decreases plasma free fatty acids, adipose cell size, and blood pressure in sucrose-fed rats. El Hafidi M, Pérez I, Zamora J, Soto V, Carvajal-Sandoval G, Baños G. Am J Physiol Regul Integr Comp Physiol. 2004 Dec; 287(6):R1387-93. http://ajpregu.physiology.org/content/287/6/R1387.long[↩]
- Purves D, Augustine GJ, Fitzpatrick D, et al., editors. Neuroscience. 2nd edition. Sunderland (MA): Sinauer Associates; 2001. GABA and Glycine. Available from: https://www.ncbi.nlm.nih.gov/books/NBK11084/[↩]
- Cytoprotective effects of glycine and glutathione against hypoxic injury to renal tubules. Weinberg JM, Davis JA, Abarzua M, Rajan T. J Clin Invest. 1987 Nov; 80(5):1446-54. https://www.ncbi.nlm.nih.gov/pmc/articles/PMC442402/pdf/jcinvest00095-0238.pdf[↩][↩]
- L-Glycine: a novel antiinflammatory, immunomodulatory, and cytoprotective agent. Zhong Z, Wheeler MD, Li X, Froh M, Schemmer P, Yin M, Bunzendaul H, Bradford B, Lemasters JJ. Curr Opin Clin Nutr Metab Care. 2003 Mar; 6(2):229-40. https://www.ncbi.nlm.nih.gov/pubmed/12589194/[↩][↩][↩][↩]
- Inagawa K., Hiraoka T., Kohda T., Yamadera W., Takahashi M. (2006a). Subjective effects of glycine ingestion before bedtime on sleep quality. Sleep Biol. Rhythms 4, 75–7710.1111/j.1479-8425.2006.00193.x[↩][↩][↩]
- Yamadera W., Inagawa K., Chiba S., Bannai M., Takahashi M., Nakayama K. (2007). Glycine ingestion improves subjective sleep quality in human volunteers, correlating with polysomnographic changes. Sleep Biol. Rhythms 5, 126–13110.1111/j.1479-8425.2007.00262.x[↩][↩][↩]
- The distribution of glycine in cat spinal cord and roots. Aprison MH, Werman R. Life Sci. 1965 Nov; 4(21):2075-83. https://www.ncbi.nlm.nih.gov/pubmed/5866625/[↩]
- The metabolism in vivo of glycine and serine in eight areas of the rat central nervous system. Shank RP, Aprison MH. J Neurochem. 1970 Oct; 17(10):1461-75. https://www.ncbi.nlm.nih.gov/pubmed/5471908/[↩]
- The release of 14C-glycine from electrically stimulated rat spinal cord slices. Hopkin JM, Neal MJ. Br J Pharmacol. 1970 Sep; 40(1):136P-138P. https://www.ncbi.nlm.nih.gov/pmc/articles/PMC1702670/pdf/brjpharm00555-0154.pdf[↩]
- Molecular basis of NMDA receptor functional diversity. Paoletti P. Eur J Neurosci. 2011 Apr; 33(8):1351-65. https://www.ncbi.nlm.nih.gov/pubmed/21395862/[↩]
- Glycine–an important neurotransmitter and cytoprotective agent. Gundersen RY, Vaagenes P, Breivik T, Fonnum F, Opstad PK. Acta Anaesthesiol Scand. 2005 Sep; 49(8):1108-16. https://www.ncbi.nlm.nih.gov/pubmed/16095452/[↩][↩]
- Molecular mechanisms underlying glutamatergic dysfunction in schizophrenia: therapeutic implications. Gaspar PA, Bustamante ML, Silva H, Aboitiz F. J Neurochem. 2009 Nov; 111(4):891-900. https://www.ncbi.nlm.nih.gov/pubmed/19686383/[↩]
- Harvey R.J., Betz H. 2000 Structure, diversity, pharmacology and pathology of glycine receptor chloride channels Pharmacology of Ionic Channel Function: Activators and Inhibitorseds. Endo, M., Kurachi, Y. & Mishina, M. pp 479–499.Berlin: Springer-Verlag[↩]
- Molecular structure and function of the glycine receptor chloride channel. Lynch JW. Physiol Rev. 2004 Oct; 84(4):1051-95. http://physrev.physiology.org/content/84/4/1051.long[↩][↩]
- Molecular mechanisms of inherited startle syndromes. Rajendra S, Schofield PR. Trends Neurosci. 1995 Feb; 18(2):80-2. https://www.ncbi.nlm.nih.gov/pubmed/7537418/[↩][↩]
- Hyperekplexia associated with compound heterozygote mutations in the beta-subunit of the human inhibitory glycine receptor (GLRB). Rees MI, Lewis TM, Kwok JB, Mortier GR, Govaert P, Snell RG, Schofield PR, Owen MJ. Hum Mol Genet. 2002 Apr 1; 11(7):853-60. https://www.ncbi.nlm.nih.gov/pubmed/11929858/[↩]
- Isoform heterogeneity of the human gephyrin gene (GPHN), binding domains to the glycine receptor, and mutation analysis in hyperekplexia. Rees MI, Harvey K, Ward H, White JH, Evans L, Duguid IC, Hsu CC, Coleman SL, Miller J, Baer K, Waldvogel HJ, Gibbon F, Smart TG, Owen MJ, Harvey RJ, Snell RG. J Biol Chem. 2003 Jul 4; 278(27):24688-96. http://www.jbc.org/content/278/27/24688.long[↩]
- Deletion of the mouse glycine transporter 2 results in a hyperekplexia phenotype and postnatal lethality. Gomeza J, Ohno K, Hülsmann S, Armsen W, Eulenburg V, Richter DW, Laube B, Betz H. Neuron. 2003 Nov 13; 40(4):797-806. https://www.ncbi.nlm.nih.gov/pubmed/14622583/[↩]
- Bowery NG, Smart TG. GABA and glycine as neurotransmitters: a brief history. British Journal of Pharmacology. 2006;147(Suppl 1):S109-S119. doi:10.1038/sj.bjp.0706443. https://www.ncbi.nlm.nih.gov/pmc/articles/PMC1760744/[↩][↩]
- Epidemiology of insomnia: what we know and what we still need to learn. Ohayon MM. Sleep Med Rev. 2002 Apr; 6(2):97-111. https://www.ncbi.nlm.nih.gov/pubmed/12531146/[↩]
- Subjective ratings of the characteristics and sequelae of good and poor sleep in normals. Zammit GK. J Clin Psychol. 1988 Mar; 44(2):123-30. https://www.ncbi.nlm.nih.gov/pubmed/3360924/[↩]
- Cognitive deficits in insomnia patients. Hauri PJ. Acta Neurol Belg. 1997 Jun; 97(2):113-7. https://www.ncbi.nlm.nih.gov/pubmed/9246379/[↩]
- Hypnotic activities of chamomile and passiflora extracts in sleep-disturbed rats. Shinomiya K, Inoue T, Utsu Y, Tokunaga S, Masuoka T, Ohmori A, Kamei C. Biol Pharm Bull. 2005 May; 28(5):808-10. https://www.ncbi.nlm.nih.gov/pubmed/15863883/[↩]
- Effectiveness of Valerian on insomnia: a meta-analysis of randomized placebo-controlled trials. Fernández-San-Martín MI, Masa-Font R, Palacios-Soler L, Sancho-Gómez P, Calbó-Caldentey C, Flores-Mateo G. Sleep Med. 2010 Jun; 11(6):505-11. https://www.ncbi.nlm.nih.gov/pubmed/20347389/[↩]
- Cumulative sleepiness, mood disturbance, and psychomotor vigilance performance decrements during a week of sleep restricted to 4-5 hours per night. Dinges DF, Pack F, Williams K, Gillen KA, Powell JW, Ott GE, Aptowicz C, Pack AI. Sleep. 1997 Apr; 20(4):267-77. https://www.ncbi.nlm.nih.gov/pubmed/9231952/[↩]
- Patterns of performance degradation and restoration during sleep restriction and subsequent recovery: a sleep dose-response study. Belenky G, Wesensten NJ, Thorne DR, Thomas ML, Sing HC, Redmond DP, Russo MB, Balkin TJ. J Sleep Res. 2003 Mar; 12(1):1-12. https://www.ncbi.nlm.nih.gov/pubmed/12603781/[↩]
- The cumulative cost of additional wakefulness: dose-response effects on neurobehavioral functions and sleep physiology from chronic sleep restriction and total sleep deprivation. Van Dongen HP, Maislin G, Mullington JM, Dinges DF. Sleep. 2003 Mar 15; 26(2):117-26. https://www.ncbi.nlm.nih.gov/pubmed/12683469/[↩]
- Dynamic aspects of whole body glycine metabolism: influence of protein intake in young adult and elderly males. Gersovitz M, Bier D, Matthews D, Udall J, Munro HN, Young VR. Metabolism. 1980 Oct; 29(11):1087-94. https://www.ncbi.nlm.nih.gov/pubmed/7432172/[↩]
- The glycine receptor. Rajendra S, Lynch JW, Schofield PR. Pharmacol Ther. 1997; 73(2):121-46. https://www.ncbi.nlm.nih.gov/pubmed/9131721/[↩]
- Glycine potentiates the NMDA response in cultured mouse brain neurons. Johnson JW, Ascher P. Nature. 1987 Feb 5-11; 325(6104):529-31. https://www.ncbi.nlm.nih.gov/pubmed/2433595/[↩]
- The glycine site on the NMDA receptor: structure-activity relationships and therapeutic potential. Leeson PD, Iversen LL. J Med Chem. 1994 Nov 25; 37(24):4053-67. https://www.ncbi.nlm.nih.gov/pubmed/7990104/[↩]
- Pharmacokinetics and cerebral distribution of glycine administered to rats. Kawai N, Bannai M, Seki S, Koizumi T, Shinkai K, Nagao K, Matsuzawa D, Takahashi M, Shimizu E. Amino Acids. 2012 Jun; 42(6):2129-37. https://www.ncbi.nlm.nih.gov/pubmed/21647662/[↩]
- Kawai N., Bannai M., Takahashi M. (2009). “Glycine decreases core body temperature and increases cutaneous blood flow via NMDA receptors in rats,” in Neuroscience 2009, Abstract.[↩][↩]
- New therapeutic strategy for amino acid medicine: glycine improves the quality of sleep. Bannai M, Kawai N. J Pharmacol Sci. 2012; 118(2):145-8. https://www.jstage.jst.go.jp/article/jphs/118/2/118_11R04FM/_pdf[↩][↩]
- An hPer2 phosphorylation site mutation in familial advanced sleep phase syndrome. Toh KL, Jones CR, He Y, Eide EJ, Hinz WA, Virshup DM, Ptácek LJ, Fu YH. Science. 2001 Feb 9; 291(5506):1040-3. https://www.ncbi.nlm.nih.gov/pubmed/11232563/[↩]
- Functional consequences of a CKIdelta mutation causing familial advanced sleep phase syndrome. Xu Y, Padiath QS, Shapiro RE, Jones CR, Wu SC, Saigoh N, Saigoh K, Ptácek LJ, Fu YH. Nature. 2005 Mar 31; 434(7033):640-4. https://www.ncbi.nlm.nih.gov/pubmed/15800623/[↩]
- The transcriptional repressor DEC2 regulates sleep length in mammals. He Y, Jones CR, Fujiki N, Xu Y, Guo B, Holder JL Jr, Rossner MJ, Nishino S, Fu YH. Science. 2009 Aug 14; 325(5942):866-70. https://www.ncbi.nlm.nih.gov/pmc/articles/PMC2884988/[↩]
- Melatonin, sleep, and circadian rhythms: rationale for development of specific melatonin agonists. Turek FW, Gillette MU. Sleep Med. 2004 Nov; 5(6):523-32. https://www.ncbi.nlm.nih.gov/pubmed/15511698/[↩][↩]
- Melatonin: characteristics, concerns, and prospects. Arendt J. J Biol Rhythms. 2005 Aug; 20(4):291-303. https://www.ncbi.nlm.nih.gov/pubmed/16077149/[↩][↩]
- The circadian visual system, 2005. Morin LP, Allen CN. Brain Res Rev. 2006 Jun; 51(1):1-60. https://www.ncbi.nlm.nih.gov/pubmed/16337005[↩]
- Bannai M, Kawai N, Ono K, Nakahara K, Murakami N. The Effects of Glycine on Subjective Daytime Performance in Partially Sleep-Restricted Healthy Volunteers. Frontiers in Neurology. 2012;3:61. doi:10.3389/fneur.2012.00061. https://www.ncbi.nlm.nih.gov/pmc/articles/PMC3328957/[↩][↩][↩]
- Expressions of per1 clock gene and genes of signaling peptides vasopressin, vasoactive intestinal peptide, and oxytocin in the suprachiasmatic and paraventricular nuclei of hypertensive TGR[mREN2]27 rats. Dzirbíková Z, Kiss A, Okuliarová M, Kopkan L, Cervenka L. Cell Mol Neurobiol. 2011 Mar; 31(2):225-32. https://www.ncbi.nlm.nih.gov/pubmed/21061153/[↩]
- Indirect projections from the suprachiasmatic nucleus to the ventrolateral preoptic nucleus: a dual tract-tracing study in rat. Deurveilher S, Burns J, Semba K. Eur J Neurosci. 2002 Oct; 16(7):1195-213. https://www.ncbi.nlm.nih.gov/pubmed/12405980/[↩]
- Noradrenergic afferents and receptors in the medial preoptic area: neuroanatomical and neurochemical links between the regulation of sleep and body temperature. Kumar VM, Vetrivelan R, Mallick HN. Neurochem Int. 2007 May; 50(6):783-90. https://www.ncbi.nlm.nih.gov/pubmed/17403554/[↩]
- Human Metabolome Database. Glycine. http://www.hmdb.ca/metabolites/HMDB00123[↩]
- Van Hove J, Coughlin C II, Scharer G. Glycine Encephalopathy. 2002 Nov 14 [Updated 2013 Jul 11]. In: Pagon RA, Adam MP, Ardinger HH, et al., editors. GeneReviews® [Internet]. Seattle (WA): University of Washington, Seattle; 1993-2017. Available from: https://www.ncbi.nlm.nih.gov/books/NBK1357/[↩][↩][↩]
- Glycine transporters and synaptic function. Zafra F, Giménez C. IUBMB Life. 2008 Dec; 60(12):810-7. https://www.ncbi.nlm.nih.gov/pubmed/18798526/[↩]
- Glycine cleavage system: reaction mechanism, physiological significance, and hyperglycinemia. Kikuchi G, Motokawa Y, Yoshida T, Hiraga K. Proc Jpn Acad Ser B Phys Biol Sci. 2008; 84(7):246-63. https://www.ncbi.nlm.nih.gov/pmc/articles/PMC3666648/[↩][↩]
- Compartmentalization of Mammalian folate-mediated one-carbon metabolism. Tibbetts AS, Appling DR. Annu Rev Nutr. 2010 Aug 21; 30():57-81. https://www.ncbi.nlm.nih.gov/pubmed/20645850/[↩]
- Studies on a unique organelle localization of a liver enzyme, serine:pyruvate (or alanine:glyoxylate) aminotransferase. Ichiyama A. Proc Jpn Acad Ser B Phys Biol Sci. 2011; 87(5):274-86. https://www.ncbi.nlm.nih.gov/pmc/articles/PMC3165904/[↩]
- Protective effect of glycine on liver injury during liver transplantation. Wang YS, Yan YH, Zou XF. Chin Med J (Engl). 2010 Jul; 123(14):1931-8. https://www.ncbi.nlm.nih.gov/pubmed/20819580/[↩]
- The role of glycine in hepatic ischemia-reperfusion injury. Habib MM, Hodgson HJ, Davidson BR. Curr Pharm Des. 2006; 12(23):2953-67. https://www.ncbi.nlm.nih.gov/pubmed/16918424/[↩]
- Glycine: a new anti-inflammatory immunonutrient. Wheeler MD, Ikejema K, Enomoto N, Stacklewitz RF, Seabra V, Zhong Z, Yin M, Schemmer P, Rose ML, Rusyn I, Bradford B, Thurman RG. Cell Mol Life Sci. 1999 Nov 30; 56(9-10):843-56. https://www.ncbi.nlm.nih.gov/pubmed/11212343/[↩]
- Dietary glycine prevents the development of liver tumors caused by the peroxisome proliferator WY-14,643. Rose ML, Cattley RC, Dunn C, Wong V, Li X, Thurman RG. Carcinogenesis. 1999 Nov; 20(11):2075-81. https://www.ncbi.nlm.nih.gov/pubmed/10545408/[↩]
- Glycine rinse protects against liver injury during transplantation. Arora AS, Nichols JC, DeBernardi M, Steers JL, Krom RA, Gores GJ. Transplant Proc. 1999 Feb-Mar; 31(1-2):505-6. https://www.ncbi.nlm.nih.gov/pubmed/10083211/[↩]
- Extended experience with glycine for prevention of reperfusion injury after human liver transplantation. Schemmer P, Golling M, Kraus T, Mehrabi A, Mayatepek E, Herfarth Ch, Klar E. Transplant Proc. 2002 Sep; 34(6):2307-9. https://www.ncbi.nlm.nih.gov/pubmed/12270410/[↩]
- Luntz SP, Unnebrink K, Seibert-Grafe M, et al. HEGPOL: Randomized, placebo controlled, multicenter, double-blind clinical trial to investigate hepatoprotective effects of glycine in the postoperative phase of liver transplantation [ISRCTN69350312]. BMC Surgery. 2005;5:18. doi:10.1186/1471-2482-5-18. https://www.ncbi.nlm.nih.gov/pmc/articles/PMC1208918/[↩]
- Supplementation of amino acids to prevent reperfusion injury after liver surgery and transplantation–where do we stand today? Hoffmann K, Büchler MW, Schemmer P. Clin Nutr. 2011 Apr; 30(2):143-7. https://www.ncbi.nlm.nih.gov/pubmed/20965621/[↩]
- Glycine does not add to the beneficial effects of perioperative oral immune-enhancing nutrition supplements in high-risk cardiac surgery patients. Tepaske R, te Velthuis H, Oudemans-van Straaten HM, Bossuyt PM, Schultz MJ, Eijsman L, Vroom M. JPEN J Parenter Enteral Nutr. 2007 May-Jun; 31(3):173-80. https://www.ncbi.nlm.nih.gov/pubmed/17463141/[↩]