Contents
- Flavonoids
- Types of flavonoids
- Flavonoids food
- Flavonoid supplements
- Flavonoids metabolism and bioavailability
- Flavonoids health benefits
- Table 10. Flavonoids biological activity and mechanism of action
- Table 11. Flavonoids rich-foods against chronic diseases
- Table 12. Summary of studies indicating reduced mortalities with intake of flavonoids rich-foods
- Antioxidant activity
- Metal chelation
- Skin health
- Wound healing
- Cardiovascular disease prevention
- Diabetes prevention
- Neuroprotection and cognitive function
- Anticancer activity
- Flavonoids side effects
Flavonoids
Flavonoids are a large family of hydroxylated polyphenolic plant chemicals (polyphenols or phytochemicals) found in many plant products, including teas, fruits, citrus fruits, vegetables, fruit juices, herbs, stems, cereals, nuts, flowers, seeds and some beverages 1, 2, 3, 4, 5. Flavonoids create the vibrant colors of many fruits, vegetables and flowers. In plants flavonoids act as antioxidants, antimicrobials, photoreceptors, visual attractors, feeding repellents, and for light screening 6. Citrus fruits are rich sources of flavonoids 7. Two flavonoids, narigenin and hesperetin, are found in oranges, lemons, and grapes 8. Anthocyanins and quercetin glycosides flavonoids are present in mulberry 9. A higher intake of flavonoid rich foods has a number of health benefits 10. Many studies have suggested that flavonoids exhibit biological activities, including antidiabetic, anti-allergenic, antiviral, antimicrobial, antiangiogenic, antimalarial, neuroprotective, anti-inflammatory, anti-cancer, and widening of blood vessels (vasodilating) actions 6, 11, 12, 13, 14, 15. However, most interest has been devoted to the antioxidant activity of flavonoids which is due to their ability to reduce free radical formation and to scavenge free radicals. Flavonoids have been used in natural dyes 16, 17, in cosmetics and skin care products 18, 19, and anti-wrinkle skin agents 20. Apple peel extracts rich in flavonoids inhibits acetylcholinesterase (ACE) in test tube study and is effective in lowering high blood pressure 21, 22, 23, 24. It also prevents nonalcoholic fatty liver disease (NAFLD) 25 and displays better preservation of cognitive performance with aging 26.
There are more than 10,000 flavonoid compounds that have been isolated and identified and hundreds of flavonoids can exist in a single food item 27, 28. Flavonoids are mainly found in plant cell vacuoles in the form of C-glycosides or O-glycosides 29. The basic molecular structure of flavonoids consists of a 15-carbon skeleton (carbon skeleton can be abbreviated as C6-C3-C6), which contains two phenyl (A & B) rings and (C) heterocyclic ring labeled A, B, and C (see Figure 1 below). According to the IUPAC (International Union of Pure and Applied Chemistry) nomenclature, flavonoids can be categorized into 3 categories: flavonoids or bioflavonoids, isoflavonoids (3-phenyl-1,4-benzopyrone), and neoflavonoids (4-phenyl-1,2-benzopyrone) 30, 31.
Flavonoids could be further classified into 7 major subclasses based on modifications to their basic structures; these subclasses include flavonols (e.g., quercetin, rutin), flavanones (e.g., naringenin, hesperidin), flavanols (e.g., epicatechin, gallocatechin), flavones (e.g., luteolin, apigenin), isoflavones (also called isoflavone are genistein and daidzein), chalcones, and anthocyanins (e.g., pelargonidin, malvidin), with flavonols being the most widespread in the human diet 32, 33.
Figure 1. Flavonoid chemical structure
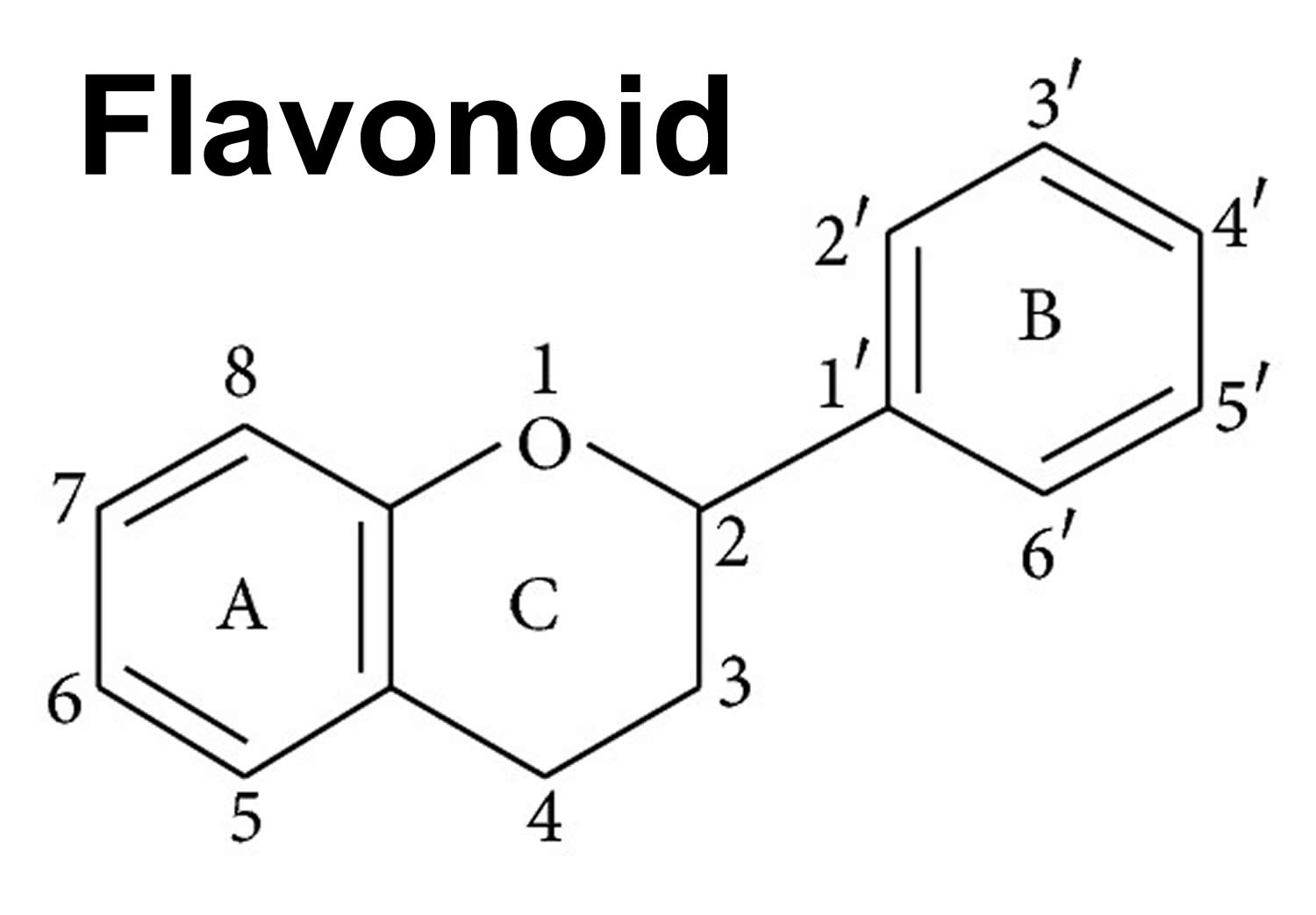
Figure 2. Flavonoids main classes
Figure 3. Types of flavonoids
[Sources 34, 6 ]Figure 4. Flavonoid synthesis pathway
Footnotes: Chalcone synthase (CHS) can catalyze three molecules of malonyl-CoA and one molecule of p-coumaroyl-CoA to form naringeninchalcone 36. Malonyl-CoA is an important precursor for the synthesis of natural products, including flavonoids and polyketides 37. Chalcone isomerase (CHI) converted naringenin-chalcone into flavanones 38. Naringenin, as an important flavonoid skeleton, is catalyzed by flavone synthase 1 (FNS 1) and flavone synthase 2 (FNS 2) and isoflavone synthase (IFS) to form flavones and isoflavones, respectively 39. Furthermore, flavanone-3-hydroxylase (F3H), flavonol 3′-hydroxylase (F3′H), and flavonol 3′5′-hydroxylase (F3′5′H) catalyzed naringenin to generate dihydro-myricetin, dihydro-kaempferol, and dihydro-quercetin, respectively 40. The flavonol synthase (FLS) converted flavanols (dihydroflavonols) into flavonols (kaempferol, quercetin, and myricetin), which was catalyzed by the dihydroflavonol 4-reductase (DFR) to generate leucoanthocyanidins 41, which was catalyzed by leucoanthocyanidin dioxygenase (LDOX) to produce anthocyanidins 42. Anthocyanidins and leucoanthocyanidins were further converted to proanthocyanidins catalyzed by leucoanthocyanidin reductase (LAR) and anthocyanidin reductase (ANR), respectively 43. Modification of anthocyanins is responsible for the stabilization of vacuolar anthocyanins, including glycosylation, methylation, and acylation 44.
[Source 45 ]Figure 5. Flavonoids rich foods potential health benefits
[Source 46 ]Types of flavonoids
Flavonoids are classified into 7 major subclasses based on their chemical structure, degree of unsaturation, and oxidation of carbon ring 47. Six of which, namely anthocyanidins, flavanols (flavan-3-ols or catechins), flavonols, flavones, flavanones, and isoflavones are of dietary significance (Table 1). Glycosylated flavonols (bound to at least one sugar molecule) are the most widely distributed flavonoids in the diet 48, 49.
Table 1. Flavonoids examples
Flavonoid Subclass | Dietary Flavonoids (aglycones) | Common food sources |
---|---|---|
Anthocyanidins* | Cyanidin, Delphinidin, Malvidin, Pelargonidin, Peonidin, Petunidin | Red, blue, and purple berries; red and purple grapes; red wine |
Flavanols (flavan-3-ols or catechins) | Monomers (Catechins): (+)-Catechin, (-)-Epicatechin, (-)-Epigallocatechin, (+)-Gallocatechin; and their gallate derivatives | Teas (particularly white, green, and oolong), cocoa-based products, grapes, berries, apples |
Dimers and Polymers: Proanthocyanidins# | Apples, berries, cocoa-based products, red grapes, red wine | |
Theaflavins, Thearubigins | Black tea | |
Flavonols | Isorhamnetin, Kaempferol, Myricetin, Quercetin | Onions, scallions, kale, broccoli, apples, berries, teas |
Flavones | Apigenin, Luteolin, Baicalein, Chrysin | Parsley, thyme, celery, hot peppers |
Flavanones | Eriodictyol, Hesperetin, Naringenin | Citrus fruit and juices, e.g., oranges, grapefruits, lemons |
Isoflavones | Daidzein, Genistein, Glycitein, Biochanin A, Formononetin | Soybeans, soy foods, legumes |
Footnotes:
* Anthocyanidins with one or more sugar moieties (anthocyanidin glycosides) are called anthocyanins.
# Proanthocyanidin oligomers formed from (+)-catechin and (-)-epicatechin subunits are called procyanidins.
Anthocyanins
Anthocyanins are the specific type of flavonoids that give most flowers, seeds, fruits, and vegetables their vibrant color from orange, red, and purple to blue, depending on the pH of the micro-environment of the flowers, seeds, fruits, and vegetative tissues 51. Anthocyanins are a group of deep red, purple and blue pigments mainly found in the outer cell layer of various fruits and vegetables, such as blackcurrants, grapes, and berries 52, 53. Anthocyanins play important roles in visual acuity, cholesterol decomposition, and reducing risk of cardiovascular disease in humans 54, 55. In addition, anthocyanins are commonly used as food colorants 56.
The position and number of hydroxyl and methoxyl groups present as substituents in the flavylium structure result in different anthocyanins 45. Therefore, over 650 anthocyanins have been identified in many plants 57; these are grouped into the following six categories: cyanidin, delphinidin, malvidin, pelargonidin, peonidin, petunidin, and their corresponding derivatives 51, 58, 59. The antioxidant ability of anthocyanins is associated with their ring orientation and the position and number of free hydroxyls around the pyrone ring.
There are six main types of anthocyanins:
- Cyanidin
- Delphinidin
- Malvidin
- Pelargonidin
- Peonidin
- Petunidin
You can find anthocyanins in foods like:
- Black plums
- Cranberries
- Blueberries
- Strawberries
- Blackberries
- Cherries
- Bananas
- Red, black and purple grapes
- Pomegranates
- Red wine
- Oranges including blood oranges.
Berries have the highest levels of anthocyanins, particularly black elderberries and aronia berries (chokeberries). Blueberries, blackberries, raspberries and strawberries are also great sources. Additional foods with a high anthocyanin punch include:
- Vegetables: Red cabbage, red onions, red radishes, purple cauliflower, purple corn and the skin of purple eggplant.
- Legumes and rice: Black beans, black rice and black soybeans.
- Beverages: Grape juice and wine.
Fresh and frozen forms of the foods listed retain the highest levels of anthocyanins. You’ll also find many types of anthocyanin-containing supplements. Extracts of elderberry, aronia, tart cherry and blueberry are just a few that have become popular. However, unlike anthocyanin-rich foods are an important part of a healthy diet, there’s no clear evidence supporting the use of anthocyanin supplements. While anthocyanin supplements are widely available and claim many health benefits, data guiding the use of these products is generally nonexistent.
Researchers are still investigating the role of anthocyanins in treating and preventing specific diseases. Overall, evidence suggests that anthocyanins may provide a range of health benefits.
- Lowers blood pressure. Anthocyanins may help reduce blood pressure, according to a review of 66 studies 60. Study participants with high blood pressure (hypertension) took a variety of extracts and preparations from anthocyanin-containing plants. The study found there was a consistent lowering effect on blood pressure. In other studies, however, researchers identified many factors that led to mixed results, such as:
- Dosage and type of anthocyanin (food, juice, freeze-dried powder or extract).
- Number of doses (single vs. long term).
- Patient characteristics (a person’s baseline blood pressure and other health conditions).
- This study highlights the need for more research to establish a dosing regimen and determine who might benefit from anthocyanins.
- Reduces risk of heart disease. Atherosclerosis is when plaque builds up on the inside of your arteries. This buildup can cause high blood pressure, heart attack, stroke, peripheral artery disease or kidney failure. The formation of plaque is a multi-stage process that depends on many factors. Research shows that anthocyanins can intervene at different stages in the plaque formation process by reducing:
- Cholesterol, a main part of plaque.
- High blood pressure, which can damage blood vessels and make them more likely to develop atherosclerosis.
- Inflammation, which leads to plaque formation.
- Prevents neurological diseases. Research shows that anthocyanins can help protect and improve your brain function.
- One study found that a daily dose of cherry juice improved speech and memory in people aged 70 and over with mild or moderate dementia 61.
- Another study reported anthocyanins increased blood flow to and activated brain areas that control memory, language and attention.
- Researchers believe that anthocyanins’ antioxidant and anti-inflammatory properties are responsible for these improvements.
- Slows cancer growth. Anthocyanins may act in many ways to slow or stop cancer. Studies suggest that anthocyanins might 62:
- Block the changes in DNA that cause cancer.
- Destroy cancer cells or stop their growth.
- Prevent tumors from becoming malignant (cancerous).
- Reverse drug resistance and increase tumors’ sensitivity to chemotherapy.
However, research on anthocyanins and cancer have mainly happened in the laboratory. There are only limited data from clinical trials on people. Researchers need to continue to investigate these findings so they can determine exactly how anthocyanins affect cancer growth and treatment.
Flavanols (Flavan-3-ols)
Flavanols also called catechins, flavan-3-ols or dihydroflavonols are characterized by a hydroxyl group at position 3 in the C-ring 63, 64. Unlike other flavonoids, flavanols lack a double bond between positions 2 and 3 in the C-ring 65. Several flavanols, including catechin, epigallocatechin gallate (EGCG), gallocatechin, epicatechin, epicatechin gallate (ECG), catechin 3-gallate, and epicatechin 3-gallate, are widely distributed in many fruits (e.g., apples, bananas, pears, and blueberries) 66, 67, 65, 68. Flavanols can protect blood vessels against tobacco by increasing the content of nitric oxide (NO) in blood vessels. A flavanol-rich diet can facilitate the permanent improvement of endothelial function and prevent the development of cardiovascular diseases 69, 70.
Flavanols (catechins or flavan-3-ols) can be found in foods like:
- Black tea
- Green tea
- Cocoa products
- Cinnamon
- Grapes
- Red wine
- Apples.
Flavonols
Flavonols also called 3-hydroxy flavone, can be identified by specific substitutions in their A- and B-rings, which are connected by a three-carbon chain 71. Flavonols possess hydroxyl groups at positions 5 and 7 in the A-ring and are mainly present in epidermal cells to protect DNA against UV-induced damage 72. Four types of flavonol compounds (quercetin, galangin, myricetin and fisetin) are mainly distributed in vegetables and fruits, such as asparagus, onions, lettuce, broccoli, tomato, and apples 73. Flavonols exhibit interesting biological activities, including antioxidant, antibacterial, cardioprotective, anticancer, and antiviral activities 45. Dietary flavonols can significantly decrease the risk of gastric cancer in smokers and in women 45.
Flavones
Flavones, are one of the important subgroups of flavonoids, consist of 4H-chromen-4-one bearing a phenyl substituent at position 2 45. Flavones mostly occur as 7-O-glycosides, which are found in celery, parsley, red pepper, chamomile, mint, and ginkgo biloba 3, 74, 75. Apigenin, luteolin and and tangeritin are the common flavones 45. In nature, apigenin is usually found in a glycosylated form, with a sugar moiety attached to the tricyclic core structure via hydroxyl groups (O-glycosides) or directly to carbon (C-glycosides) 76. The principal ingredients of apigenin are glycosylated apiin, apigenin, vitexin, isovitexin, or rhoifolin. Apigenin can scavenge free radicals and regulate antioxidant enzyme activity in pancreatic cells, and apigenin can decrease inflammation in cancer, neuroinflammation, and cardiovascular diseases 77, 78. The peels of citrus fruits are rich in the polymethoxylated flavones, tageretin, nobiletin and sinensetin 48. Most flavones of vegetables and fruits have a hydroxyl group in position 5 of the A ring, while hydroxylation in other positions, for the most part in position 7 of the A ring or 3′ and 4′ of the B ring, may vary according to the taxonomic classification of the particular vegetable or fruit.
Flavones can be found in ingredients like:
- Hot peppers
- Thyme
- Parsley
- Celery
- Oregano
- Peppermint
- Chamomile.
Flavanones
Flavanones (dihydro-flavones) possess a saturated C-ring 79. The saturated double bond between positions 2 and 3 in the C-ring represents the only structural difference between flavanones and other flavonoid compounds 80. Flavanones are abundant in citrus fruits and citrus fruit juices, including oranges, lemons, mandarins, grapefruits, clementines, and limes 81, 82. Flavanones contain hydroxyl groups at positions 5 and 7 in the A-ring and possess hydroxyl/methoxy substituents at the C3 or C4 positions of the B-ring 83. The defining characteristic of flavanones is a disaccharidic moiety linked to the seven positions of aglycone 84. Depending on their structural differences, flavanones can occur in the form of naringin, naringenin, hesperidin, hesperetin, pinocembrin, likvirtin, and eriodictyol 85. Among them, naringenin and hesperetin, as the main dietary flavanones, occur almost exclusively in citrus fruits 82, 86. Naringin can increase the activity of antioxidant enzymes (CAT, PON, GPx, and SOD) and enhance the immune system. Furthermore, naringenin and hesperetin have been shown to recover impaired thyroid function in rats 45.
Flavanones can be found in foods like 87:
- Lemons
- Limes
- Grapefruits
- Mandarins
- Oranges
- Tomatoes
Figure 6. Citrus flavanones chemical structures
[Source 88 ]Isoflavones
Isoflavones have a B-ring at the C3 position of the heterocyclic C-ring of the diphenylpropane (C6–C3–C6) backbone, which represents their only chemical structural difference from other flavonoids 89. Isoflavones are characteristic metabolites of leguminous plants and play essential roles in nodule induction and microbial signaling in legumes 90, 91. Isoflavones are classified into three groups: genistein, daidzein, and glycitein 92. The molecular structure of isoflavones is similar to that of animal estrogens. Isoflavones are plant estrogens (phytoestrogens) that have potent estrogenic activity. Phytoestrogens are similar in structure to the human female hormone 17-beta-estradiol in that they bind to estrogen receptors 93. In addition, isoflavones possess a strong antioxidant activity, which can decrease the risk of cancers by inhibiting free radical-induced DNA damage 93.
Chalcones
Chalcones (1,3-diaryl-2-propen-1-ones) are natural open-chain flavonoids, carrying up to three modified or unmodified C5-, C10-, and C15-prenyl moieties on both their A and B-rings 45. Chalcones products are widely distributed in the legumes family, Moraceae (mulberry family or fig family), ginger family (Zingiberaceae) and hemp family (Cannabaceae) 77. Chalcones exhibit a wide spectrum of pharmacological effects, including antioxidant, antibacterial, anthelmintic, antiulcer, antiviral, antiprotozoal, and anticancer effects 94. Chalcones are precursors of flavonoids and isoflavonoids 45. Their structural features are easily constructed from simple aromatic compounds. Their prominent bioactivity has inspired the synthesis of chalcone analogs, as well as minor structural modifications to natural chalcones; these compounds form a large collection of bioactive chalcone derivatives 95. Xanthohumol and isbavirachalone are two chalcones derivatives that exhibit abundant biological and pharmacological activity 54.
Flavonoids food
The main dietary sources of flavonoids include tea, citrus fruit, citrus fruit juices, berries, red wine, apples, and legumes 30, 50. Tea, wine, leafy vegetables, onions, apples, berries, cherries, soybeans, and citrus fruits are the primary dietary sources of flavonoids in eastern and western societies 31, 96, 97, 46. Individual flavonoid intakes may vary considerably depending on whether tea, red wine, soy products, or fruit and vegetables are commonly consumed 48. Recent data analyses of the National Health and Nutrition Examination Survey (NHANES) estimated flavonoid intakes in US adults (aged ≥19 years) average between 200 and 250 mg/day, with 80% being flavan-3-ols, 8% for flavonols, 6% for flavanones, 5% for anthocyanidins, and ≤1% for isoflavones and flavones 98, 99.
Information on the flavonoid content of some flavonoid-rich foods is presented in Tables 2 below. These values should be considered approximate since a number of factors may affect the flavonoid content of foods, including agricultural practices, environmental conditions, ripening, storage, and food processing. For additional information about the flavonoid content of food, the USDA provides databases for the content of selected foods in flavonoids 100 and proanthocyanidins 101. For more information on the isoflavone content of soy foods, see the USDA database for the isoflavone content of selected foods 102.
Table 2. Flavonoids-rich Foods
Flavonoid | Class | Dietary sources |
---|---|---|
Quercetin | Flavonols | Vegetables, fruits and beverages, spices, soups, fruit juices |
Rutin | Flavonols | Green tea, grape seeds, red pepper, apple, citrus fruits, berries, peaches |
Macluraxanthone | Xanthones | Maclura tinctoria (Hedge apple), Dyer’s mulberry |
Genistein | Isoflavone | Fats, oils, beef, red clover, soyabeans, psoralea, lupin, fava beans, kudzu, psoralea |
Scopoletin | Coumarin | Vinegar, dandelion coffee |
Daidzein | Isoflavone | Soyabeans, tofu |
Taxifolin | Flavanonol | Vinegar |
Naringenin | Flavanone | Grapes |
Abyssinones | Flavanone | French bean seeds |
Rutin | Flavonol | Citrus fruits, apple, berries, peaches |
Eriodictyol | Flavanone | Lemons, rosehips |
Fisetin | Flavonol | Strawberries, apples, persimmons, onions, cucumbers |
Theaflavin | Catechins | Tea leaves, black tea, oolong tea |
Peonidin | Anthocyanidin | Cranberries, blueberries, plums, grapes, cherries, sweet potatoes |
Diosmetin | Flavone | Vetch |
Tricin | Flavone | Rice bran |
Biochanin | Isoflavone | Red clover, soya, alfalfa sprouts, peanuts, chickpeas (Cicer arietinum), other legumes |
Hesperidin | Flavanone | Bitter orange, petit grain, orange, orange juice, lemon, lime |
Epicatechin | Flavan-3-ols | Milk, chocolate, commercial, reduced fat |
Myricetin | Flavonols | Vegetables, fruits, nuts, berries, tea, red wine |
Taxifolin | Flavanonol | Citrus fruits |
Kaempferol | Flavonols | Apples, grapes, tomatoes, green tea, potatoes, onions, broccoli, Brussels sprouts, squash, cucumbers, lettuce, green beans, peaches, blackberries, raspberries, spinach |
Luteolin | Flavones | Celery, broccoli, green pepper, parsley, thyme, dandelion, perilla, chamomile tea, carrots, olive oil, peppermint, rosemary, navel oranges, oregano |
Apigenin | Flavones | Milk, chocolate, commercial, reduced fat |
Table 3. Anthocyanidin-rich foods
Anthocyanidins (mg/100 g or 100 mL*) | ||||||
---|---|---|---|---|---|---|
Food | Cyanidin | Delphinidin | Malvidin | Pelargonidin | Peonidin | Petunidin |
Blackberries, raw | 100 | 0 | 0 | <1 | <1 | 0 |
Blood orange juice | 5.5 | <1 | – | – | <1 | – |
Blueberries, raw | 8.5 | 35.4 | 67.6 | 0 | 20.3 | 31.5 |
Currants, black, raw | 62.5 | 89.6 | – | 1.2 | <1 | 3.9 |
Elderberries, raw | 485.3 | 0 | – | <1 | – | 0 |
Grapes, red | 1.2 | 2.3 | 39 | <1 | 3.6 | 2 |
Onions, red, raw | 3.2 | 4.3 | – | <1 | 2.1 | – |
Plums, raw | 5.6 | 0 | 0 | 0 | <1 | 0 |
Radishes, raw | 0 | 0 | 0 | 63.1 | 0 | 0 |
Raspberries, raw | 45.8 | 1.3 | <1 | 1 | <1 | <1 |
Red cabbage, raw | 209.8 | <1 | – | <1 | – | – |
Strawberries, raw | 1.7 | <1 | <1 | 24.9 | <1 | <1 |
Wine, red, Shiraz | – | 9.3 | 121.6 | – | 7.8 | 14.2 |
Footnote: * per 100 g (fresh weight) or 100 mL (liquids); 100 grams is equivalent to about 3.5 ounces; 100 mL is equivalent to about 3.5 fluid ounces.
[Source 50 ]Table 4. Flavan-3-ol-rich foods
Food | Flavon-3-ol Monomers and Thearubigins (mg/100 g or 100 mL*) | ||||||
---|---|---|---|---|---|---|---|
Catechin (C) | Gallocatechin (GC) | Epicatechin (EC) | Epicatechin gallate (ECG) | Epigallocatechin (EGC) | Epigallocatechin gallate (EGCG) | Thearubigins | |
Apples, Red Delicious, raw, with skin | 2 | 0 | 9.8 | 0 | <1 | <1 | – |
Apricots, raw | 3.7 | 0 | 4.7 | 0 | 0 | 0 | – |
Chocolate, dark | 24.2 | – | 84.4 | – | – | – | – |
Tea, black, brewed | 1.5 | 1.2 | 2.1 | 5.9 | 8 | 9.4 | 81.3 |
Tea, green, brewed | 4.5 | 1.5 | 8.3 | 17.9 | 29.2 | 70.2 | 1.1 |
Tea, oolong, brewed | <1 | – | 2.5 | 6.3 | 6.1 | 34.5 | – |
Tea, white, brewed | – | – | – | 8.3 | 18.6 | 42.4 | – |
Wine, red, Shiraz | 6.8 | – | 10 | – | – | – | – |
Footnote: * per 100 g (fresh weight) or 100 mL (liquids); 100 grams is equivalent to about 3.5 ounces; 100 mL is equivalent to about 3.5 fluid ounces.
[Source 50 ]Table 5. Proanthocyanidin rich foods
Food | Proanthocyanidins (Flavon-3-ol Polymers) (mg/100 g or 100 mL*) | |||
---|---|---|---|---|
Monomers | Dimers | Trimers | ≥4mers | |
Apples, Red Delicious, raw, with skin | 8.3 | 15.1 | 10.1 | 94.2 |
Baking chocolate, unsweetened | 198.5 | 206.5 | 130.9 | 1100 |
Cocoa, dry powder, unsweetened | 316.6 | 183.5 | 159.5 | 713.4 |
Cranberries, raw | 7.3 | 25.9 | 18.9 | 385.6 |
Currants, black, raw | <1 | 2.9 | 3 | 142.9 |
Grapes, red, raw | 1.4 | 2.4 | 1 | 56.9 |
Nuts, pecan | 17.2 | 42.1 | 26 | 408.6 |
Nuts, pistachio | 10.9 | 13.3 | 10.5 | 202.6 |
Peaches, yellow, with peel, raw | 4.5 | 12.2 | 4.4 | 50.6 |
Plums, with peel, raw | 10.9 | 38.5 | 22.2 | 149.1 |
Spices, cinnamon, ground | 23.9 | 256.3 | 1252.2 | 6576 |
Strawberries, raw | 3.7 | 5.3 | 4.9 | 127.8 |
Wine, table, red | 16.6 | 20.5 | 1.8 | 22.7 |
Footnote: * per 100 g (fresh weight) or 100 mL (liquids); 100 grams is equivalent to about 3.5 ounces; 100 mL is equivalent to about 3.5 fluid ounces.
[Source 50 ]Table 6. Flavonol-rich foods
Food | Flavonols (mg/100 g or 100 mL*) | |||
---|---|---|---|---|
Isorhamnetin | Kaempferol | Myricetin | Quercetin | |
Blueberries, raw | – | 1.7 | 1.3 | 7.7 |
Broccoli, raw | – | 7.8 | <1 | 3.3 |
Chili peppers, green, raw | – | 0 | 1.2 | 14.7 |
Cowpea, black seeds, raw | – | 1.9 | 2.7 | 17.2 |
Kale, raw | 23.6 | 46.8 | 0 | 22.6 |
Onions, red, raw | 4.6 | <1 | 2.2 | 39.2 |
Parsley, fresh | 0 | 1.5 | 14.8 | <1 |
Rocket, wild, raw | <1 | 1.8 | – | 66.2 |
Scallions, raw | – | 1.4 | 0 | 10.7 |
Spinach, raw | – | 6.4 | <1 | 4 |
Tea, black, brewed | – | 1.4 | <1 | 2.2 |
Tea, green, brewed | – | 1.3 | 1 | 2.5 |
Watercress, raw | 0 | 23 | <1 | 30 |
Footnote: * per 100 g (fresh weight) or 100 mL (liquids); 100 grams is equivalent to about 3.5 ounces; 100 mL is equivalent to about 3.5 fluid ounces.
Table 7. Flavone-rich foods
Food | Flavones (mg/100 g or 100 mL*) | |
---|---|---|
Apigenin | Luteolin | |
Celery hearts, green | 19.1 | 3.5 |
Celery, raw | 2.8 | 1 |
Chili peppers, green, raw | 1.4 | 3.9 |
Oregano, fresh | 2.6 | 1 |
Parsley, fresh | 215.5 | 1.1 |
Peppermint, fresh | 5.4 | 12.7 |
Thyme, fresh | 2.5 | 45.2 |
Footnote: * per 100 g (fresh weight) or 100 mL (liquids); 100 grams is equivalent to about 3.5 ounces; 100 mL is equivalent to about 3.5 fluid ounces.
[Source 50 ]Table 8. Flavanone-rich foods
Food | Flavanones (mg/100 g or 100 mL*) | ||
---|---|---|---|
Eriodictyol | Hesperetin | Naringenin | |
Grapefruit juice, white, fresh | <1 | 2.3 | 18.2 |
Grapefruit, white, raw | – | <1 | 21.3 |
Lemon juice, fresh | 4.9 | 14.5 | 1.4 |
Lemon, raw | 21.4 | 27.9 | <1 |
Orange juice, fresh | <1 | 12 | 2.1 |
Orange, raw | – | 27.2 | 15.3 |
Pummelo juice, fresh | 2.9 | 1.8 | 25.3 |
Footnote: * per 100 g (fresh weight) or 100 mL (liquids); 100 grams is equivalent to about 3.5 ounces; 100 mL is equivalent to about 3.5 fluid ounces.
[Source 50 ]Table 9. Isoflavone-rich foods
Food | Isoflavones (mg/100 g or 100 mL*) | ||
---|---|---|---|
Diadzein | Genistein | Glycitein | |
Black bean sauce | 6 | 4 | <1 |
Natto | 33.2 | 37.7 | 10.5 |
Soybeans, mature seeds, raw | 62.1 | 81 | 15 |
Soymilk, low-fat | 1 | 1.5 | <1 |
Tofu, firm, cooked | 10.3 | 10.9 | 1.3 |
Footnote: * per 100 g (fresh weight) or 100 mL (liquids); 100 grams is equivalent to about 3.5 ounces; 100 mL is equivalent to about 3.5 fluid ounces.
[Source 50 ]Flavonoid supplements
Anthocyanins
Bilberry, elderberry, black currant, blueberry, red grape, and mixed berry extracts that are rich in anthocyanins are available as dietary supplements without a prescription in the US. The anthocyanin content of these products may vary considerably. Standardized extracts that list the amount of anthocyanins per dose are available.
Flavanols (Flavan-3-ols)
Numerous tea extracts are available in the US as dietary supplements and may be labeled as tea catechins or tea polyphenols. Green tea extracts are the most commonly marketed, but black and oolong tea extracts are also available. Green tea extracts generally have higher levels of catechins (flavan-3-ol monomers), while black tea extracts are richer in theaflavins and thearubigins (tea flavan-3-ol dimers and polymers, respectively). Oolong tea extracts fall somewhere in between green and black tea extracts with respect to their flavan-3-ol content. Some tea extracts contain caffeine, while others are decaffeinated. Flavan-3-ol and caffeine content vary considerably among different products, so it is important to check the label or consult the manufacturer to determine the amounts of flavan-3-ols and caffeine that would be consumed daily with each supplement.
In clinical trials employing caffeinated green tea extracts, cancer patients who took 6 g/day in three to six divided doses reported mild-to-moderate gastrointestinal side effects, including nausea, vomiting, abdominal pain, and diarrhea 103, 104. Central nervous system symptoms, including agitation, restlessness, insomnia, tremors, dizziness, and confusion, have also been reported. In one case, confusion was severe enough to require hospitalization 103. In a systematic review published in 2008, the US Pharmacopeia (USP) Dietary Supplement Information Expert Committee identified 34 adverse event reports implicating the use of green tea extract products (containing 25%-97% of polyphenols) as the likely cause of liver damage (hepatotoxicity) in humans 105. In a four-week clinical trial that assessed the safety of decaffeinated green tea extracts (800 mg/day of epigallocatechin gallate [EGCG]) in healthy individuals, a few of the participants reported mild nausea, stomach upset, dizziness, or muscle pain 105. In the Minnesota Green Tea Trial 106, 1,075 postmenopausal women were randomized to receive green tea extracts (1,315±116 mg/day of catechins; the equivalent of four 8-ounce mugs of brewed decaffeinated green tea) or a placebo for one year. The total number of adverse events and the number of serious adverse events were not different between the treatment and placebo groups 106. However, the use of green tea extracts was directly associated with abnormally high liver enzyme levels in 7 out of the 12 women who experienced serious adverse events. Also, the incidence of nausea was twice as high in the green tea arm as in the placebo group 106.
In a recent randomized, double-blind, controlled study in healthy adults, the daily intake of 2 g of cocoa flavan-3-ols for 12 weeks was found to be well tolerated with no adverse side effects 107.
Quercetin
Extracts of quercetin have been purported to be beneficial to health, improving metabolic function, modulating inflammation and decreasing serum cholesterol. Animal, test tube and human studies suggest a long list of desirable effects result from consuming quercetin in the diet. These include antioxidative, anti-inflammatory, antibacterial, immunomodulatory, anticarcinogenic, and cardioprotective actions 118 and of the food mutagen 2-amino-3-methylimadazo[4,5-f]-quinoline (IQ) in lymphocytes from patients with inflammatory bowel disease (IBD). Mutagenesis. 2009;(5):405-411.)), 119, 120, 121, 122, 123. High quercetin intake via diet is associated with decreased rates of colorectal, kidney, pancreatic, prostate, and lung cancer; cardiovascular disease; and diabetes 124, 125, 126, 127, 128, 129, 130, 131, 132.
Quercetin has been purported to improve athletic performance and reduce postexercise inflammation and oxidative stress. The diverse potential beneficial effects of quercetin have led it to be marketed in multiple dietary supplements with many health claims. Quercetin supplements have been used to treat or prevent diverse conditions including cardiovascular disease, high cholesterol (hypercholesterolemia), rheumatic diseases, infections and cancer but have not been shown to be effective in clinical trials for any medical condition.
The beneficial effects of quercetin have been suggested by several studies of human nutrition which have shown decreases in illness and death associated with higher intake of quercetin-rich foods such as fruits and green vegetables. Flavonoids have antioxidant effects that may protect against cell injury. In test tube study, antioxidants such as quercetin have been shown to decrease oxidation of cholesterol esters which appears to play a role in atherosclerosis. However, it is quite difficult to demonstrate that specific food components in isolation such as flavonoids have biologically important antioxidant effects in the human body. Quercetin also has anti-inflammatory activity inhibiting cyclooxygenase (COX) enzymes, synthesis of leukotrienes and prostaglandins and inhibiting histamine release. In cell culture and animal models, quercetin appears to have anti-cancer effects which have led to its evaluation in several forms of cancer, although some studies have used concentrations of the flavonoids that are 100 times or higher than achieved by eating diets high in flavonoid-containing foods. To put the concentrations used in typical cell culture studies into perspective, it was estimated that blood concentrations of quercetin ranging between about 50 nmol/L and about 140 nmol/L are reached for persons consuming their habitual diets and diets high in vegetables, fruits, and berries, respectively 133. Nieman et al. 134 reported achieving levels of 1,158 µg/L, or about 3.8 µmol/L, in test subjects receiving 1,000 mg of quercetin daily for several weeks. These blood levels are substantially below those of most test tube trials. It is not known whether concentrations of quercetin higher than plasma levels might be achieved in other tissues after consuming quercetin from diet. However, it appears that the majority of test tube studies have involved quercetin levels that are not achieved even with high-dose dietary supplements. The applicability of the findings of these high-dose test tube studies to human health and safety is questionable.
In a double-blind, randomized, crossover study, MacRae and Mefferd 135 investigated whether 6 weeks of supplementation with an antioxidant would enhance the performance of elite male cyclists in 30-km time trials. The supplement contained a variety of nutrient and nonnutrient antioxidants, and included a total of 600 mg of quercetin. The results showed that the supplement improved the time trial performance and enhanced power output 135. These findings could not be attributed to quercetin, however, as there was no quercetin-only supplement. Nieman et al. 134 tested whether 1,000 mg/day of quercetin would have an effect on upper respiratory tract infections (URTI) and exercise-induced changes in immune function in trained male cyclists (n=40). Participants were randomized to receive quercetin or placebo supplements twice daily under double-blind conditions for 3 weeks prior to, during, and 2 weeks following a 3-day period in which subjects cycled at high output for 3 hours per day 134. The results of this trial showed no effects on natural killer cell activity, PHA-stimulated lymphocyte proliferation, polymorphonuclear oxidative burst activity, or salivary immunoglobulin A (IgA) output. However, the incidence of upper respiratory tract infections (URTI) during the 2-week postexercise period differed significantly between groups, with quercetin resulting in only one versus nine episodes of URTI in the placebo group. Interestingly, plasma quercetin was increased from 113 µg/L in the placebo group to 1,158 µg/L in supplemented groups. The authors concluded that even in the absence of demonstrated effects of the supplement on multiple measures of immune function, quercetin may have a direct antiviral mechanism 134.
Davis et al. 136 evaluated the effects of 7 days of an oral gavage of quercetin (either 12.5 or 25 mg/kg body weight) on tissue mitochondrial enzymes and performance on a treadmill in previously sedentary mice. Both dose levels of quercetin were associated with significant increases in mitochondrial content in skeletal muscle and brain cells as well as increased endurance capacity in the mice.
Oral supplementation with quercetin glycosides at doses ranging between 3 mg/day to 1,000 mg/day for up to three months has not resulted in significant adverse effects in clinical studies 137. A randomized, placebo-controlled study in 30 patients with chronic prostatitis reported one case of headache and another of tingling of the extremities associated with supplemental quercetin (1,000 mg/day for one month); both issues resolved after the study ended 138. In a phase 1 clinical trial in cancer patients unresponsive to standard treatments, administration of quercetin via intravenous infusion resulted in symptoms of nausea, vomiting, sweating, flushing, and dyspnea (difficulty breathing) at doses ≥10.5 mg/kg body weight (~756 mg of quercetin for a 70 kg individual) 139. Higher doses of quercetin up to 51.3 mg/kg body weight (~3,591 mg of quercetin) were associated with kidney toxicity, yet without evidence of nephritis, infection, or obstructive uropathy 137. Okamoto 140 concluded that it is unlikely that administration of quercetin at a typical dosage could cause any adverse effect. There do not appear to be any safety concerns about quercetin supplements at doses of 1,000 mg daily or less. However, most dietary supplements currently on the market contain mixtures of compounds, not just quercetin in isolation. There are no clear interactions of quercetin with other dietary supplements or medications.
Flavanones
Citrus bioflavonoid supplements may contain glycosides of hesperetin (hesperidin), naringenin (naringin), and eriodictyol (eriocitrin). Hesperidin is also available in hesperidin-complex supplements, with daily doses from 500 mg to 2 g 141.
Flavones
The peels and tissues of citrus fruit (e.g., oranges, tangerines, and clementines) are rich in polymethoxylated flavones: tangeretin, nobiletin, and sinensetin 48. Although dietary intakes of these naturally occurring flavones are generally low, they are often present in citrus bioflavonoid complex supplements. Several dietary supplements may also contain various amounts of baicalein (aglycone) and/or baicalin (glycoside). Some tea preparations may also include baicalein-7-glucuronide 141.
Flavonols
The flavonol aglycone, quercetin, and its glycoside rutin are available as dietary supplements without a prescription in the US. Other names for rutin include rutoside, quercetin-3-rutinoside, and sophorin 141. Citrus bioflavonoid supplements may also contain quercetin or rutin.
Isoflavones
A 50-mg soy isoflavone supplement usually includes glycosides of the isoflavones: genistein (genistin; 25 mg), daidzein (daidzin; 19 mg), and glycitein (glycitin; about 6 mg). Smaller amounts of daidzein, genistein, and formononetin are also found in biochanin A-containing supplements (derived from red clover) 141.
Flavonoids metabolism and bioavailability
The amount of flavonoids present in ingested food has little importance unless dietary flavonoids are absorbed and become available to target tissues within the body. During and after intestinal absorption, flavonoids are rapidly and extensively metabolized in intestinal and liver cells such that they are likely to appear as metabolites (e.g., phase 2 metabolites) in the bloodstream and urine 142. Additionally, the biological activities of flavonoid metabolites are likely to be different from those of their parent compounds 143.
Most flavonoids occur in edible plants and foods as beta-glycosides, i.e., bound to one or more sugar molecules 144. Exceptions include flavan-3-ols (catechins and proanthocyanidins) and fermented soy-based products that are exposed to microbial beta-glucosidases, which catalyze the release of sugar molecules from glycosylated isoflavones 145. Even after food processing and cooking, most flavonoid glycosides reach the small intestine intact. Only flavonoid aglycones (not bound to a sugar molecule) and a few flavonoid glucosides (bound to glucose) are easily absorbed in the small intestine 146. Glycosylated flavonoids might be able to penetrate the mucus layer of the intestine and be deglycosylated on the cell surface before absorption. Those that cannot be deglycosylated in the small intestine may be hydrolyzed by bacterial enzymes in the large intestine (the colon) 145. Nevertheless, colonic bacteria might remove sugar moieties and rapidly degrade aglycone flavonoids, thus limiting their absorption in the colon 147.
In contrast to monomeric flavan-3-ols (catechins), the polymeric nature of proanthocyanidins likely prevents their intestinal absorption. Flavan-3-ol monomers and procyanidins are transformed by the intestinal microbiota to 5-(hydroxyphenyl)-γ-valerolactones which appear in the circulatory system and are excreted in urine as sulfate and glucuronide metabolites 50. Valerolactones may be further degraded by the colonic microbiota to smaller phenolic acids and aromatic compounds. The colonic microbiota also metabolize the gallate esters of flavonoids, generating gallate, which is further catabolized to pyrogallol. Microbe-derived flavonoid metabolites are readily absorbed into the circulatory system and excreted in both free forms and as phase 2 metabolites in urine 146.
In the large intestine (the colon), gut microbial enzymes transform flavonoids through deglycosylation, ring fission, dehydroxylation, demethylation, etc. into metabolites that can then be absorbed or excreted 147, 148. The diversity and activity of colonic bacteria, which are partly dependent on a person’s dietary habits, will determine which metabolites can be produced from ingested flavonoids 149, 150. The composition of the colonic microbiota can therefore affect the metabolic fate and bioavailability of dietary flavonoids 151.
The presence of macronutrients in food can aslo influence the bioavailability of co-ingested flavonoids 146, 152, 153. The binding affinity and potential interactions of flavonoids with food proteins, carbohydrates, and fats are directly associated with the physicochemical properties of flavonoids 146. Proteins in milk might reduce the absorption of polyphenols from cocoa or black tea. The presence of milk proteins bound to flavonoids was shown to weaken the flavonoid antioxidant capacity in test tube study 154 and milk consumption has been shown to blunt the vascular benefits of tea flavonoids in healthy volunteers 155. Some carbohydrate-rich foods may increase the deglycosylation and absorption of flavonoids by stimulating gastrointestinal motility, mucosal blood flow, and colonic fermentation 50. Conversely, dietary flavonoids have been shown to interfere with carbohydrate digestion and absorption 50.
In general, the bioavailability of flavonoids is low due to limited absorption, extensive metabolism, and rapid excretion 50. Isoflavones are thought to be the most bioavailable of all flavonoid subclasses, while anthocyanins and galloylated catechins are very poorly absorbed 146, 156. Given the wide variability in structures within subclasses, it is difficult to generalize the absorbability and bioavailability of flavonoids based only on their structural classification. In addition, when evaluating the data from flavonoid research in cultured cells, it is important to consider whether the flavonoid concentrations and metabolites used are physiologically relevant 157. In humans, peak plasma concentrations of soy isoflavones and citrus flavanones have not been found to exceed 10 micromoles/liter (μM) after oral consumption. Peak plasma concentrations measured after the consumption of anthocyanins, flavan-3-ols, and flavonols (including those from tea) are generally lower than 1 μM 48. A recent quantitative analysis of 88 polyphenolic metabolites (not limited to flavonoids) identified in human blood and urine found median peak concentrations of 0.9 μM and 3.2 μM after food intake and oral supplementation, respectively 142.
Flavonoids health benefits
Research has shown that flavonoids have a range of health benefits, including antioxidant, anti-inflammatory and anti-cancer properties. They’ve been linked to a reduced risk of chronic diseases such as cardiovascular disease, as well as improved cognitive function.
Table 10. Flavonoids biological activity and mechanism of action
Flavonoids | Biological Activity | Mechanism of Action | Sources of Plant |
---|---|---|---|
Proanthocyanidins (anthocyanins) | antioxidant, anti-inflammatory, antibacterial, antifungal and anti-cardiovascular | polymerizing flavonoid monomer and Catechin polymer | grapes, apples, sorghum, cherries, black rice, black beans, purple potatoes, blueberries |
Fisetin | antioxidant activity | inhibiting the activities of oxidase | strawberry, apple, onion, cucumber, and other fruits and vegetables |
Hydroxyflavones | antioxidant activity | generating complexes with metal cations | fruits, vegetables, herbs, and other natural plants |
Kaempferol | antioxidant, anti-inflammatory, antibacterial, antiviral, and anticancer | chelate Cu(II) ions | Many vegetables, including onions, hot peppers, broccoli, snap beans, kale, and lettuce contain flavonol compounds quercetin and kaempferol |
Catechol | antioxidant activity | generating stable metallic complexes | fruits, vegetables, herbs, and other natural plants |
Quercetin | antioxidant activity anticardiovascular action | interacting with α-tocopherol radicals protecting the heart against ischemia-reperfusion injury | grapes, apples, sorghum, cherries, and other natural plant |
Epigallocatechin Gallate Naringin | antioxidant, antibacterial, antifungal, anti-cardiovascular, and antiviral | interacting with α-tocopherol radicals suppressing the biosynthesis of ATP | green tea, teas, cranberries, strawberries, blackberries, kiwis, cherries, pears, peaches, apples, avocados, pecans, pistachios, and hazelnuts |
Apigenin | antibacterial, antifungal, and antiviral | reducing the steady-state mRNA levels induced by TNF-α | parsley, celery, onions, grapes, apples, strawberries, oranges, chamomile tea and red wine |
Quercetin catechins | antiinflammatory action | reducing the expression of pro-inflammatory cytokines | red grapes, citrus fruit, raspberries, cranberries, tomato, broccoli and other leafy green vegetables |
Kaempferol Myricetin | antiinflammatory action | inhibit the activity of lipoxygenase | grapes, apples, sorghum, cherries, and other natural plant |
Diosmin Hesperidin | antiinflammatory action | inhibiting leukotriene B4 biosynthesis | citrus fruits |
Chalcones | anti-inflammatory and anticancer | inhibiting proinflammatory cytokines activity | apple, citrus, soybean, ginger, mulberry |
Vitexin Orientin Rutin | antioxidant, anti-inflammatory, and anticancer | inhibiting iNOS and COX2 expression | passion flower, Açaí palm, buckwheat sprouts,millets Chinese globeflower, pigeon pea, English hawthorn mistletoe fig, greater duckweed (Spirodela polyrhiza) |
Naringenin Hesperetin | anticardiovascular action | reducing high blood pressure by promoting vasodilation | grapefruits, sour orange, tart cherries, tomatoes and Greek oregano blood orange, mandarin juice, blond orange juice, lemon and lime |
Quercetin | anticardiovascular action | protecting the heart against ischemia-reperfusion injury via the cardioprotective effects of quercetin. | citrus fruits, apples, onions, parsley, sage, olive oil, grapes, dark cherries, dark berries such as blueberries, blackberries, and bilberries, tea, and red wine. |
Isoflavones | anticardiovascular action | inhibiting monocyte-endothelial cell adhesion | soybeans, chickpeas, fava beans, pistachios, peanuts, and other fruits and nuts |
Anthocyanins | anticardiovascular action | improving systolic blood pressure, and reduce the content of triglycerides, total cholesterol, and low-density lipoprotein cholesterol | grapes, apples, sorghum, cherries, and other natural plant |
Xanthohumol | anticardiovascular action | increasing PTEN expression and inhibited AKT/mTOR phosphorylation | common hop or hops (Humulus lupulus) |
Flavonoid | anticardiovascular action | modulating the PI3K/Akt/GSK3β pathway or downregulating the ROS-mediated JNK/p38MAPK/NFκB pathway | grapes, apples, sorghum, cherries, and other natural plant |
the citrus flavonoid naringenin | anticardiovascular action | enhancing SIRT1 expression, reduced ROS production | citrus fruits like grapefruits, sour orange, tart cherries, tomatoes, Greek oregano, bergamot, cocoa, water mint, Drynaria, as well as in beans |
Quercetin | antibacterial action | blocking the biofilm formation and suppressed the DNA replication | citrus fruits, apples, onions, parsley, sage, olive oil, grapes, dark cherries, and dark berries such as blueberries, blackberries, and bilberries, tea, and red wine |
Apigenin Naringenin Chrysin Genistein Kaempferol Daidzin | Antibacterial action | blocking the biofilm formation or regulate ROS species, decrease lipid peroxidation | parsley, celery, onions, grapes, apples, strawberries, oranges, chamomile tea and red wine citrus fruits like grapefruits, sour orange, tart cherries, tomatoes, Greek oregano, bergamot, cocoa, water mint, Drynaria, as well as in beans passion flowers, carrots, chamomile, fruits, and mushrooms Genistein is a polyphenol phytoestrogen found in legumes, such as beans, soy, lentils, and peas Many vegetables, including onions, hot peppers, broccoli, snap beans, kale, and lettuce contain flavonol compounds quercetin and kaempferol Soy and soy products are the primary dietary sources of isoflavones, which include daidzein, genistein and glycitein |
Baicalein Luteolin Myricetin | antibacterial action | suppressing the DNA replication or suppressed the biosynthesis of ATP | Chinese medicinal plant Scutellaria baicalensis, Welsh onion Vegetables and fruits such as celery, parsley, broccoli, onion leaves, carrots, peppers, cabbages, apple skins, and chrysanthemum flowers are luteolin rich Common dietary sources of myricetin include vegetables (including tomatoes), fruits (including oranges), nuts, berries, tea, and red wine. |
Epigallocatechin gallate | antibacterial action | suppressing the biosynthesis of ATP | Epigallocatechin gallate is a natural constituent predominantly found in green tea but also a broad variety of food, plants and herbs, including cranberries, strawberries, blackberries, kiwis, cherries, pears, peaches, apples, avocados, pecans, pistachios, and hazelnuts |
Glabrol | antibacterial action | increasing the permeability of the cell membrane and collapsing the proton motive force | Chinese liquorice (Glycyrrhiza uralensis) |
Glabridin | antifungal action | inhibiting the biosynthesis of the main components of fungi cell walls | Glabridin is a major flavonoid extracted from the root of licorice |
Myricetin Kaempferol Naringenin Genistein Luteolin | antifungal action | suppressing the biosynthesis of DNA, RNA, and protein | |
Glcyrrhiza Glabra Isoflavones Chalcone | antifungal action | regulating the expression level of phosphatidylserine decarboxylase | |
Apigenin | antiviral action | inhibiting viruses from entering the host cells, modulate the immune system, and reduce viral load | |
Baicalein | antiviral action | blocking the replication of the avian influenza H5N1 virus | |
Luteolin | antiviral action | inhibiting HIV-1 reactivation | |
Kaempferol | antiviral action | blocking the HIV-1 replication | |
Liquiritigenin | promoted apoptosis | increasing the p53 and Bax gene expression, decreasing Bcl-2 gene expression | |
Isoflflavone genistein | suppress the proliferation | promoting breast cancer cell arrest at the G2/M phase and subsequent ROS-dependent apoptosis | |
Kaempferol | suppress the proliferation | inducing apoptosis via cell cycle arrest MDA-MB-453 cells | |
Naringenin | inhibit the proliferation and migration | inhibiting ROS formation | |
Apigenin Luteolin | induce apoptosis | causing the alterations to ROS signaling |
Table 11. Flavonoids rich-foods against chronic diseases
Flavonoids rich-food | Biological and pharmacological effects |
---|---|
Fruits/vegetables | Anti-hypertension, reduced risk of diabetes, anti-hypercholesterolemia, anti-obesity, ↓ cardiovascular diseases, ↓ breast cancer, ↓ coronary heart diseases |
Whole grains | Reduced risk of diabetes, anticancer |
Coffee | Reduced risk of type 2 diabetes |
Berries | ↓Prostate cancer, reduced risk of type 2 diabetes |
Green tea | Reduced risk of type 2 diabetes, ↓ blood glucose, anticancer |
Apple | Reduced risk of type 2 diabetes, ↓ breast cancer, ↓ cardiovascular diseases |
Black tea | ↓Blood glucose, ↓ total and LDL cholesterol, ↓ myocardial infarction, reduced risk of coronary heart disease |
Onion | Antihyperglycemic effects, ↓ breast cancer |
Garlic | Anti-platelet aggregation, modification of LDL, antihyperglycemic effects, anticancer |
Cruciferous vegetables | Anticancer |
Cabbage | Anticancer, ↓ vascular diseases |
Broccoli | Anticancer, ↓ prostate cancer |
Cauliflower | Anticancer, ↓ prostate cancer |
Brussels sprouts | Anticancer |
Soy | Reduced risk of breast and prostate cancer |
Citrus fruits | Antiproliferative, ↓ vascular diseases |
Tomato | ↓ Prostate cancer |
Turmeric | Anti-hepatocarcinogenesis, anticancer |
Ginger | Inhibit platelet aggregation, anticancer, anti-thrombotic |
Carrots | Anticancer |
Footnote: ↓: decreased; LDL: low-density lipoprotein
[Source 46 ]Table 12. Summary of studies indicating reduced mortalities with intake of flavonoids rich-foods
Type of flavonoids rich-foods | Dose | Type of study | Number of participants | Follow-up period | Outcomes | References |
---|---|---|---|---|---|---|
Fruit and vegetable | ≥5 servings/day | Population-based cohort | 71706 | 13 year | 53 percent higher all-cause mortality rate in those who never consumed fruits and vegetables than those who consumed five servings/day | 158 |
Cruciferous vegetable | 144, 232, 307, 398 & 583 g/day | Prospective cohort study | 134796 | 4.6 year | A dose-response pattern was evident for increasing quintiles of cruciferous vegetables intake and reduction in total mortality as well as CVD mortality | 159 |
Fruit and vegetables | 178.8, 316.8, 468.4 & 725.4 g/day | Population cohort study | 451,151 | 10 year | A stronger association was observed for raw vegetable and fruit consumption and reduction in all-cause mortality as well as CVD mortality | 160 |
Coffee | 1 to >6 cups/day | Cohort study | 41, 836 | 15 year | 1-3 cups/d significantly reduced the mortality from CVD and other inflammatory diseases in postmenopausal women | 161 |
Green tea | 1 to >5 cups/day | A population-based, prospective cohort study | 40, 530 | 11 year | A 16% lowered mortality from all-cause and CVD in people who consumed 5 or more cups/day than those consuming less than 1 cup/day | 162 |
Fruit, vegetable, and beans | Fruit: 0.9, 2.3, 3.9 &5.9 servings/wk; Vegetable: 1.2, 2.3, 3.4 & 5.2 servings/wk; Bean: 0.8, 1.8, 3.0 & 4.5 servings/wk | Cohort study | 59485 | 13 year | Fruit intake followed by vegetable and bean intake exhibited the significant inverse association for total and CVD mortality | 163 |
Whole grains | ≥3 servings/ day | Cross-sectional study | 535 | 3 year | A significant inverse association was recorded for whole-grain intake and mortality from CVD | 164 |
Green-yellow vegetables and fruits | ≤ 1, 2-4 servings/wk and one serving daily | Prospective study | 38, 540 | 18 year | Subjects had 12% lower mortality from all cancers and 20% lower mortality from lung and stomach cancer; as well as 8% lower mortality from all cancers and 25% lower mortality from liver cancer, those consuming 1 or about 1 serving/day of fruits and green-yellow vegetables, respectively, comparing with those who ate these foods once per week or less. | 165 |
Fruit and vegetables | 0.87, 1.61, 2.31, 3.21 & 4.89 servings/day | Prospective study | 6151 | 13 year | Compared with the bottom fifth, highest fifth of fruit and vegetable consumers had a significantly lower all-cause, cancer, and CVD mortality | 166 |
Fruit and vegetables | < 5 to ≥5 servings/d | Cohort study | 501 | 18 year | Individuals consumed the combination of ≥5 servings/day of fruits and vegetables and ≤12 energy from saturated fat had lower all-cause (31%) and CVD (76%) mortality, compared with those consuming <5 servings/day of fruits and vegetables and >12% saturated fats | 167 |
Fruit and vegetables | <1 to 8 times/day | Cohort study | 9608 | 19 year | Fruit and vegetable consumption ≥3 times/day as compared with <1 time/day significantly reduce stroke mortality (42%), ischemic heart disease mortality (24%), CVD mortality (27%), and all-cause mortality (15%) | 168 |
Fruits | 0-1, 2-3, 4-5, 6-7 times/wk | Prospective cohort study | 792 | 26 year | Significantly lower total and CVD mortality were recorded in men with high fruit consumption. This association existed up to 16 year follow-up (at the age of 70) | 169 |
Fruit, berry, and vegetables | <133, 133-214, 215-293, 294-408 &>408 g/day | Prospective cohort study | 2641 | 12.8 year | A significantly lower CVD, non-CVD, and all-cause mortality was observed in men with the highest consumption of fruit, vegetable, and berries | 170 |
Antioxidant activity
Antioxidant is any substance that prevents or reduces damage caused by reactive oxygen species (ROS) or reactive nitrogen species (RNS). Antioxidants help protect your body from damage to cells, protein, and even DNA that occurs as a result of stress and free radicals. Reactive oxygen species (ROS) are produced in the human body mainly as byproducts of the electron transport chain. Reactive oxygen species (ROS) are essential for protein phosphorylation, initiation of numerous transcriptional factors, apoptosis, immunity, and differentiation processes. However, reactive oxygen species (ROS) also cause oxidative stress upon reacting with molecules such as fats, proteins, or nucleic acids. Fat peroxidation by reactive oxygen species (ROS) causes cellular membrane damage. This membrane has a potential, with positive charges on the outside of the cell, and negative charges inside the cell. The damage to membrane alters the cell membrane potential and the cell’sosmotic pressure, eventually causing cell death. The human defense system use different mechanisms and enzymes to battle endogenous elevated reactive oxygen species (ROS) 171. Flavonoids are effective scavengers of free radicals in the test tube (in vitro) 172, 173. Flavonoids act as antioxidants and are directly oxidized by radicals to form less reactive species via four mechanisms, namely (1) the inhibition of nitric-oxide synthase activity, (2) inhibition of xanthine oxidase activity, (3) modulation of channel pathways, or by (4) interacting with other enzyme systems 174, 175. However, even with very high flavonoid intakes, plasma and intracellular flavonoid concentrations in humans are likely to be 100 to 1,000 times lower than concentrations of other antioxidants, such as vitamin C (ascorbic acid), uric acid, and glutathione 50. Most circulating flavonoids are actually flavonoid metabolites, some of which have lower antioxidant activity than the parent flavonoid 143. For these reasons, the relative contribution of dietary flavonoids to plasma and tissue antioxidant function in the body is likely to be very small or negligible 176, 177, 178.
Figure 7. Flavonoid antioxidant activity
Footnotes: Flavonoid antioxidant activity in test tube. (a) Direct scavenging of reactive oxygen species (ROS); (b) Activation of antioxidant enzymes; (c) Activation of metal-chelating activity; (d) Increasing alpha-tocopheryl radical levels; (e) Inhibiting NAPDH oxidases; (f) Mitigation of oxidative stress caused by nitric oxide (NO); (g) Increasing uric acid levels; (h) Increasing antioxidant properties of low-molecular-weight antioxidants.
[Source 45 ]Metal chelation
Metal ions, such as iron and copper, can catalyze the production of free radicals. The ability of flavonoids to bind (chelate) metal ions appears to contribute to their antioxidant activity in test tube study 179, 180, 181. The chelation takes place via hydroxyl groups or their carbonyl moiety, if present 182. In living organisms, most iron and copper are bound to proteins, limiting their participation in reactions that produce free radicals. Although the metal-chelating activities of flavonoids may be beneficial in pathological conditions of iron or copper excess, it is not known whether flavonoids or their metabolites function as effective metal chelators in the body 176.
Flavonoids can bind nonheme iron, inhibiting its intestinal absorption 183, 184. Nonheme iron is the principal form of iron in plant foods, dairy products, and iron supplements. The consumption of one cup of tea or cocoa with a meal has been found to decrease the absorption of nonheme iron in that meal by about 70% 185, 186. Flavonoids can also inhibit intestinal heme iron absorption 187. Interestingly, vitamin C (ascorbic acid) greatly enhances the absorption of iron and is able to counteract the inhibitory effect of flavonoids on nonheme and heme iron absorption 184, 187, 188. To maximize iron absorption from a meal or iron supplements, flavonoid-rich food and beverages and flavonoid supplements should not be consumed at the same time.
Skin health
The ability of flavonoids to absorb ultraviolet (UV) light and modulate signaling pathways that influence cellular function appears to underlie flavonoids beneficial effects in skin health. Exposure to UV-radiation (UVR) has many negative effects on skin, including redness, swelling, sunburned cells, hyperplasia, inflammation, immunosuppression, photoaging, and initiation of cancer by UV light 189. Studies performed in cell culture, animals, and humans demonstrate that treatment with certain flavonoids can minimize adverse skin reactions caused by UV-radiation. Skin application of certain flavonoids may protect skin by absorbing UV-radiation before it can interact with and damage cellular components, thereby providing a sunscreen effect. Topically applied flavonoids may protect skin by absorbing UV light and blocking UV penetration. Pycnogenol (a registered mixture of naturally occurring mono- and oligomeric procyanidins) and honeybush extract (containing the flavanone hesperidin and xanthone mangiferin) absorb light in the UV-B range 190, 191. Topical application of these flavonoids would function as sunscreens when applied prior to UV exposure.
The majority of information on flavonoids and skin health relates to prevention against damaging effects of intense solar radiation of green tea polyphenols, catechins, and genistein. Both oral supplementation and skin administration of the flavanol subclass in particular have demonstrated photoprotective effects in humans 192. Experimentation with topically applied flavonoids typically test purified compounds or concentrated plant extracts dissolved in organic solvent; although they show promise as photoprotective agents, delivery is an issue that can influence how commercially available formulations penetrate and function in human skin 192. Flavonoids exert a wide range of influence due to their specific and nonspecific affinity for a diversity of proteins throughout the cell. The precise mechanisms by which flavonoids protect skin from the damaging effects of UV-radiation are still being investigated, but there is evidence that flavonoids physically block UV penetration, influence DNA repair, reduce oxidative damage, attenuate the inflammatory response, preserve immune function, and induce cytoprotective pathways 192.
Human epidermal keratinocytes express specific members of the organic anion transporting polypeptides (OATP) family, transporters responsible for the uptake of a variety of xenobiotics (chemical compounds that are foreign to human), drugs, and large amphipathic molecules 193. It is hypothesized that flavonoids enter epidermal cells via this route. Once inside the cell, flavonoids can bind catalytic ATP-binding sites on a diversity of proteins, thus exerting influence on a wide range of cellular processes 194, 177. For example, genistein and quercetin are known to inhibit tyrosine kinase and PI3 kinase, respectively 177. Specific interactions between certain flavonoids and cellular proteins also occur. Soy isoflavones for example bind to estrogen receptor-β (ER-β), the estrogen receptor isoform expressed in skin cells and the cardiovascular system 195, 196.
The timing of flavonoid administration dictates if the intervention is being used as a preventive or treatment. The majority of information reports on flavonoid administration prior to UV exposure, as a means to prevent UV-induced photodamage. However, flavonoid administration following UV exposure has been evaluated for several flavonoids. Genistein or epigallocatechin gallate (EGCG) dissolved in acetone was applied to hairless mouse skin one or four hours post-irradiation (2X MED), and 24 hours later, epidermal sections were collected and analyzed 197. Both flavonoids decreased the number of sunburn cells, epidermal hyperplasia, and immune suppression even when applied subsequent to UV exposure. Widyarini et al. 198 applied isoflavone extracts (20 μM) from red clover (Trifolium pretense) to the skin of hairless mice immediately following UV exposure in order to evaluate their ability to protect against acute effects induced by UV-radiation. Genistein and the isoflavone metabolites equol, isoequol, and dehydroequol significantly reduced inflammation, swelling, and immunosuppression caused by UV exposure. In a randomized, double-blind, placebo-controlled trial, Casetti et. al. 199 compared a luteolin-rich Reseda extract to hydrocortisone, a standard anti-inflammatory agent, for its efficacy following UV exposure. Forty healthy volunteers (both sexes, 18 years of age and older) were exposed to UVB (1.5X MED) followed by immediate application of a topical nanoparticle formulation of Reseda extract (2.5%), hydrocortisone (0.1%), or vehicle (glycerol). Compared to vehicle, both Reseda extract and hydrocortisone significantly reduced UVB-induced erythema to a similar extent 199.
The benefit of suppressing the sunburn response in order to minimize skin damage is a subject of debate. Preventing sun damage in the first place is advised as primary protection against the damaging effects of UV-radiation.
Green tea polyphenols
Heinrich et.al. 200 conducted a 12-week, placebo-controlled trial in 60 healthy women (40-65 years of age) to evaluate the effect of dietary green tea polyphenol consumption on skin photoprotection (protection against ultraviolet (UV) radiation-induced skin damage and skin cancers), structure, and function. Subjects consumed 1 liter of beverage throughout the day, containing 1,402 mg of green tea catechins or inert ingredients matched for additives and flavor 200. Redness formation in response to 1.25X MED, skin elasticity and structure (roughness, scaling, volume, and wrinkles), transepidermal water loss (TEWL), cutaneous blood flow, and serum flavonoid concentration were measured at 0, 6, and 12 weeks of treatment. Serum levels of the green tea polyphenols epigallocatechin gallate (EGCG), epicatechin gallate (ECG), and epicatechin (EC) significantly increased in the treatment group at both six and 12 weeks of intake 200. Additionally, all skin variables measured were significantly improved in those consuming green tea beverage compared to placebo at both time points 200.
Recognizing the challenges of consuming 1 liter of beverage over the course of a day, Heinrich et.al. 200 performed a small dosing study to assess the effects of green tea extract ingested in capsule form. Fifteen healthy women received placebo or 0.5, 1, or 2 g of encapsulated green tea extract 200. Serum flavonoid content and capillary blood flow to the dermis were measured over the course of four hours in order to assess the short-term effects of a single dose of encapsulated green tea extract. Dermal microcirculation was measured since increased cutaneous blood flow may contribute to enhanced delivery of oxygen and nutrients to the skin, a proposed impact of flavonoids on skin health.
There was an equivalent quick and brief increase in dermal microcirculation (15-30 minutes) at all doses of green tea extract ingested compared to placebo. Serum epicatechin levels increased in a dose-dependent manner, with a maximum concentration two hours post-ingestion.
Ingestion of high-flavanol cocoa powder, rich in epicatechin (EC) and catechin, for 12 weeks also improved photoprotection and skin structure in healthy female subjects 201. In this double-blind intervention, 24 female volunteers (18-25 years of age) were randomly assigned to ingest a high (326 mg) or low (27 mg) flavanol-containing cocoa beverage daily for 12 weeks 201. As with green tea beverage, high-flavanol cocoa powder diminished UV-induced redness formation, increased microcirculation, improved skin structure (as roughness, scaling, volume, and wrinkles), and reduced transepidermal water loss (TEWL); none of these parameters changed in the low-flavanol group 201. Additionally, a single dose of high-flavanol (329 mg) cocoa beverage quickly and transiently increased plasma epicatechin levels and dermal microcirculation compared to low-flavanol cocoa beverage 202. The effects of catechins on skin structure, texture, and water homeostasis may be due to their ability to increase cutaneous blood flow 202, 200, 201. Vasodilatory mechanisms, which have also been shown for cocoa polyphenols in non-skin vessels, may underlie the vascular benefits associated with flavanols 203, 204.
Katiyar et al. 205, 206, 207 studied the effects of topically applied green tea extract on several UV-mediated skin responses in humans. In each study, four to six volunteers (both male and female, aged 25 to 55 years old) received a topical application of purified green tea extract (1 mg/cm² dissolved in acetone to a sun-protected skin) containing a mixture of the four major polyphenols in green tea: epigallocatechin-3-gallate (EGCG), epicatechin (EC), epigallocatechin (EGC), and epicatechin-3-gallate (ECG). Twenty or 30 minutes after topical application, skin sites were exposed to UVB (4X MED). Twenty-four or 48 hours later, punch biopsies (i.e., both epidermis and dermis) were collected and various endpoints measured. In each case, pretreatment with purified green tea polyphenols inhibited UV-induced inflammation 206, DNA damage 207 and formation of reactive oxygen species (ROS) 205 compared to vehicle-treated sites on the same individual.
In a similar study design, Elmets et. al. 208 also observed a protective effect of topically applied purified green tea extract on UV-induced photodamage, assessed as erythema formation, presence of sunburn cells, DNA damage, and number of Langerhans cells, a marker of immunosuppression. A 5% solution that contained a mixture of the four major green tea polyphenols (0.5 g of purified extract dissolved in ethanol/water) was the most effective at minimizing UV-damage compared to vehicle-treated sites in the same subjects. Epigallocatechin gallate (EGCG) and epicatechin gallate (ECG) were also protective, although to a lesser extent than the mixed extract.
Camouse et. al. 209 performed a double-blind treatment in ten volunteers to compare topical green tea and white tea extracts as photoprotective agents. Pretreatment with either tea extract (2.5 mg/cm² in organic solvent) protected against depletion of Langherhans cells and oxidative DNA damage caused by UVB exposure (2X MED) compared to vehicle-treated sites on the same individual.
UV damages DNA by causing strand breaks or creating cyclobutane pyrimidine dimers (CPDs), a photoproduct formed when energy derived from UV-radiation is absorbed by DNA, forming an unwanted covalent bond between pyrimidine bases. A cell can either repair the damage or sacrifice itself (apoptosis) as a way to protect the organism from mutations and malignant transformation. The mechanism by which green tea polyphenols combat UV-induced cellular damage appears to be due primarily to their induction of DNA repair pathways in the skin 207, 208, 210 and influence on certain immune mediators known as cytokines 211, 212, 213, 214, 206. Because DNA damage initiates immunosuppression, a risk factor for skin cancer, green tea polyphenols appear to function early in the UV-damage response in the skin.
Genistein
The photoprotective effect of genistein has been investigated in animals and in test tube models of human skin. Pretreatment with topical genistein (5 µM, 60 minutes prior to UV exposure) reduced skin roughness and wrinkling and epidermal hyperproliferation in hairless mice that were exposed to daily doses of acute and chronic UVB irradiation 215. As observed with epigallocatechin gallate (EGCG), the photoprotective effects of genistein may result from its impact on UV-induced DNA damage as topical genistein decreased CPD formation and restored proliferating cell nuclear antigen expression, a marker of proliferation and DNA repair 215. The authors performed a small study in six men to extend their observations to humans: topical genistein (5 μM/cm²) applied 30 minutes before UVB exposure (1X MED) blocked erythema formation as evaluated photographically 24 hours after treatment 215. Moreover, pretreatment with topical genistein dose-dependently reduced cyclobutane pyrimidine dimer (CPD) formation and increased proliferating cell nuclear antigen (PCNA) expression in human reconstituted skin samples 216.
Other flavonoids
Silymarin is a special type of flavonoid classified as a flavonolignan, part flavonoid and part lignan. Silymarin is present in the seeds of milk thistle (Silybum marianum), and its major bioactive flavonoid is called silibinin. Like green tea polyphenols, topical silymarin minimizes UV-induced photodamage and photocarcinogenesis in animal studies 217. Experiments using primary cultures of normal human epidermal keratinocytes (NHEKs) and transgenic mice indicate that topical silymarin inhibits UV-induced apoptosis and reduces cyclobutane pyrimidine dimer (CPD) formation in the skin 218. By using cells and animals deficient in nucleotide excision repair, the authors further demonstrated that topical silymarin contributes to photoprotection by upregulating DNA repair processes 218.
Wound healing
Onion extract, rich in the flavonoids quercetin and kaempferol, has been used to reduce scar formation, particularly keloid scars. Cho et. al. 219 demonstrated that onion extract and quercetin induce matrix metalloproteinase-1 (MMP-1) expression in cultured human skin fibroblasts and hairless mouse skin. Matrix metalloproteinases (MMPs) are enzymes secreted by epidermal keratinocytes and dermal fibroblasts in response to various stimuli, including UV radiation, oxidative stress, and inflammatory cytokines. UV radiation induces three matrix metalloproteinases: MMP-1 (collagenase), MMP-3, (stromelysin), and MMP-9 (gelatinase) that cleave and degrade skin collagen and contribute to photoaging 220. In the case of wound healing, a balance between MMP-1 (collagenase) and tissue inhibitor matrix metalloproteinase-1 (TIMP-1) enzymatic activity affects the amount of extracellular matrix (including collagen) formed at the wound site. Therefore, quercetin may influence extracellular matrix deposition during wound healing in order to reduce hypertrophic scarring.
Cardiovascular disease prevention
Flavonoids have been shown to (1) reduce inflammation by suppressing the expression of pro-inflammatory mediators; (2) down-regulate the expression of vascular cell adhesion molecules, which contribute to the recruitment of inflammatory white blood cells from the blood to the arterial wall; (3) increase the production of nitric oxide (NO) by endothelial nitric oxide synthase (eNOS), thus improving vascular endothelial function; (4) inhibit angiotensin-converting enzyme, thus inducing vascular relaxation; (5) inhibit platelet aggregation; and (6) oppose smooth muscle cell proliferation and migration occurring during atherogenesis 221, 222, 223, 224, 225, 226, 227, 228, 229.
Several prospective cohort studies conducted in the US and Europe have examined the relationship between some measure of dietary flavonoid intake and cardiovascular disease (CVD) or mortality. A recent meta-analysis of 14 prospective studies published between 1996 and 2012 reported that higher intakes in each flavonoid subclass were significantly associated with a reduced risk of cardiovascular events 230. Top versus bottom quantiles of intake for each of the flavonoid subclasses were associated with an approximate 10% reduction in the risk of cardiovascular disease. Another meta-analysis of eight prospective studies found a 14% reduced risk of stroke with the highest versus lowest quintile of flavonol intakes 231. However, several serious limitations highlighted in a recent publication by Jacques et al. 232 suggested caution when interpreting these results. In particular, most of the prospective studies in these meta-analyses did not include all flavonoid subclasses nor calculate intakes using the latest and more complete versions of the USDA databases for the flavonoid content of foods 50. Another major concern is the lack of adjustment regarding the overall quality of the diet. Consumers with higher flavonoid intakes are likely to have a greater consumption of fruit and vegetables and overall healthier diets than those with poor flavonoid intakes. Additionally, none of the studies excluded potential bias due to constituents of flavonoid-rich foods that are known to either lower (e.g., other phytochemicals, vitamins, dietary fiber) or increase (e.g., sodium, saturated fat) the risk of cardiovascular events 232.
In the Framingham Offspring Cohort study that followed 2,880 adults for a mean of 14.9 years, consumption of all flavonoid subclasses except flavones and flavanones was inversely associated with cardiovascular disease 232. Yet, adjusting for confounding factors, including fruit and vegetable intake and overall diet quality, reduced these relationships such that they were no longer statistically significant. An analysis of a larger prospective study of the EPIC-Norfolk cohort (24,885 participants) that considered confounding by many dietary factors (vitamin C, dietary fiber, fat, saturated fat, potassium, sodium, and alcohol) found no significant association between flavan-3-ol intake and cardiovascular disease-related or all-cause mortality 233.
A number of large prospective studies and small-scale, randomized controlled trials have investigated the effects of flavonoids on established biomarkers of cardiovascular disease, including those involved in oxidative stress, inflammation, abnormal blood lipid profile, endothelial dysfunction, and hypertension.
Anti-hypertensive effect
A meta-analysis of 20 short-term, randomized controlled trials, including a total of 856 mainly healthy participants, found that consumption of flavan-3-ol-rich dark chocolate and cocoa products significantly reduced systolic blood pressure by 2.77 mm Hg and diastolic blood pressure by 2.20 mm Hg. However, heterogeneity across studies was high, and risk of bias was significant 234. A greater blood pressure-reducing effect was observed in a subanalysis of studies using flavan-3-ol-free rather than flavan-3-ol-low control groups 234. Another meta-analysis of 22 trials (highly heterogeneous) found reductions in diastolic blood pressure (-1.60 mm Hg) and mean arterial pressure (-1.64 mm Hg) with chocolate or cocoa intake but no change in systolic blood pressure 235. Additionally, green tea flavan-3-ols have been shown to lower blood pressure especially in (pre-) hypertensive subjects. A pooled analysis of 13 randomized controlled trials in 1,040 subjects found a 2.05 mm Hg reduction in systolic blood pressure and a 1.71 mm Hg reduction in diastolic blood pressure with green tea consumption for at least three weeks 236. The inhibition of angiotensin-converting enzyme (ACE), a key regulator of arterial blood pressure, may partly explain how flavan-3-ol-rich food and beverages might exert blood pressure-lowering effects 237.
Some intervention trials have also examined the effect of the flavonol quercetin on blood pressure in human subjects. In a randomized, double-blind, cross-over, placebo-controlled trial in 96 participants diagnosed with metabolic disorders, supplementation with 150 mg/day of quercetin aglycone significantly reduced systolic blood pressure by 2.6 mm Hg without affecting diastolic blood pressure and other cardiometabolic markers 131. Similar results were found with 730 mg/day of quercetin in hypertensive individuals 238 and with 500 mg/day of quercetin in women with type 2 diabetes mellitus 239. In a recent six-week, cross-over, randomized, double-blind, placebo-controlled trial, daily ingestion of 162 mg of quercetin decreased 24 h-ambulatory blood pressure — but not systolic blood pressure in the resting state — in hypertensive but not in pre-hypertensive participants 240. There was no change in biomarkers of lipid metabolism, inflammation, oxidative stress, or endothelial function, including total, HDL-cholesterol (high-density lipoprotein or “good” cholesterol), LDL-cholesterol (low-density lipoprotein or “bad” cholesterol), serum C-reactive protein (CRP), soluble adhesion molecules, plasma oxidized LDL, urinary 8-isoprostaglandin F2α, serum endothelin-1, serum ACE, and plasma endogenous NOS inhibitor 240.
Additional trials may help establish whether the blood pressure-lowering effect of some flavonoids could be translated into long-term benefits for cardiovascular health.
Biochemical markers of cardiovascular disease
In a cross-sectional analysis of the Framingham Offspring Cohort study, the highest versus lowest intake of anthocyanins (≤3.5 mg/day versus ≥23.5 mg/day) was associated with lower concentrations of acute-phase reactant proteins (-100%), pro-inflammatory cytokines (-75%), and markers of oxidative stress (-52%), even after adjustment for confounding variables 241. Interestingly, a food-based analysis revealed that intakes of foods rich in anthocyanins, e.g., apples, red wine, and strawberries, were also inversely associated with an overall inflammation score based on 12 different biomarkers. Higher intakes of polymeric flavan-3-ols (i.e., theaflavins, thearubigins, and proanthocyanidins) were correlated with lower concentrations of pro-inflammatory cytokines and biomarkers of oxidative stress. Intake levels of total flavonoids and flavan-3-ol monomers (i.e., catechins) were inversely associated with concentrations of the biomarkers of oxidative stress. Although tea is a major source of flavan-3-ols, tea consumption was not correlated with the composite inflammation score or any components of this score in this study 241.
Cocoa is another source of flavan-3-ols, in particular (-)-epicatechin and procyanidins, that may provide cardiovascular benefits 242. A recent randomized, double-blind, placebo-controlled study in 100 healthy adults (ages, 35-60 years) suggested that short-term benefits of cocoa flavan-3-ol consumption on cardiovascular health, including improvements in lipoprotein profile (i.e., higher HDL-cholesterol and lower total and LDL-cholesterol) and blood pressure, could be extrapolated to predict a 20%-30% reduced 10-year risk of cardiovascular disease and cardiovascular disease-related mortality 243.
An increasing number of trials in which participants were fed with berries 244, 245, 246 or juices 247 rich in anthocyanins or with purified anthocyanins 248 also reported reduced levels of inflammatory markers and/or improved antioxidant status, decreased LDL-cholesterol (low-density lipoprotein or “bad” cholesterol), improved insulin sensitivity, and lowered blood pressure 249. In a randomized, double-blind, placebo-controlled study in 150 individuals with hypercholesterolemia, supplementation with a purified anthocyanin mixture (320 mg/day) for 24 weeks reduced circulating markers of inflammation, including C-reactive protein (CRP), interleukin-1β (IL-1β), and soluble vascular adhesion molecule-1 (sVCAM-1) 250. Supplementation of dyslipidemic patients for 12 or 24 weeks with a mixture of 17 anthocyanins improved cholesterol clearance via the HDL-mediated reverse cholesterol transport from extra-hepatic tissues back to the liver and lowered LDL-cholesterol compared to a placebo in two randomized controlled trials 251, 252. However, a 12-week, randomized, double-blind, placebo-controlled study in 52 healthy postmenopausal women found that daily consumption of 500 mg of elderberry anthocyanins (as cyanidin-3-glucoside) had no effect on inflammation markers, markers of vascular health, lipid profile, and glycemia; all of these measures were in normal range of concentrations at baseline 253. Whether exposure to high-dose anthocyanins could lower the risk of cardiovascular disease in subjects with established cardiovascular disease risk factors and/or help maintain cardiovascular health in apparently healthy individuals remains to be confirmed.
Endothelial dysfunction
The vascular endothelial cells that line the inner surface of all blood vessels synthesize an enzyme, endothelial nitric oxide synthase (eNOS), whose function is essential to normal vascular physiology. Specifically, endothelial nitric oxide synthase (eNOS) produces nitric oxide (NO), a compound that regulates vascular tone and blood flow by promoting the relaxation (vasodilation) of all types of blood vessels, including arteries 254. Nitric oxide (NO) also regulates vascular homeostasis and protects the integrity of the endothelium by inhibiting vascular inflammation, leukocyte adhesion, platelet adhesion and aggregation, and proliferation of vascular smooth muscle cells 255. In the presence of cardiovascular risk factors (e.g., hypertension, hypercholesterolemia, hyperglycemia), early alterations in the structure and function of the vascular endothelium are associated with the loss of normal nitric oxide (NO)-mediated endothelium-dependent vasodilation. Endothelial dysfunction results in widespread vasoconstriction and coagulation abnormalities and is considered to be an early step in the development of atherosclerosis. Measures of brachial flow-mediated dilation (FMD), a surrogate marker of endothelial function, have been found to be inversely associated with risk of future cardiovascular events 256.
Preclinical studies have demonstrated the benefits of berry fruits, extracts, or purified anthocyanins on vascular function. Anthocyanin supplementation to diabetic mice was found to improve diabetes-induced vascular dysfunction by promoting nitric oxide (NO)-mediated endothelium-dependent vasodilation through the upregulation of adipocyte-derived adiponectin 257. Supplementation with purified anthocyanins (320 mg/day for 12 weeks) also increased serum adiponectin concentrations and improved FMD in 58 individuals with type 2 diabetes 257. In a randomized trial of 150 participants with hypercholesterolemia, supplemental anthocyanins increased flow-mediated dilation values by 28.4% compared to 2.2% in the placebo group 258.
Several small-scale, intervention studies have also examined the effect of flavan-3-ol-rich food and beverages, including tea, red wine, purple grape juice, cocoa, and chocolate, on endothelium-dependent vasodilation. A meta-analysis of nine intervention studies in a total of 213 participants estimated that the acute ingestion of 2 to 3 cups of tea (500 mL) — containing about 248 mg of flavonoids in green tea and 415 mg in black tea — significantly increased brachial flow-mediated dilation 259. Another meta-analysis of 18 randomized controlled studies found that acute (2 hour post-ingestion) and chronic (≤18 months) consumption of flavan-3-ol-rich cocoa beverages and chocolate bars significantly increased flow-mediated dilation in participants 235. A small 15-day, cross-over intervention study in hypertensive individuals with endothelial dysfunction found that 100 g/day of flavan-3-ol-rich dark chocolate, but not 90 g/day of flavan-3-ol-free white chocolate, could restore flow-mediated dilation values almost to normal levels 260. Also, using a similar protocol, the authors showed that dark chocolate intake blunted acute endothelial dysfunction-induced by a glucose load challenge in 12 healthy volunteers 261. Other benefits of dark chocolate consumption included reductions in arterial stiffness (measured through pulse wave analysis) and serum concentrations of markers of oxidative stress and vasoconstriction (8-isoprostaglandin F2α and endothelin-1). A randomized controlled trial in overweight and obese participants also reported that the daily consumption of a high-flavan-3-ol cocoa drink (902 mg/day of flavan-3-ols), but not that of a cocoa drink low in flavan-3-ols (38 mg/day), resulted in a sustained increase in flow-mediated dilation during the 12-week study 262. A more recent four-week, randomized, double-blind, cross-over, controlled study in healthy overweight or obese adults found that the consumption of 22 g/day of natural cocoa (in the form of dark chocolate bar and cocoa drink; 814 mg/day of flavan-3-ols) increased arterial diameter and blood flow and lowered peripheral arterial stiffness, but there was no change in flow-mediated dilation 263. Another recent clinical trial found improvements in endothelium-dependent vasodilation in response to acute consumption of one bar (40 g) of dark chocolate (containing 10.8 mg of (+)-catechins and 36 mg of (-)-epicatechins) and daily consumption of two bars (80 g) for up to four weeks in 20 individuals with chronic heart failure 264. Oral administration of pure flavan-3-ol (-)-epicatechin to healthy volunteers showed nitric oxide (NO)-dependent vasodilatory effects similar to those observed following flavan-3-ol-rich cocoa ingestion 203. Administration of (-)-epicatechin also improved acetylcholine-induced endothelial-dependent vasodilation of thoracic aorta rings from rats with salt-induced hypertension 265.
Endothelial nitric oxide (NO) production also inhibits the adhesion and aggregation of platelets, one of the first steps in atherosclerosis and blood clot formation 255. A number of clinical trials that examined the potential for high flavonoid intakes to decrease various measures of platelet function outside of the body (ex vivo) have reported mixed results. A recent systematic review of these intervention studies suggested that consumption of flavan-3-ol-rich cocoa and grape seed extract was generally found to improve platelet function by inhibiting platelet adhesion, activation, and aggregation 266. Interestingly, in a cross-over, controlled study, the acute consumption of a flavan-3-ol-rich cocoa beverage (897 mg of total (-)-epicatechin and procyanidins) exhibited additive anti-platelet effects to aspirin (81 mg) in healthy volunteers 267. In contrast, the results of interventions using apigenin-rich soup, quercetin-rich supplements or onion soups, isoflavone-rich soy protein isolates, black tea, wines, berries, or grape juices have given inconsistent results 266.
Diabetes prevention
Diabetes is an epidemic health concern in both developed and developing countries, characterized by impaired insulin production and insulin resistance. In the United States, 7.8% of the population have diabetes, 35.4% have impaired fasting glucose (IFG) levels, 15.4% have impaired glucose tolerance (IGT), and 40.1% have pre-diabetes (impaired fasting glucose, impaired glucose tolerance, or both) 268, while in China 9.7% and 15.5% of people are positive for diabetes and pre-diabetes, respectively 269. Diabetes could reduce life expectancy up to 15 years and account as a significant cause of mortalities in both developed and developing countries 270. Diabetes is also associated with other chronic diseases like stroke, heart diseases, nervous system disorders, kidney diseases, and vision problems. Its prevention is more accessible than a cure. In randomized trials, it was consistently observed that increased physical activity, weight loss and healthy diet are the ideal approaches to prevent diabetes 271.
Numerous physiological studies had found that free radicals might contribute to the autoimmune destruction of pancreatic beta cells, leading to diabetes 272, and may impair insulin action 273. Fruits, vegetables, and whole grains possess strong scavenging ability against these radicals resulting in reduced risk of type 1 diabetes and type 2 diabetes 274, 275. It was also hypothesized that plant-derived foods like fruits and vegetables are low in carbohydrate contents, therefore could prevent the rise of blood sugars 271. Other dietary factors that have been related to reducing the risk of type 2 diabetes include coffee 276, berries 275 and tea 277. High intakes of quercetin and myricetin, mainly from dietary consumption of apples and berries also associated with reduced risk of type 2 diabetes 278. Among European adult persons who drank coffee frequently (≥7 cups/day) had a 29% to 52% reduced risk for diabetes compared with those who drank less coffee (≤2 cups/day or no cups/day) 276, 279. Moreover, in another study in Japan, it was reported that consumption of green tea, coffee, and large total caffeine was associated with a reduced risk for type 2 diabetes 280. Furthermore, antidiabetic (blood glucose reducing) effect of green tea 281 and black tea 282 has also been observed, indicating them as potent preventive and curative agents. Onion and garlic also have been found to have antihyperglycemic effects 283, probably based on their phytochemical contents.
Flavonoids have been found to interfere with the digestion, absorption, and metabolism of carbohydrates 284. Each subclass of flavonoids has also demonstrated anti-diabetic properties, including (1) improving insulin secretion and viability of pancreatic beta-cells under glucotoxic or pro-inflammatory conditions, (2) increasing insulin-stimulated glucose uptake by target cells, (3) protecting muscle cells against fatty acid-induced insulin resistance, and (4) reducing hyperglycemia and improving glucose tolerance in animal models of obesity and/or type 2 diabetes mellitus 285.
The association between flavonoid consumption and risk for type 2 diabetes mellitus has been examined in a recent European, multicenter, nested case-control study — the “EPIC-InterAct” project — that included 16,835 diabetes-free participants and 12,043 diabetics. In this study, participants in the highest quintile of total flavonoid intake (>608.1 mg/day) had a 10% lower risk of diabetes than those in the lowest quintile (<178.2 mg/day) 286. The risk of diabetes was inversely correlated with the intake of flavan-3-ols (monomers and dimers only) and flavonols 286, 287. Recent meta-analyses of randomized controlled trials have examined the possible health effects of green tea flavan-3-ol monomers (catechins) on glucose metabolism and have provided conflicting results. A meta-analysis of seven trials in pre-diabetic and diabetic patients found no effect of green tea or green tea extracts on fasting plasma glucose, fasting serum insulin, or measures of glycemic control (glycated hemoglobin, HbA1c) and insulin sensitivity (HOMA-IR) 288. Conversely, another meta-analysis of 17 trials in pre-diabetic, diabetic, or overweight/obese subjects found that administration of green tea extracts for 4 to 16 weeks improved fasting plasma glucose and HbA1c level 289. The effect on fasting glucose was observed only with high doses of catechins (≥457 mg/day) and when the confounding effect of caffeine was removed. A third meta-analysis of 25 trials found that ingestion of green tea extracts for at least two weeks could lower fasting blood glucose in both the presence or absence of caffeine 290.
Dark chocolate is another good source of flavan-3-ols such that the effects of cocoa flavan-3-ols have been examined in individuals at-risk or with established type 2 diabetes. In a 15-day, cross-over, randomized controlled study, the daily consumption of 100 g of dark chocolate bars containing 110.9 mg of (-)-epicatechin and 36.1 mg of (+)-catechin significantly improved measures of pancreatic beta-cell function and insulin sensitivity, along with cardiometabolic markers in glucose-intolerant and hypertensive subjects 291. Daily supplementation with flavonoid-enriched chocolate containing 850 mg of flavan-3-ols and 100 mg of isoflavones for one year significantly improved insulin sensitivity and reduced a predicted risk of coronary heart disease at 10 years in 93 postmenopausal women treated for type 2 diabetes 292.
The EPIC-InterAct study did not find any association between dietary anthocyanin intake and risk of diabetes 286, 287. Yet, a 10-fold increase in anthocyanin consumption was correlated with a 15% lower risk of diabetes in the pooled analysis of three large US prospective cohorts (120,003 participants) 293. This pooled analysis also reported a moderately higher risk of diabetes (+6%) in individuals in the highest versus lowest quintiles of flavone and flavanone intakes. Moreover, the consumption of berries, rich in anthocyanins, has been shown to trigger favorable glycemic responses in type 2 diabetics 249. In recent intervention studies, anthocyanins demonstrated beneficial effects on metabolic abnormalities in patients at-risk or diagnosed with diabetes. In an eight-week, randomized, double-blind, placebo-controlled trial in 38 healthy overweight and obese subjects, the consumption of 2 g/day of grape polyphenols rich in proanthocyanidins and anthocyanins prevented increases in oxidative stress and insulin resistance induced by a six-day, high-fructose challenge 294. Another six-week randomized trial in individuals with diabetes showed that daily supplementation with Cornelian cherry (Cornus mas) extracts containing 600 mg of anthocyanins significantly lowered serum levels of HbA1c and triglycerides and increased serum insulin concentrations 295. The administration of 320 mg/day of anthocyanins for 24 weeks also improved serum lipid and lipoprotein profile, decreased markers of oxidative stress and inflammation, elevated antioxidant capacity, and reduced insulin resistance compared to a placebo in patients with diabetes 296. Furthermore, supplemental anthocyanins up-regulated adiponectin expression and improved nitric oxide-mediated endothelium-dependent vasodilation within 12 weeks of treatment 257.
These promising findings warrant additional randomized controlled trials to confirm preventive and/or therapeutic benefits of (cocoa) flavanols and anthocyanins in type 2 diabetes.
Neuroprotection and cognitive function
Flavonoids are thought to (1) promote neurogenesis, synaptic growth, and neuron survival in the learning and memory-related brain regions (e.g., hippocampus) by stimulating the production of neurotrophins like brain derived neurotrophic factor (BDNF); (2) protect hippocampal cells and striatal dopaminergic cells from cytotoxic molecules (pro-inflammatory mediators and ROS) released by abnormally activated microglia and hypertrophic astrocytes in neurodegenerative disorders; (3) reduce neuroinflammation by inhibiting the generation of pro-inflammatory cytokines, lipid mediators, and reactive oxygen species by astrocytes and microglial cells; (4) stimulate the production of nitric oxide (NO), which improves endothelial function, increases cerebral blood flow, and protects artery walls against the buildup of atherosclerotic plaques 297, 298.
Inflammation, oxidative stress, and transition metal accumulation appear to play a role in the pathology of several neurodegenerative diseases, including Parkinson’s disease and Alzheimer’s disease 299. The various properties of flavonoids, including their role in protecting vascular health, could have beneficial effects on the brain, possibly in the protection against cerebrovascular disorders, cognitive impairments, and subsequent stroke and dementias. Dietary flavonoids and/or their metabolites have been shown to cross the blood-brain barrier 298 and exert preventive effects towards cognitive impairments in animal models of normal and pathological aging 297.
The cross-sectional data analysis of 2,031 participants (ages, 70-74 years) from the Hordaland Health Study in Norway indicated that, when compared to non-consumers, consumers of flavonoid-rich chocolate, tea, and wine had better global cognitive function, assessed by a battery of six cognitive tests 300. The risk of poor performance in all tests was estimated to be 60 to 74% lower in consumers of all three flavonoid-rich foods compared to non-consumers. An early prospective cohort study in 1,367 older French men and women (aged ≥65 years; free from dementia at baseline) found that those with the lowest flavonoid intakes (<11.5 mg/day) had a 50% higher risk of developing dementia over the next five years than those with higher intakes 301. In addition, those with higher dietary flavonoid intakes at baseline experienced significantly less age-related cognitive decline over a 10-year period than those with the lowest flavonoid intakes 302.
The effect of cocoa flavan-3-ols have been investigated in an eight-week, randomized, double-blind trial — the Cognitive, Cocoa, and Aging (CoCoA) study — in 90 individuals (ages, 64-82 years) with mild cognitive impairments (MCI); participants were given dairy-based cocoa drinks with either high (993 mg/day) or low (48 mg/day) levels of flavan-3-ols 303. The daily consumption of the cocoa drink high in flavan-3-ols improved some, but not all, measures of cognitive process speed and flexibility and verbal fluency compared to baseline test scores and scores following low flavan-3-ol drink consumption. A composite test score reflecting overall cognitive performance was found to be significantly greater in those given cocoa drinks high rather than low in flavan-3-ols. The study also reported reductions in cardiovascular risk markers (i.e., systolic and diastolic blood pressure, total and LDL-cholesterol, insulin resistance), and these changes were proposed to partly contribute to ameliorate cognitive performance in those who consumed the flavan-3-ol-rich cocoa drink 303. The data could be replicated in cognitively healthy older people (ages, 61-85 years), suggesting that cocoa flavan-3-ols might enhance some aspects of cognitive function during healthy aging 304. A two-week, randomized, double-blind, controlled study has reported an increase in blood flow velocity in the middle cerebral artery of 21 healthy subjects (mean age, 72 years) following the daily intake of a flavan-3-ol-rich cocoa drink (900 mg/day of flavan-3-ols) 305. Because cerebral blood flow is correlated with cognitive function in humans, these preliminary data suggest that cocoa flavan-3-ol consumption could exert a protective effect against dementia 298.
In other randomized controlled trials 306, 307, 308, the lack of an effect of cocoa flavan-3-ols on blood pressure, cerebral blood flow, mental fatigue, and cognitive performance in healthy young and old adults suggested that benefits may only be seen in very demanding cognitive exercises 309.
Some randomized controlled studies also reported improvements in measures of cognitive function in healthy and cognitively impaired subjects with other flavonoid subclasses, including anthocyanins 310, flavanones 311, 312 and isoflavones 313, 314. Although some flavonoids and flavonoid-rich foods may enhance cognitive function in the aging brain, it is not yet clear whether their consumption could lower the risk of cognitive impairments and dementia in humans 50.
A recent observational study reported that people with high intakes of flavonols had a significantly lower rate of developing Alzheimer’s disease compared to people with low intakes 315. Flavonols are a class of flavonoids found naturally in many fruits, vegetables, tea, and wine, and have antioxidant and anti-inflammatory properties. Researchers found that participants who were in the top 20% in total flavonol intake had a 48% lower rate of developing Alzheimer’s disease compared to those in the bottom 20%, after controlling for major lifestyle and other factors associated with dementia, such as age, education, genetic risk factor (ApoE), cognitive activity (e.g., reading, playing games, and writing letters), and physical activity (e.g., walking, biking, yard work). Interestingly, the association between higher flavonol intake and reduced Alzheimer’s risk was stronger in men compared to women, such that men who were in the top 20% in total flavonol intake had a 76% lower rate of developing Alzheimer’s compared to men in the bottom 20%. The reasons for this sex difference are unknown. Because this study was an observational study and not a randomized clinical trial, it was not designed to prove that flavonols, or specific subclasses of flavonols like kaempferol, prevent Alzheimer’s disease. People who eat a diet rich in flavonols may be more likely to practice other healthy habits that are good for the brain. However, it is worth emphasizing that the associations between high flavonol intake and reduced Alzheimer’s disease risk remained significant even after controlling for some of these brain-healthy habits, such as cognitive and physical exercise. Findings from this current study is consistent with previous ones reporting that greater flavonoid intake is associated with higher cognitive scores and slower cognitive decline 316, 302. Therefore, a diet rich in fruits, dark-colored vegetables, and legumes appears to be beneficial for brain health and dementia prevention.
Anticancer activity
Flavonoids have been found to (1) scavenge free radicals that can damage macromolecules, including DNA 317, 318; (2) interfere with biotransformation enzymes and efflux transporters, possibly preventing the activation of procarcinogenic chemicals and promoting their excretion from the body 319, 320; (3) regulate proliferation, DNA repair, or activation of pathways leading to apoptosis (programmed cell death) in case of irreversible DNA damage 321; and (4) inhibit tumor invasion and angiogenesis 322, 323.
Hesperedin is an important flavonoid which displays efficient anticancer activity 324. Polylactic-co-glycolic acid (PLGA) nanoparticles were synthesized and loaded with hesperedin to form hesperidin nanoparticles to determine its potential application as an anticancer agent against C6 glioma cells. The encapsulated hesperedin exhibited decreased in vitro cell viability against the C6 glioma cell line, and the controlled release of hesperedin decreased the cytotoxicity of polylactic-co-glycolic acid (PLGA) 325.
Aurone, a benzo-furanone, is another flavonoid that has been extensively used as an anticancer agent 326. Various analogues of aurone display different mechanisms against cancer cells because there are many possible targets. These targets include cyclin dependent kinase, histone deacetylase, the adenosine receptor, telomerase, sirtuins, and microtubules 326.
Although various flavonoids have been found to inhibit the development of chemically-induced cancers in animal models of lung, oral, esophageal, gastric, colon, skin, prostate and breast cancer, observational studies do not provide convincing evidence that high intakes of dietary flavonoids are associated with substantial reductions in human cancer risk 327. A meta-analysis of 13 case-control and 10 prospective cohort studies found little-to-no evidence to support a preventive role of dietary flavonoid intake in gastric and colorectal cancer 328. In addition, a recently published analysis of two large prospective studies (the Health Professionals Follow-up Study [HPFS] and the Nurses’ Health Study [NHS]) — using the most up-to-date flavonoid food composition databases — found no association between the risk of colorectal cancer and intakes of each subclass of flavonoids or flavonoid-rich foods (tea, blueberries, oranges) 329. A meta-analysis of 19 case-control studies and 15 cohort studies found that total flavonoid intake and intakes of specific flavonoid subclasses (i.e., flavonols, flavones, flavanones) were inversely correlated with the risk of smoking-sensitive cancers of the aerodigestive tract (mouth, pharynx, larynx, esophagus, and stomach) in smokers but not in nonsmokers 330. The risk of lung cancer was not significantly associated with high flavonoid intakes 330, although an earlier meta-analysis of eight prospective studies (with substantial heterogeneity across them) suggested a protective role of flavonoids against lung cancer in smokers only 331. Moreover, a prospective analysis of over 45,000 postmenopausal women from the Multiethnic Cohort Study found a reduced risk of endometrial cancer with the highest intakes of total isoflavones, daidzein, and genistein 332. Additionally, limited evidence from observational studies suggests no relationship between total flavonoid intake and ovarian cancer 333, 334, 335, 336. To date, there is little evidence that flavonoid-rich diets might protect against various cancers, but larger prospective cohort studies are needed to address the association.
Because isoflavones are phytoestrogens, it is thought that they may interfere with the synthesis and activity of endogenous hormones, eventually influencing hormone-dependent signaling pathways and protecting against breast and prostate cancers 337. A meta-analysis of 14 observational studies that examined breast cancer incidence in 369,934 women found an overall 11% reduced risk of breast cancer with the highest versus lowest intake of soy isoflavones 338. Subgroup analyses revealed a 24% lower risk of cancer in Asian but not in European or US women, and the risk was 22% lower in postmenopausal but not lower in premenopausal women. In addition to the ethnicity and menopausal status, polymorphisms for hormone receptors 339 and phase 1 biotransformation enzymes 340 have been found to modify the association between isoflavone intake and breast cancer. Another recent meta-analysis of 12 observational studies (six prospective cohort studies, one nested case-control study, and five case-control studies) investigated the chemopreventive effects of flavonoids (except isoflavones) 341. The results suggested that intakes of flavonols and flavones may also be inversely associated with the risk of breast cancer. Furthermore, a pooled analysis of four case-control studies that stratified by menopausal status showed inverse associations between breast cancer and intakes of flavonols, flavones, or flavan-3-ols in postmenopausal women only. A meta-analysis of four prospective cohort studies found an overall 16% reduced risk of breast cancer recurrence in women with high versus low isoflavone intakes 338.
A meta-analysis of 13 observational studies also suggested an inverse relationship between prostate cancer risk and consumption of soy products, especially tofu 342. Yet, further analyses supported a protective role of soy food based only on case-control studies, which have inherent flaws such that associations may often be overestimated or underestimated. In a recent 12-month, multicenter, randomized, double-blind, placebo-controlled phase 2 clinical trial in 158 Japanese men (aged ≥50 years) with elevated risk of prostate cancer, oral isoflavone (60 mg/day) resulted in a significant decrease in prostate cancer incidence in participants aged 65 years and older 343. In this study, no changes were reported in sex hormone concentrations in blood, suggesting that isoflavones may reduce prostate cancer incidence without interfering with hormone-dependent pathways.
Additional investigations will be necessary to determine whether supplementation with specific flavonoids could benefit cancer prevention or treatment.
Flavonoids side effects
No adverse effects have been associated with high dietary intakes of flavonoids from plant-based food. Flavonoids that derive from fruits and vegetables are consumed in relatively low quantities. This lack of adverse effects may be explained by the relatively low bioavailability and rapid metabolism and elimination of most flavonoids from fruits and vegetables. However, flavonoid supplements may affect the action of blood thinners (medicines that help prevent blood clots) and increase the toxicity of a wide range of drugs when taken concurrently. In both animal and human studies, anti-thyroid and goitrogenic activities were observed by a high dose of green tea extracts and isoflavones 344, 345. Another adverse effect of high flavonoids doses includes inhibition of vitamin C transport, decreased trace element bioavailability, and impaired folate uptake 346.
Pregnancy and lactation
The safety of flavonoid supplements in pregnancy and lactation has not been established 141.
Interactions of flavonoids with drug transporters
Inhibition of ATP-binding cassette (ABC) drug transporters
A substantial number of studies demonstrate effects of flavonoids on transporters involved in drug metabolism 347, 348, 349. There are 2 main classes of drug transporters that are involved in the uptake and efflux of drugs and drug conjugates: 1) the ATP-binding cassette (ABC) transporters, which belong to the ATP-binding cassette family; and 2) the transporters of the solute carrier family 347, 348. Among the ATP-binding cassette (ABC) transporters, interactions have been shown with P-glycoprotein, multidrug resistance protein (MRP) associated proteins (MRP1 and MRP2), and with breast cancer resistance protein (BCRP) 350. ATP-binding cassette (ABC) drug transporters, including P-glycoprotein, multidrug resistance protein (MRP), and breast cancer-resistant protein (BCRP), function as ATP-dependent efflux pumps that actively regulate the excretion of a number of drugs limiting their systemic bioavailability 146. ATP-binding cassette (ABC) transporters are found throughout the body, yet they are especially important in organs with a barrier function like the intestines, the blood-brain barrier, blood-testis barrier, and the placenta, as well as in liver and kidneys 351. Most of the studies have demonstrated inhibitory effects of flavonoids on the substrate efflux in cells that either endogenously expressed these transporters or that were transfected with them 347, 350. This flavonoid-ABC-transporter interaction could be beneficial for poorly absorbed drugs but could also result in severe drug intoxication, especially for drugs with a narrow therapeutic window. On the other hand, flavonoids are themselves substrates of ABC transporters. These proteins can affect the oral availability and tissue distribution of these compounds, modifying their beneficial effects 352, 349. In contrast to the well-described interactions of flavonoids with ABC transporters, evidence for potential flavonoid effects on transporters of the solute carrier family is less clear.
There is some evidence that the consumption of grapefruit juice inhibits the activity of P-glycoprotein 353. Genistein, biochanin A, quercetin, naringenin, hesperetin, green tea flavan-3-ol epicatechin gallate (ECG), epigallocatechin (EGC), and epigallocatechin gallate (EGCG), and others have been found to inhibit the efflux activity of P-glycoprotein in cultured cells and in animal models 351. Therefore, very high or supplemental intakes of these flavonoids could potentially increase the toxicity of drugs that are substrates of P-glycoprotein, e.g., digoxin, antihypertensive agents, antiarrhythmic agents, chemotherapeutic (anticancer) agents, antifungal agents, HIV protease inhibitors, immunosuppressive agents, H2 receptor antagonists, some antibiotics, and others 354.
Many anthocyanins and anthocyanidins, as well as some flavones (apigenin, chrysin), isoflavones (biochanin A, genistein), flavonols (kaempferol), and flavanones (naringenin), have been identified as inhibitors of breast cancer-resistant protein (BCRP)-mediated transport, theoretically affecting drugs like anticancer agents (mitoxantrone, topotecan, thyrosine kinase inhibitors), antibiotics (fluoroquinolones), beta-blockers (prazosin), and antiarthritics (sulfasalazine) 50. Flavonols (quercetin, kaempferol, myricetin), flavanones (naringenin), flavones (apigenin, robinetin), and isoflavones (genistein) have been reported to inhibit multidrug resistance protein (MRP), potentially affecting MRP-mediated transport of many anticancer drugs, e.g., vincristin, etoposide, cisplatin, irinotecan, methotrexate, camptothecin, anthracyclines, vinca alkaloids 351.
Blood thinner (anticoagulant) and antiplatelet drugs
High intakes of flavonoids from purple grape juice (500 mL/day) and dark chocolate (235 mg/day of flavan-3-ols) have been found to inhibit platelet aggregation in ex vivo assays 355, 356, 357, 358. Theoretically, high intakes of flavonoids (e.g., from supplements) could increase the risk of bleeding when taken with anticoagulant drugs, such as warfarin (Coumadin), heparin, dalteparin (Fragmin), enoxaparin (Lovenox), and antiplatelet drugs, such as clopidogrel (Plavix), dipyridamole (Persantine), non-steroidal anti-inflammatory drugs (NSAIDs: diclofenac, ibuprofen, naproxen), aspirin, and others 50.
Inhibition of CYP 3A4 by flavonoid-rich grapefruit
Cytochrome P450 (CYP) enzymes are phase 1 biotransformation enzymes involved in the metabolism of a broad range of compounds, from endogenous molecules to therapeutic agents. The most abundant CYP isoform in the liver and intestines is cytochrome P450 3A4 (CYP3A4); the CYP3A family catalyzes the metabolism of about one-half of all marketed drugs in the US and Canada 359. One grapefruit or as little as 200 mL (7 fluid ounces) of grapefruit juice have been found to irreversibly inhibit intestinal CYP3A4 353. The most potent inhibitors of CYP3A4 in grapefruit are thought to be furanocoumarins, particularly dihydroxybergamottin, rather than flavonoids. All forms of the grapefruit — freshly squeezed juice, frozen concentrate, or whole fruit — can potentially affect the activity of CYP3A4. Some varieties of other citrus fruit (Seville oranges, limes, and pomelos) that contain furanocoumarins can also interfere with CYP3A4 activity.
The inhibition of intestinal CYP3A4 enzyme by grapefruit consumption is known or predicted to increase the bioavailability and the risk of toxicity of more than 85 drugs. Because drugs with very low bioavailability are more likely to be toxic when CYP3A4 activity is inhibited, they are associated with a higher risk of overdose with grapefruit compared to drugs with high bioavailability. Some of the drugs with low bioavailability include, but are not limited to, anticancer drugs (everolimus); anti-infective agents halofantrine, maraviroc); statins (atorvastatin, lovastatin, and simvastatin); cardioactive drugs (amiodarone, clopidogrel, dronedarone, eplenorone, ticagrelor); HIV protease inhibitors (saquinavir), immunosuppressants (cyclosporine, sirolimus, tacrolimus, everolimus); antihistamines (terfenadine); gastrointestinal agents (domperidone); central nervous system agents (buspirone, dextromethorphan, oral ketamine, lurasidone, quetiapine, selective serotonin reuptake inhibitors [sertraline]); and urinary tract agents (darifenacin) 359. Because of the potential for adverse drug interactions, some clinicians recommend that people taking medications with low bioavailability (i.e., undergoing extensive metabolism by CYP3A4) avoid consuming grapefruit and grapefruit juice altogether during the treatment period 359.
Iron interactions
Flavonoids can bind nonheme iron, inhibiting its intestinal absorption 183, 184. Nonheme iron is the principal form of iron in plant foods, dairy products, and iron supplements. The consumption of one cup of tea or cocoa with a meal has been found to decrease the absorption of nonheme iron in that meal by about 70% 185, 186. Flavonoids can also inhibit intestinal heme iron absorption 187. Interestingly, vitamin C (ascorbic acid) greatly enhances the absorption of iron and is able to counteract the inhibitory effect of flavonoids on nonheme and heme iron absorption 184, 187, 188. To maximize iron absorption from a meal or iron supplements, flavonoid-rich food and beverages and flavonoid supplements should not be consumed at the same time.
- Santos E.L., Maia B., Ferriani A.P., Teixeira S.D. Flavonoids: From Biosynthesis to Human Health. Volume 13. IntechOpen; London, UK: 2017. Flavonoids: Classification, biosynthesis and chemical ecology; pp. 78–94.[↩]
- Lin J.K., Weng M.S. Flavonoids as nutraceuticals. Sci. Flavonoids. 2006;7:213–238. doi: 10.1007/978-0-387-28822-2_8[↩]
- Panche A.N., Diwan A.D., Chandra S.R. Flavonoids: An overview. J. Nutr. Sci. 2016;5:e47. doi: 10.1017/jns.2016.41[↩][↩]
- Shan X., Cheng J., Chen K.l., Liu Y.M., Juan L. Comparison of Lipoxygenase, Cyclooxygenase, Xanthine Oxidase Inhibitory Effects and Cytotoxic Activities of Selected Flavonoids. DEStech Trans. Environ. Energy Earth Sci. 2017 doi: 10.12783/dteees/gmee2017/16624[↩]
- Feliciano R.P., Pritzel S., Heiss C., Rodriguez-Mateos A. Flavonoid intake and cardiovascular disease risk. Curr. Opin. Food Sci. 2015;2:92–99. doi: 10.1016/j.cofs.2015.02.006[↩]
- Panche AN, Diwan AD, Chandra SR. Flavonoids: an overview. J Nutr Sci. 2016 Dec 29;5:e47. doi: 10.1017/jns.2016.41[↩][↩][↩][↩]
- Ullah A, Munir S, Badshah SL, Khan N, Ghani L, Poulson BG, Emwas AH, Jaremko M. Important Flavonoids and Their Role as a Therapeutic Agent. Molecules. 2020 Nov 11;25(22):5243. doi: 10.3390/molecules25225243[↩]
- Khan M.K., Zill E.H., Dangles O. A comprehensive review on flavanones, the major citrus polyphenols. J. Food Compos. Anal. 2014;33:85–104. doi: 10.1016/j.jfca.2013.11.004[↩]
- Khalifa I., Zhu W., Li K.-k., Li C.-m. Polyphenols of mulberry fruits as multifaceted compounds: Compositions, metabolism, health benefits, and stability—A structural review. J. Funct. Foods. 2018;40:28–43. doi: 10.1016/j.jff.2017.10.041[↩]
- Bondonno N.P., Lewis J.R., Blekkenhorst L.C., Bondonno C.P., Shin J.H., Croft K.D., Woodman R.J., Wong G., Lim W.H., Gopinath B. Association of flavonoids and flavonoid-rich foods with all-cause mortality: The Blue Mountains Eye Study. Clin. Nutr. 2019;39:141–150. doi: 10.1016/j.clnu.2019.01.004[↩]
- García-Lafuente A., Guillamón E., Villares A., Rostagno M.A., Martínez J.A. Flavonoids as anti-inflammatory agents: Implications in cancer and cardiovascular disease. Inflamm. Res. 2009;58:537–552. doi: 10.1007/s00011-009-0037-3[↩]
- Zhao L., Yuan X., Wang J., Feng Y., Ji F., Li Z., Bian J. A review on flavones targeting serine/threonine protein kinases for potential anticancer drugs. Bioorganic Med. Chem. 2019;27:677–685. doi: 10.1016/j.bmc.2019.01.027[↩]
- Zhao K., Yuan Y., Lin B., Miao Z., Li Z., Guo Q., Lu N. LW-215, a newly synthesized flavonoid, exhibits potent anti-angiogenic activity in vitro and in vivo. Gene. 2018;642:533–541. doi: 10.1016/j.gene.2017.11.065[↩]
- Camero C.M., Germanò M.P., Rapisarda A., D’Angelo V., Amira S., Benchikh F., Braca A., De Leo M. Anti-angiogenic activity of iridoids from Galium tunetanum. Rev. Bras. de Farmacogn. 2018;28:374–377. doi: 10.1016/j.bjp.2018.03.010[↩]
- Patel K., Kumar V., Rahman M., Verma A., Patel D.K. New insights into the medicinal importance, physiological functions and bioanalytical aspects of an important bioactive compound of foods ‘Hyperin’: Health benefits of the past, the present, the future. Beni-Suef Univ. J. Basic Appl. Sci. 2018;7:31–42. doi: 10.1016/j.bjbas.2017.05.009[↩]
- Villela A., van Vuuren M.S., Willemen H.M., Derksen G.C., van Beek T.A. Photo-stability of a flavonoid dye in presence of aluminium ions. Dyes Pigment. 2019;162:222–231. doi: 10.1016/j.dyepig.2018.10.021[↩]
- Paramita V., Kusumayanti H., Amalia R., Leviana W., Nisa Q.A. Application of Flavonoid and Anthocyanin Contents from Rambutan (Nephelium lappaceum) Peel as Natural Dyes on Cotton Fabric. Adv. Sci. Lett. 2018;24:9853–9855. doi: 10.1166/asl.2018.13160[↩]
- Lanzendörfer G., Stäb F., Untiedt S. Cosmetic and Dermatological Preparations with Flavonoids. WO/1996/018379. 1996 Jun 20[↩]
- Danihelová M., Viskupičová J., Šturdík E. Lipophilization of flavonoids for their food, therapeutic and cosmetic applications. Acta Chim. Slovaca. 2012;5:59–69. doi: 10.2478/v10188-012-0010-6[↩]
- Chuarienthong P., Lourith N., Leelapornpisid P. Clinical efficacy comparison of anti-wrinkle cosmetics containing herbal flavonoids. Int. J. Cosmet. Sci. 2010;32:99–106. doi: 10.1111/j.1468-2494.2010.00522.x[↩]
- Balasuriya N., Rupasinghe H.V. Antihypertensive properties of flavonoid-rich apple peel extract. Food Chem. 2012;135:2320–2325. doi: 10.1016/j.foodchem.2012.07.023[↩]
- Xue Z., Wang J., Chen Z., Ma Q., Guo Q., Gao X., Chen H. Antioxidant, antihypertensive, and anticancer activities of the flavonoid fractions from green, oolong, and black tea infusion waste. J. Food Biochem. 2018;42:e12690. doi: 10.1111/jfbc.12690[↩]
- Khan S., Khan T., Shah A.J. Total phenolic and flavonoid contents and antihypertensive effect of the crude extract and fractions of Calamintha vulgaris. Phytomedicine. 2018;47:174–183. doi: 10.1016/j.phymed.2018.04.046[↩]
- Lagunas-Herrera H., Tortoriello J., Herrera-Ruiz M., Martínez-Henández G.B., Zamilpa A., Santamaría L.A., Lorenzana M.G., Lombardo-Earl G., Jiménez-Ferrer E. Acute and Chronic Antihypertensive Effect of Fractions, Tiliroside and Scopoletin from Malva parviflora. Biol. Pharm. Bull. 2019;42:18–25. doi: 10.1248/bpb.b18-00355[↩]
- Mazidi M., Katsiki N., Banach M. A higher flavonoid intake is associated with less likelihood of nonalcoholic fatty liver disease: Results from a multiethnic study. J. Nutr. Biochem. 2019;65:66–71. doi: 10.1016/j.jnutbio.2018.10.001[↩]
- Aguiar L.M., Geraldi M.V., Cazarin C.B.B., Junior M.R.M. Functional Food Consumption and Its Physiological Effects. In: Campos M.R.S., editor. Bioactive Compounds. Woodhead Publishing; Sawston, UK: 2019. pp. 205–225.[↩]
- Ullah A., Munir S., Badshah S.L., Khan N., Ghani L., Poulson B.G., Emwas A.-H., Jaremko M. Important Flavonoids and Their Role as a Therapeutic Agent. Molecules. 2020;25:5243. doi: 10.3390/molecules25225243[↩]
- Nijveldt RJ, van Nood E, van Hoorn DE, Boelens PG, van Norren K, van Leeuwen PA. Flavonoids: a review of probable mechanisms of action and potential applications. Am J Clin Nutr. 2001 Oct;74(4):418-25. doi: 10.1093/ajcn/74.4.418[↩]
- Hussain T., Tan B., Murtaza G., Liu G., Yin Y. Flavonoids and type 2 diabetes: Evidence of efficacy in clinical and animal studies and delivery strategies to enhance their therapeutic efficacy. Pharm. Res. 2020;152:104629. doi: 10.1016/j.phrs.2020.104629[↩]
- Harborne JB, Williams CA. Advances in flavonoid research since 1992. Phytochemistry. 2000 Nov;55(6):481-504. doi: 10.1016/s0031-9422(00)00235-1[↩][↩]
- Liu RH. Health-promoting components of fruits and vegetables in the diet. Adv Nutr. 2013 May 1;4(3):384S-92S. doi: 10.3945/an.112.003517[↩][↩]
- Rehan M. Bioactive Compounds-Biosynthesis, Characterization and Applications. IntechOpen; London, UK: 2021. Biosynthesis of Diverse Class Flavonoids via Shikimate and Phenylpropanoid Pathway; p. 75392.[↩]
- Zakaryan H., Arabyan E., Oo A., Zandi K. Flavonoids: Promising natural compounds against viral infections. Arch. Virol. 2017;162:2539–2551. doi: 10.1007/s00705-017-3417-y[↩]
- Kumar, Shashank, Pandey, Abhay K., Chemistry and Biological Activities of Flavonoids: An Overview, The Scientific World Journal, 2013, 162750, 16 pages, 2013. https://doi.org/10.1155/2013/162750[↩][↩]
- Shamsudin NF, Ahmed QU, Mahmood S, Shah SAA, Sarian MN, Khattak MMAK, Khatib A, Sabere ASM, Yusoff YM, Latip J. Flavonoids as Antidiabetic and Anti-Inflammatory Agents: A Review on Structural Activity Relationship-Based Studies and Meta-Analysis. Int J Mol Sci. 2022 Oct 20;23(20):12605. doi: 10.3390/ijms232012605[↩]
- Cheng A.X., Han X.J., Wu Y.F., Lou H.X. The function and catalysis of 2-oxoglutarate-dependent oxygenases involved in plant flavonoid biosynthesis. Int. J. Mol. Sci. 2014;15:1080–1095. doi: 10.3390/ijms15011080[↩]
- Dastmalchi M., Dhaubhadel S. Soybean chalcone isomerase: Evolution of the fold, and the differential expression and localization of the gene family. Planta. 2015;241:507–523. doi: 10.1007/s00425-014-2200-5[↩]
- Richter A.S. Tansley insight. New Phytol. 2022;236:2037–2043. doi: 10.1111/nph.18488[↩]
- Baba S.A., Ashraf N. Functional characterization of flavonoid 3′-hydroxylase, CsF3′ H, from Crocus sativus L: Insights into substrate specificity and role in abiotic stress. Arch. Biochem. Biophys. 2019;667:70–78. doi: 10.1016/j.abb.2019.04.012[↩]
- Li H., Tian J., Yao Y., Zhang J., Song T., Li K., Yao Y. Identification of leucoanthocyanidin reductase and anthocyanidin reductase genes involved in proanthocyanidin biosynthesis in Malus crabapple plants. Plant Physiol. Biochem. 2019;139:141–151. doi: 10.1016/j.plaphy.2019.03.003[↩]
- Ma S., Hu R., Ma J., Fan J., Wu F., Wang Y., Huang L., Feng G., Li D., Nie G., et al. Integrative analysis of the metabolome and transcriptome provides insights into the mechanisms of anthocyanins and proanthocyanidins biosynthesis in Trifolium repens. Ind. Crop. Prod. 2022;187:115529. doi: 10.1016/j.indcrop.2022.115529[↩]
- Rauf A., Imran M., Abu-Izneid T., Haq I.U., Pate S., Pan X., Naz S., Silva A.S., Saeed F., Suleria H.A.R. Proanthocyanidins: A comprehensive review. Biomed. Pharmacother. 2019;116:108999. doi: 10.1016/j.biopha.2019.108999[↩]
- Ni J., Zhao Y., Tao R., Yin L., Gao L., Strid Å., Qian M., Li J., Li Y., Shen J., et al. Ethylene mediates the branching of the jasmonate-induced flavonoid biosynthesis pathway by suppressing anthocyanin biosynthesis in red Chinese pear fruits. Plant Biotechnol. J. 2020;18:1223–1240. doi: 10.1111/pbi.13287[↩]
- Kim M.J., Paramanantham A., Lee W.S., Yun J.W., Chang S.H., Kim D.C., Park H.S., Choi Y.H., Kim G.S., Ryu C.H., et al. Anthocyanins Derived from Vitis coignetiae Pulliat Contributes Anti-Cancer Effects by Suppressing NF-κB Pathways in Hep3B Human Hepatocellular Carcinoma Cells and In Vivo. Molecules. 2020;25:5445. doi: 10.3390/molecules25225445[↩]
- Chen S, Wang X, Cheng Y, Gao H, Chen X. A Review of Classification, Biosynthesis, Biological Activities and Potential Applications of Flavonoids. Molecules. 2023 Jun 25;28(13):4982. doi: 10.3390/molecules28134982[↩][↩][↩][↩][↩][↩][↩][↩][↩][↩][↩]
- Waheed Janabi AH, Kamboh AA, Saeed M, Xiaoyu L, BiBi J, Majeed F, Naveed M, Mughal MJ, Korejo NA, Kamboh R, Alagawany M, Lv H. Flavonoid-rich foods (FRF): A promising nutraceutical approach against lifespan-shortening diseases. Iran J Basic Med Sci. 2020 Feb;23(2):140-153. doi: 10.22038/IJBMS.2019.35125.8353[↩][↩][↩][↩]
- Panche A., Diwan A., Chandra S. Flavonoids: An overview. J. Nutr. Sci. 2016;5:e47. doi: 10.1017/jns.2016.41[↩]
- Manach C, Scalbert A, Morand C, Rémésy C, Jiménez L. Polyphenols: food sources and bioavailability. Am J Clin Nutr. 2004 May;79(5):727-47. doi: 10.1093/ajcn/79.5.727[↩][↩][↩][↩][↩]
- Xiao J, Kai G, Yamamoto K, Chen X. Advance in dietary polyphenols as α-glucosidases inhibitors: a review on structure-activity relationship aspect. Crit Rev Food Sci Nutr. 2013;53(8):818-36. doi: 10.1080/10408398.2011.561379[↩]
- Flavonoids. https://lpi.oregonstate.edu/mic/dietary-factors/phytochemicals/flavonoids[↩][↩][↩][↩][↩][↩][↩][↩][↩][↩][↩][↩][↩][↩][↩][↩][↩][↩]
- Fang J. Classification of fruits based on anthocyanin types and relevance to their health effects. Nutrition. 2015;31:1301–1306. doi: 10.1016/j.nut.2015.04.015[↩][↩]
- Tohge T., de Souza L.P., Fernie A.R. Current understanding of the pathways of flavonoid biosynthesis in model and crop plants. J. Exp. Bot. 2017;68:4013–4028. doi: 10.1093/jxb/erx177[↩]
- Kim J.I., Hidalgo-Shrestha C., Bonawitz N.D., Franke R.B., Clint C. Spatio-temporal control of phenylpropanoid biosynthesis by inducible complementation of a cinnamate 4-hydroxylase mutant. J. Exp. Bot. 2021;72:3061–3073. doi: 10.1093/jxb/erab055[↩]
- Shen N., Wang T., Gan Q., Liu S., Wang L., Jin B. Plant flavonoids: Classification, distribution, biosynthesis, and antioxidant activity. Food Chem. 2022;383:132531. doi: 10.1016/j.foodchem.2022.132531[↩][↩]
- Gonzali S., Perata P. Anthocyanins from purple tomatoes as novel antioxidants to promote human health. Antioxidants. 2020;9:1017. doi: 10.3390/antiox9101017[↩]
- Qaisar U., Afzal M., Tayyeb A. Commercial Application of Plant pigments. Int. J. Biotech Trend. Technol. 2019;9:18–22. doi: 10.14445/22490183/IJBTT-V9I3P604[↩]
- Khoo H.E., Azlan A., Tang S.T., Lim S.M. Anthocyanidins and anthocyanins: Colored pigments as food, pharmaceutical ingredients, and the potential health benefits. Food Nutr. Res. 2017;61:1361779. doi: 10.1080/16546628.2017.1361779[↩]
- Veberic R., Slatnar A., Bizjak J., Stampar F., Mikulic-Petkovsek M. Anthocyanin composition of different wild and cultivated berry species. LWT-Food Sci. Technol. 2015;60:509–517. doi: 10.1016/j.lwt.2014.08.033[↩]
- Chaves-Silva S., Dos Santos A.L., Chalfun-Júnior A., Peres L.E.P., Zhao J., Benedito V.A. Understanding the genetic regulation of anthocyanin biosynthesis in plants–tools for breeding purple varieties of fruits and vegetables. Phytochemistry. 2018;153:11–27. doi: 10.1016/j.phytochem.2018.05.013[↩]
- Vendrame S, Klimis-Zacas D. Potential Factors Influencing the Effects of Anthocyanins on Blood Pressure Regulation in Humans: A Review. Nutrients. 2019 Jun 25;11(6):1431. doi: 10.3390/nu11061431[↩]
- Mattioli R, Francioso A, Mosca L, Silva P. Anthocyanins: A Comprehensive Review of Their Chemical Properties and Health Effects on Cardiovascular and Neurodegenerative Diseases. Molecules. 2020 Aug 21;25(17):3809. doi: 10.3390/molecules25173809[↩]
- Lin BW, Gong CC, Song HF, Cui YY. Effects of anthocyanins on the prevention and treatment of cancer. Br J Pharmacol. 2017 Jun;174(11):1226-1243. doi: 10.1111/bph.13627[↩]
- Dutta M.S., Mahapatra P., Ghosh A., Basu S. Estimation of the reducing power and electrochemical behavior of few flavonoids and polyhydroxybenzophenones substantiated by bond dissociation energy: A comparative analysis. Mol. Divers. 2022;26:1101–1113. doi: 10.1007/s11030-021-10232-4[↩]
- Aron PM, Kennedy JA. Flavan-3-ols: nature, occurrence and biological activity. Mol Nutr Food Res. 2008 Jan;52(1):79-104. doi: 10.1002/mnfr.200700137[↩]
- Pico J., Xu K., Guo M., Mohamedshah Z., Ferruzzi M.G., Martinez M.M. Manufacturing the ultimate green banana flour: Impact of drying and extrusion on phenolic profile and starch bioaccessibility. Food Chem. 2019;297:124990. doi: 10.1016/j.foodchem.2019.124990[↩][↩]
- Ding T., Cao K., Fang W., Zhu G., Chen C., Wang X., Wang L. Evaluation of phenolic components (anthocyanins, flavanols, phenolic acids, and flavonols) and their antioxidant properties of peach fruits. Sci. Hortic. 2020;268:109365. doi: 10.1016/j.scienta.2020.109365[↩]
- Yang S., Mi L., Wu J., Liao X., Xu Z. Strategy for anthocyanins production: From efficient green extraction to novel microbial biosynthesis. Crit. Rev. Food Sci. 2022:1–16. doi: 10.1080/10408398.2022.2067117[↩]
- Zhang T., Wei X., Miao Z., Hassan H., Song Y., Fan M. Screening for antioxidant and antibacterial activities of phenolics from Golden Delicious apple pomace. Chem. Cent. J. 2016;10:47. doi: 10.1186/s13065-016-0195-7[↩]
- Guven H., Arici A., Simsek O. Flavonoids in our foods: A short review. J. Basic. Clin. Health Sci. 2019;3:96–106. doi: 10.30621/jbachs.2019.555[↩]
- Di Pietro N., Baldassarre M.P.A., Cichelli A., Pandolfi A., Formoso G., Pipino C. Role of polyphenols and carotenoids in endothelial dysfunction: An overview from classic to innovative biomarkers. Oxid. Med. Cell. Longev. 2020;2020:6381380. doi: 10.1155/2020/6381380[↩]
- Proteggente A.R., Pannala A.S., Paganga G., Buren L.V., Wagner E., Wiseman S., Van De Put F., Dacombe C., Rice-Evans C.A. The antioxidant activity of regularly consumed fruit and vegetables reflects their phenolic and vitamin C composition. Free Radic. Res. 2002;36:217–233. doi: 10.1080/10715760290006484[↩]
- Martínez-Lüscher J., Brillante L., Kurtural S.K. Flavonol profile is a reliable indicator to assess canopy architecture and the exposure of red wine grapes to solar radiation. Front. Plant. Sci. 2019;10:10. doi: 10.3389/fpls.2019.00010[↩]
- Ali A., Parisi A., Normanno G. Emerging Modalities in Mitigation of Antimicrobial Resistance. Springer International Publishing; Cham, Switzerland: 2022. Polyphenols as emerging antimicrobial agents; pp. 219–259.[↩]
- Luca S.V., Macovei I., Bujor A., Miron A., Skalicka-Woźniak K., Aprotosoaie A.C., Trifan A. Bioactivity of dietary polyphenols: The role of metabolites. Crit. Rev. Food Sci. 2020;60:626–659. doi: 10.1080/10408398.2018.1546669[↩]
- Bose S., Sarkar D., Bose A., Mandal S.C. Natural flavonoids and its pharmaceutical importance. Pharma Rev. 2018;94:61–75.[↩]
- Tang D., Chen K., Huang L., Li J. Pharmacokinetic properties and drug interactions of apigenin, a natural flavone. Expert. Opin. Drug Metab. Toxi. 2017;13:323–330. doi: 10.1080/17425255.2017.1251903[↩]
- Vicente O., Boscaiu M. Flavonoids: Antioxidant compounds for plant defence and for a healthy human diet. Not. Bot. Horti Agrobot. Cluj. 2018;46:14–21. doi: 10.15835/nbha46110992[↩][↩]
- Ginwala R., Bhavsar R., Chigbu D.G.I., Jain P., Khan Z.K. Potential role of flavonoids in treating chronic inflammatory diseases with a special focus on the anti-inflammatory activity of apigenin. Antioxidants. 2019;8:35. doi: 10.3390/antiox8020035[↩]
- Vandercook C.E., Stephenson R.G. Lemon juice composition. Identification of major phenolic compounds and estimation by paper chromatography. J. Agr. Food Chem. 1966;14:450–454. doi: 10.1021/jf60147a003[↩]
- Wang Y., Liu X.J., Chen J.B., Cao J.P., Sun C.D. Citrus flavonoids and their antioxidant evaluation. Crit. Rev. Food Sci. 2022;62:3833–3854. doi: 10.1080/10408398.2020.1870035[↩]
- Peterson J.J., Dwyer J.T., Beecher G.R., Bhagwat S.A., Gabhardt S.E., Haytowitz D.B., Holden J.M. Flavanones in oranges, tangerines (mandarins), tangors and tangelos: A compilation and review of the data from the analytical literature. J. Food Compos. Anal. 2006;19:S66–S73. doi: 10.1016/j.jfca.2005.12.006[↩]
- Najmanová I., Vopršalová M., Saso L., Mladěnka P. The pharmacokinetics of flavanones. Crit. Rev. Food Sci. 2020;60:3155–3171. doi: 10.1080/10408398.2019.1679085[↩][↩]
- Roy M., Datta A. Cancer Genetics and Therapeutics. Springer; Singapore: 2019. Fundamentals of phytochemicals; pp. 49–81.[↩]
- Galleano M., Calabro V., Prince P.D., Litterio M.C., Piotrkowski B., Vazquez-Prieto M.A., Miatello R.M., Oteiza P.I., Fraga C.G. Flavonoids and metabolic syndrome. Ann. Acad. Sci. 2012;1259:87–94. doi: 10.1111/j.1749-6632.2012.06511.x[↩]
- Liu Y., Qian J., Li J., Xing M., Grierson D., Sun C., Xu C., Li X., Chen K. Hydroxylation decoration patterns of flavonoids in horticultural crops: Chemistry, bioactivity, and biosynthesis. Hortic. Res. 2022;9:uhab068. doi: 10.1093/hr/uhab068[↩]
- Shah A., Smith D.L. Flavonoids in agriculture: Chemistry and roles in, biotic and abiotic stress responses, and microbial associations. Agronomy. 2020;10:1209. doi: 10.3390/agronomy10081209[↩]
- Bharti S., Rani N., Krishnamurthy B., Arya D.S. Preclinical evidence for the pharmacological actions of naringin: A review. Planta Med. 2014;80:437–451. doi: 10.1055/s-0034-1368351[↩]
- Testai L, Calderone V. Nutraceutical Value of Citrus Flavanones and Their Implications in Cardiovascular Disease. Nutrients. 2017 May 16;9(5):502. doi: 10.3390/nu9050502[↩]
- Kopečná-Zapletalová M., Krasulová K., Anzenbacher P., Hodek P., Anzenbacherová E. Interaction of isoflavonoids with human liver microsomal cytochromes P450: Inhibition of CYP enzyme activities. Xenobiotica. 2017;47:324–331. doi: 10.1080/00498254.2016.1195028[↩]
- Hummelova J., Rondevaldova J., Balastikova A., Lapcik O., Kokoska L. The relationship between structure and in vitro antibacterial activity of selected isoflavones and their metabolites with special focus on antistaphylococcal effect of demethyltexasin. Lett. Appl. Microbiol. 2015;60:242–247. doi: 10.1111/lam.12361[↩]
- Lim Y.J., Jeong H.Y., Gil C.S., Kwon S.J., Na J.K., Lee C., Eom S.H. Isoflavone accumulation and the metabolic gene expression in response to persistent UV-B irradiation in soybean sprouts. Food Chem. 2020;303:125376. doi: 10.1016/j.foodchem.2019.125376[↩]
- Meng N., Yu B.J., Guo J.S. Ameliorative effects of inoculation with Bradyrhizobium japonicum on Glycine max and Glycine soja seedlings under salt stress. Plant. Growth Regul. 2016;80:137–147. doi: 10.1007/s10725-016-0150-6[↩]
- Mori-Yasumoto K., Hashimoto Y., Agatsuma Y., Fuchino H., Yasumoto K., Shirota O., Satake M., Sekita S. Leishmanicidal phenolic compounds derived from Dalbergia cultrata. Nat. Prod. Res. 2021;35:4907–4915. doi: 10.1080/14786419.2020.1744140[↩][↩]
- Goyal K., Kaur R., Goyal A., Awasthi R. Chalcones: A review on synthesis and pharmacological activities. J. Appl. Pharm. Sci. 2021;11:001–014. doi: 10.7324/JAPS.2021.11s101[↩]
- Jasim H.A., Nahar L., Jasim M.A., Moore S.A., Ritchie K.J., Sarker S.D. Chalcones: Synthetic chemistry follows where nature leads. Biomolecules. 2021;11:1203. doi: 10.3390/biom11081203[↩]
- Butt MS, Imran A, Sharif MK, Ahmad RS, Xiao H, Imran M, Rsool HA. Black tea polyphenols: a mechanistic treatise. Crit Rev Food Sci Nutr. 2014;54(8):1002-11. doi: 10.1080/10408398.2011.623198[↩]
- Mink PJ, Scrafford CG, Barraj LM, Harnack L, Hong CP, Nettleton JA, Jacobs DR Jr. Flavonoid intake and cardiovascular disease mortality: a prospective study in postmenopausal women. Am J Clin Nutr. 2007 Mar;85(3):895-909. doi: 10.1093/ajcn/85.3.895[↩]
- Kim K, Vance TM, Chun OK. Estimated intake and major food sources of flavonoids among US adults: changes between 1999-2002 and 2007-2010 in NHANES. Eur J Nutr. 2016 Mar;55(2):833-843. doi: 10.1007/s00394-015-0942-x[↩]
- Sebastian RS, Wilkinson Enns C, Goldman JD, Martin CL, Steinfeldt LC, Murayi T, Moshfegh AJ. A New Database Facilitates Characterization of Flavonoid Intake, Sources, and Positive Associations with Diet Quality among US Adults. J Nutr. 2015 Jun;145(6):1239-48. doi: 10.3945/jn.115.213025[↩]
- US Department of Agriculture. USDA Database for the Flavonoid Content of Selected Foods, release 3.1. May 2014. https://www.ars.usda.gov/ARSUserFiles/80400525/Data/Flav/Flav_R03-1.pdf[↩]
- US Department of Agriculture. USDA Database for the Proanthocyanidin Content of Selected Foods. August, 2004. https://www.ars.usda.gov/ARSUserFiles/80400525/Data/PA/PA.pdf[↩]
- US Department of Agriculture. USDA Database for the Isoflavone Content of Selected Foods, release 2.0. September 2008. https://www.ars.usda.gov/ARSUserFiles/80400525/Data/isoflav/Isoflav_R2.pdf[↩]
- Jatoi A, Ellison N, Burch PA, Sloan JA, Dakhil SR, Novotny P, Tan W, Fitch TR, Rowland KM, Young CY, Flynn PJ. A phase II trial of green tea in the treatment of patients with androgen independent metastatic prostate carcinoma. Cancer. 2003 Mar 15;97(6):1442-6. doi: 10.1002/cncr.11200[↩][↩]
- Pisters KM, Newman RA, Coldman B, Shin DM, Khuri FR, Hong WK, Glisson BS, Lee JS. Phase I trial of oral green tea extract in adult patients with solid tumors. J Clin Oncol. 2001 Mar 15;19(6):1830-8. doi: 10.1200/JCO.2001.19.6.1830[↩]
- Chow HH, Cai Y, Hakim IA, Crowell JA, Shahi F, Brooks CA, Dorr RT, Hara Y, Alberts DS. Pharmacokinetics and safety of green tea polyphenols after multiple-dose administration of epigallocatechin gallate and polyphenon E in healthy individuals. Clin Cancer Res. 2003 Aug 15;9(9):3312-9.[↩][↩]
- Dostal AM, Samavat H, Bedell S, Torkelson C, Wang R, Swenson K, Le C, Wu AH, Ursin G, Yuan JM, Kurzer MS. The safety of green tea extract supplementation in postmenopausal women at risk for breast cancer: results of the Minnesota Green Tea Trial. Food Chem Toxicol. 2015 Sep;83:26-35. doi: 10.1016/j.fct.2015.05.019[↩][↩][↩]
- Ottaviani JI, Balz M, Kimball J, Ensunsa JL, Fong R, Momma TY, Kwik-Uribe C, Schroeter H, Keen CL. Safety and efficacy of cocoa flavanol intake in healthy adults: a randomized, controlled, double-masked trial. Am J Clin Nutr. 2015 Dec;102(6):1425-35. doi: 10.3945/ajcn.115.116178[↩]
- Terao J. Potential Role of Quercetin Glycosides as Anti-Atherosclerotic Food-Derived Factors for Human Health. Antioxidants. 2023;12:258. doi: 10.3390/antiox12020258[↩]
- Georgiou N, Kakava MG, Routsi EA, Petsas E, Stavridis N, Freris C, Zoupanou N, Moschovou K, Kiriakidi S, Mavromoustakos T. Quercetin: A Potential Polydynamic Drug. Molecules. 2023 Dec 17;28(24):8141. doi: 10.3390/molecules28248141[↩]
- Cui Z, Zhao X, Amevor FK, Du X, Wang Y, Li D, Shu G, Tian Y, Zhao X. Therapeutic application of quercetin in aging-related diseases: SIRT1 as a potential mechanism. Front Immunol. 2022 Jul 22;13:943321. doi: 10.3389/fimmu.2022.943321[↩]
- Lotfi N, Yousefi Z, Golabi M, Khalilian P, Ghezelbash B, Montazeri M, Shams MH, Baghbadorani PZ, Eskandari N. The potential anti-cancer effects of quercetin on blood, prostate and lung cancers: An update. Front Immunol. 2023 Feb 28;14:1077531. doi: 10.3389/fimmu.2023.1077531[↩]
- Zhang X, Tang Y, Lu G, Gu J. Pharmacological Activity of Flavonoid Quercetin and Its Therapeutic Potential in Testicular Injury. Nutrients. 2023 May 8;15(9):2231. doi: 10.3390/nu15092231[↩]
- Carrillo-Martinez EJ, Flores-Hernández FY, Salazar-Montes AM, Nario-Chaidez HF, Hernández-Ortega LD. Quercetin, a Flavonoid with Great Pharmacological Capacity. Molecules. 2024 Feb 25;29(5):1000. doi: 10.3390/molecules29051000[↩]
- Hosseini A., Razavi B.M., Banach M., Hosseinzadeh H. Quercetin and metabolic syndrome: A review. Phytother. Res. PTR. 2021;35:5352–5364. doi: 10.1002/ptr.7144[↩]
- Shen P., Lin W., Deng X., Ba X., Han L., Chen Z., Qin K., Huang Y., Tu S. Potential Implications of Quercetin in Autoimmune Diseases. Front. Immunol. 2021;12:689044. doi: 10.3389/fimmu.2021.689044[↩]
- Endothelial function and cardiovascular disease: effects of quercetin and wine polyphenols. Free Radic Res. 2006 Oct;40(10):1054-65. https://www.ncbi.nlm.nih.gov/pubmed/17015250[↩]
- Ross JA, Kasum CM. Dietary flavonoids: bioavailability, metabolic effects, and safety. Annu Rev Nutr. 2002;22:19-34. doi: 10.1146/annurev.nutr.22.111401.144957[↩]
- Najafzadeh M, Reynolds PD, Baumgartner A, Anderson D. Flavonoids inhibit the genotoxicity of hydrogen peroxide (H(2)O(2[↩]
- Nair MP, Mahajan S, Reynolds JL, Aalinkeel R, Nair H, Schwartz SA, Kandaswami C. The flavonoid quercetin inhibits proinflammatory cytokine (tumor necrosis factor alpha) gene expression in normal peripheral blood mononuclear cells via modulation of the NF-kappa beta system. Clin Vaccine Immunol. 2006 Mar;13(3):319-28. doi: 10.1128/CVI.13.3.319-328.2006[↩]
- Razavi SM, Zahri S, Zarrini G, Nazemiyeh H, Mohammadi S. Biological activity of quercetin-3-O-glucoside, a known plant flavonoid. Bioorg Khim. 2009 May-Jun;35(3):414-6. doi: 10.1134/s1068162009030133[↩]
- Kempuraj D, Castellani ML, Petrarca C, Frydas S, Conti P, Theoharides TC, Vecchiet J. Inhibitory effect of quercetin on tryptase and interleukin-6 release, and histidine decarboxylase mRNA transcription by human mast cell-1 cell line. Clin Exp Med. 2006 Dec;6(4):150-6. doi: 10.1007/s10238-006-0114-7[↩]
- Seufi AM, Ibrahim SS, Elmaghraby TK, Hafez EE. Preventive effect of the flavonoid, quercetin, on hepatic cancer in rats via oxidant/antioxidant activity: molecular and histological evidences. J Exp Clin Cancer Res. 2009 Jun 11;28(1):80. doi: 10.1186/1756-9966-28-80[↩]
- Annapurna A, Reddy CS, Akondi RB, Rao SR. Cardioprotective actions of two bioflavonoids, quercetin and rutin, in experimental myocardial infarction in both normal and streptozotocin-induced type I diabetic rats. J Pharm Pharmacol. 2009 Oct;61(10):1365-74. doi: 10.1211/jpp/61.10.0014[↩]
- Kyle JA, Sharp L, Little J, Duthie GG, McNeill G. Dietary flavonoid intake and colorectal cancer: a case-control study. Br J Nutr. 2010 Feb;103(3):429-36. doi: 10.1017/S0007114509991784[↩]
- Wilson RT, Wang J, Chinchilli V, Richie JP, Virtamo J, Moore LE, Albanes D. Fish, vitamin D, and flavonoids in relation to renal cell cancer among smokers. Am J Epidemiol. 2009 Sep 15;170(6):717-29. doi: 10.1093/aje/kwp178[↩]
- Bobe G, Weinstein SJ, Albanes D, Hirvonen T, Ashby J, Taylor PR, Virtamo J, Stolzenberg-Solomon RZ. Flavonoid intake and risk of pancreatic cancer in male smokers (Finland). Cancer Epidemiol Biomarkers Prev. 2008 Mar;17(3):553-62. doi: 10.1158/1055-9965.EPI-07-2523[↩]
- McCann SE, Ambrosone CB, Moysich KB, Brasure J, Marshall JR, Freudenheim JL, Wilkinson GS, Graham S. Intakes of selected nutrients, foods, and phytochemicals and prostate cancer risk in western New York. Nutr Cancer. 2005;53(1):33-41. doi: 10.1207/s15327914nc5301_4[↩]
- Vijayababu MR, Arunkumar A, Kanagaraj P, Arunakaran J. Effects of quercetin on insulin-like growth factors (IGFs) and their binding protein-3 (IGFBP-3) secretion and induction of apoptosis in human prostate cancer cells. J Carcinog. 2006 Apr 6;5:10. doi: 10.1186/1477-3163-5-10[↩]
- Lam TK, Rotunno M, Lubin JH, Wacholder S, Consonni D, Pesatori AC, Bertazzi PA, Chanock SJ, Burdette L, Goldstein AM, Tucker MA, Caporaso NE, Subar AF, Landi MT. Dietary quercetin, quercetin-gene interaction, metabolic gene expression in lung tissue and lung cancer risk. Carcinogenesis. 2010 Apr;31(4):634-42. doi: 10.1093/carcin/bgp334[↩]
- Cui Y, Morgenstern H, Greenland S, Tashkin DP, Mao JT, Cai L, Cozen W, Mack TM, Lu QY, Zhang ZF. Dietary flavonoid intake and lung cancer–a population-based case-control study. Cancer. 2008 May 15;112(10):2241-8. doi: 10.1002/cncr.23398[↩]
- Egert S, Bosy-Westphal A, Seiberl J, Kürbitz C, Settler U, Plachta-Danielzik S, Wagner AE, Frank J, Schrezenmeir J, Rimbach G, Wolffram S, Müller MJ. Quercetin reduces systolic blood pressure and plasma oxidised low-density lipoprotein concentrations in overweight subjects with a high-cardiovascular disease risk phenotype: a double-blinded, placebo-controlled cross-over study. Br J Nutr. 2009 Oct;102(7):1065-74. doi: 10.1017/S0007114509359127[↩][↩]
- Li YQ, Zhou FC, Gao F, Bian JS, Shan F. Comparative evaluation of quercetin, isoquercetin and rutin as inhibitors of alpha-glucosidase. J Agric Food Chem. 2009 Dec 23;57(24):11463-8. doi: 10.1021/jf903083h[↩]
- Iris Erlund, Review of the flavonoids quercetin, hesperetin, and naringenin. Dietary sources, bioactivities, bioavailability, and epidemiology. Nutrition Research, Volume 24, Issue 10, 2004, Pages 851-874, ISSN 0271-5317, https://doi.org/10.1016/j.nutres.2004.07.005[↩]
- Nieman DC, Henson DA, Gross SJ, Jenkins DP, Davis JM, Murphy EA, Carmichael MD, Dumke CL, Utter AC, McAnulty SR, McAnulty LS, Mayer EP. Quercetin reduces illness but not immune perturbations after intensive exercise. Med Sci Sports Exerc. 2007 Sep;39(9):1561-9. doi: 10.1249/mss.0b013e318076b566[↩][↩][↩][↩]
- MacRae HS, Mefferd KM. Dietary antioxidant supplementation combined with quercetin improves cycling time trial performance. Int J Sport Nutr Exerc Metab. 2006 Aug;16(4):405-19. doi: 10.1123/ijsnem.16.4.405[↩][↩]
- Davis JM, Murphy EA, Carmichael MD, Davis B. Quercetin increases brain and muscle mitochondrial biogenesis and exercise tolerance. Am J Physiol Regul Integr Comp Physiol. 2009 Apr;296(4):R1071-7. doi: 10.1152/ajpregu.90925.2008[↩]
- Harwood M, Danielewska-Nikiel B, Borzelleca JF, Flamm GW, Williams GM, Lines TC. A critical review of the data related to the safety of quercetin and lack of evidence of in vivo toxicity, including lack of genotoxic/carcinogenic properties. Food Chem Toxicol. 2007 Nov;45(11):2179-205. doi: 10.1016/j.fct.2007.05.015[↩][↩]
- Shoskes DA, Zeitlin SI, Shahed A, Rajfer J. Quercetin in men with category III chronic prostatitis: a preliminary prospective, double-blind, placebo-controlled trial. Urology. 1999 Dec;54(6):960-3. doi: 10.1016/s0090-4295(99)00358-1[↩]
- Ferry DR, Smith A, Malkhandi J, Fyfe DW, deTakats PG, Anderson D, Baker J, Kerr DJ. Phase I clinical trial of the flavonoid quercetin: pharmacokinetics and evidence for in vivo tyrosine kinase inhibition. Clin Cancer Res. 1996 Apr;2(4):659-68.[↩]
- Okamoto T. Safety of quercetin for clinical application (Review). Int J Mol Med. 2005 Aug;16(2):275-8.[↩]
- Hendler SS, Rorvik DR, eds. PDR for Nutritional Supplements. 2nd ed: Thomson Reuters; 2008.[↩][↩][↩][↩][↩]
- Rothwell JA, Urpi-Sarda M, Boto-Ordoñez M, Llorach R, Farran-Codina A, Barupal DK, Neveu V, Manach C, Andres-Lacueva C, Scalbert A. Systematic analysis of the polyphenol metabolome using the Phenol-Explorer database. Mol Nutr Food Res. 2016 Jan;60(1):203-11. doi: 10.1002/mnfr.201500435[↩][↩]
- Lotito SB, Zhang WJ, Yang CS, Crozier A, Frei B. Metabolic conversion of dietary flavonoids alters their anti-inflammatory and antioxidant properties. Free Radic Biol Med. 2011 Jul 15;51(2):454-63. doi: 10.1016/j.freeradbiomed.2011.04.032[↩][↩]
- Williamson G. Common features in the pathways of absorption and metabolism of flavonoids. In: Meskin MS, R. BW, Davies AJ, Lewis DS, Randolph RK, eds. Phytochemicals: Mechanisms of Action. Boca Raton: CRC Press; 2004:21-33.[↩]
- Németh K, Plumb GW, Berrin JG, Juge N, Jacob R, Naim HY, Williamson G, Swallow DM, Kroon PA. Deglycosylation by small intestinal epithelial cell beta-glucosidases is a critical step in the absorption and metabolism of dietary flavonoid glycosides in humans. Eur J Nutr. 2003 Jan;42(1):29-42. doi: 10.1007/s00394-003-0397-3[↩][↩]
- Gonzales GB, Smagghe G, Grootaert C, Zotti M, Raes K, Van Camp J. Flavonoid interactions during digestion, absorption, distribution and metabolism: a sequential structure-activity/property relationship-based approach in the study of bioavailability and bioactivity. Drug Metab Rev. 2015 May;47(2):175-90. doi: 10.3109/03602532.2014.1003649[↩][↩][↩][↩][↩][↩]
- Monagas M, Urpi-Sarda M, Sánchez-Patán F, Llorach R, Garrido I, Gómez-Cordovés C, Andres-Lacueva C, Bartolomé B. Insights into the metabolism and microbial biotransformation of dietary flavan-3-ols and the bioactivity of their metabolites. Food Funct. 2010 Dec;1(3):233-53. doi: 10.1039/c0fo00132e[↩][↩]
- Roowi S, Stalmach A, Mullen W, Lean ME, Edwards CA, Crozier A. Green tea flavan-3-ols: colonic degradation and urinary excretion of catabolites by humans. J Agric Food Chem. 2010 Jan 27;58(2):1296-304. doi: 10.1021/jf9032975[↩]
- Setchell KD, Brown NM, Lydeking-Olsen E. The clinical importance of the metabolite equol-a clue to the effectiveness of soy and its isoflavones. J Nutr. 2002 Dec;132(12):3577-84. doi: 10.1093/jn/132.12.3577[↩]
- Yuan JP, Wang JH, Liu X. Metabolism of dietary soy isoflavones to equol by human intestinal microflora–implications for health. Mol Nutr Food Res. 2007 Jul;51(7):765-81. doi: 10.1002/mnfr.200600262[↩]
- Marín L, Miguélez EM, Villar CJ, Lombó F. Bioavailability of dietary polyphenols and gut microbiota metabolism: antimicrobial properties. Biomed Res Int. 2015;2015:905215. doi: 10.1155/2015/905215[↩]
- Bordenave N, Hamaker BR, Ferruzzi MG. Nature and consequences of non-covalent interactions between flavonoids and macronutrients in foods. Food Funct. 2014 Jan;5(1):18-34. doi: 10.1039/c3fo60263j[↩]
- Zhang H, Yu D, Sun J, Liu X, Jiang L, Guo H, Ren F. Interaction of plant phenols with food macronutrients: characterisation and nutritional-physiological consequences. Nutr Res Rev. 2014 Jun;27(1):1-15. doi: 10.1017/S095442241300019X[↩]
- Xiao J, Mao F, Yang F, Zhao Y, Zhang C, Yamamoto K. Interaction of dietary polyphenols with bovine milk proteins: molecular structure-affinity relationship and influencing bioactivity aspects. Mol Nutr Food Res. 2011 Nov;55(11):1637-45. doi: 10.1002/mnfr.201100280[↩]
- Lorenz M, Jochmann N, von Krosigk A, Martus P, Baumann G, Stangl K, Stangl V. Addition of milk prevents vascular protective effects of tea. Eur Heart J. 2007 Jan;28(2):219-23. doi: 10.1093/eurheartj/ehl442[↩]
- Manach C, Williamson G, Morand C, Scalbert A, Rémésy C. Bioavailability and bioefficacy of polyphenols in humans. I. Review of 97 bioavailability studies. Am J Clin Nutr. 2005 Jan;81(1 Suppl):230S-242S. doi: 10.1093/ajcn/81.1.230S[↩]
- Kroon PA, Clifford MN, Crozier A, Day AJ, Donovan JL, Manach C, Williamson G. How should we assess the effects of exposure to dietary polyphenols in vitro? Am J Clin Nutr. 2004 Jul;80(1):15-21. doi: 10.1093/ajcn/80.1.15[↩]
- Bellavia A, Larsson SC, Bottai M, Wolk A, Orsini N. Fruit and vegetable consumption and all-cause mortality: a dose-response analysis. Am J Clin Nutr. 2013 Aug;98(2):454-9. doi: 10.3945/ajcn.112.056119[↩]
- Zhang X, Shu XO, Xiang YB, Yang G, Li H, Gao J, Cai H, Gao YT, Zheng W. Cruciferous vegetable consumption is associated with a reduced risk of total and cardiovascular disease mortality. Am J Clin Nutr. 2011 Jul;94(1):240-6. doi: 10.3945/ajcn.110.009340[↩]
- Leenders M, Sluijs I, Ros MM, et al. Fruit and vegetable consumption and mortality: European prospective investigation into cancer and nutrition. Am J Epidemiol. 2013 Aug 15;178(4):590-602. doi: 10.1093/aje/kwt006[↩]
- Andersen LF, Jacobs DR Jr, Carlsen MH, Blomhoff R. Consumption of coffee is associated with reduced risk of death attributed to inflammatory and cardiovascular diseases in the Iowa Women’s Health Study. Am J Clin Nutr. 2006 May;83(5):1039-46. doi: 10.1093/ajcn/83.5.1039[↩]
- Kuriyama S, Shimazu T, Ohmori K, Kikuchi N, Nakaya N, Nishino Y, Tsubono Y, Tsuji I. Green tea consumption and mortality due to cardiovascular disease, cancer, and all causes in Japan: the Ohsaki study. JAMA. 2006 Sep 13;296(10):1255-65. doi: 10.1001/jama.296.10.1255[↩]
- Nagura J, Iso H, Watanabe Y, Maruyama K, Date C, Toyoshima H, Yamamoto A, Kikuchi S, Koizumi A, Kondo T, Wada Y, Inaba Y, Tamakoshi A; JACC Study Group. Fruit, vegetable and bean intake and mortality from cardiovascular disease among Japanese men and women: the JACC Study. Br J Nutr. 2009 Jul;102(2):285-92. doi: 10.1017/S0007114508143586[↩]
- Sahyoun NR, Jacques PF, Zhang XL, Juan W, McKeown NM. Whole-grain intake is inversely associated with the metabolic syndrome and mortality in older adults. Am J Clin Nutr. 2006 Jan;83(1):124-31. doi: 10.1093/ajcn/83.1.124[↩]
- Sauvaget C, Nagano J, Hayashi M, Spencer E, Shimizu Y, Allen N. Vegetables and fruit intake and cancer mortality in the Hiroshima/Nagasaki Life Span Study. Br J Cancer. 2003 Mar 10;88(5):689-94. doi: 10.1038/sj.bjc.6600775[↩]
- Genkinger JM, Platz EA, Hoffman SC, Comstock GW, Helzlsouer KJ. Fruit, vegetable, and antioxidant intake and all-cause, cancer, and cardiovascular disease mortality in a community-dwelling population in Washington County, Maryland. Am J Epidemiol. 2004 Dec 15;160(12):1223-33. doi: 10.1093/aje/kwh339[↩]
- Tucker KL, Hallfrisch J, Qiao N, Muller D, Andres R, Fleg JL; Baltimore Longitudinal Study of Aging. The combination of high fruit and vegetable and low saturated fat intakes is more protective against mortality in aging men than is either alone: the Baltimore Longitudinal Study of Aging. J Nutr. 2005 Mar;135(3):556-61. doi: 10.1093/jn/135.3.556[↩]
- Bazzano LA, He J, Ogden LG, Loria CM, Vupputuri S, Myers L, Whelton PK. Fruit and vegetable intake and risk of cardiovascular disease in US adults: the first National Health and Nutrition Examination Survey Epidemiologic Follow-up Study. Am J Clin Nutr. 2002 Jul;76(1):93-9. doi: 10.1093/ajcn/76.1.93[↩]
- Strandhagen E, Hansson PO, Bosaeus I, Isaksson B, Eriksson H. High fruit intake may reduce mortality among middle-aged and elderly men. The Study of Men Born in 1913. Eur J Clin Nutr. 2000 Apr;54(4):337-41. doi: 10.1038/sj.ejcn.1600959[↩]
- Rissanen TH, Voutilainen S, Virtanen JK, Venho B, Vanharanta M, Mursu J, Salonen JT. Low intake of fruits, berries and vegetables is associated with excess mortality in men: the Kuopio Ischaemic Heart Disease Risk Factor (KIHD) Study. J Nutr. 2003 Jan;133(1):199-204. doi: 10.1093/jn/133.1.199[↩]
- Brunetti C., Di Ferdinando M., Fini A., Pollastri S., Tattini M. Flavonoids as antioxidants and developmental regulators: Relative significance in plants and humans. Int. J. Mol. Sci. 2013;14:3540–3555. doi: 10.3390/ijms14023540[↩]
- Heijnen CG, Haenen GR, van Acker FA, van der Vijgh WJ, Bast A. Flavonoids as peroxynitrite scavengers: the role of the hydroxyl groups. Toxicol In Vitro. 2001 Feb;15(1):3-6. doi: 10.1016/s0887-2333(00)00053-9[↩]
- Chun OK, Kim DO, Lee CY. Superoxide radical scavenging activity of the major polyphenols in fresh plums. J Agric Food Chem. 2003 Dec 31;51(27):8067-72. doi: 10.1021/jf034740d[↩]
- Nijveldt R.J., Van Nood E., Van Hoorn D.E., Boelens P.G., Van Norren K., Van Leeuwen P.A. Flavonoids: A review of probable mechanisms of action and potential applications. Am. J. Clin. Nutr. 2001;74:418–425. doi: 10.1093/ajcn/74.4.418[↩]
- Kumar S., Pandey A.K. Chemistry and biological activities of flavonoids: An overview. Sci. World J. 2013;2013:1–16. doi: 10.1155/2013/162750[↩]
- Frei B, Higdon JV. Antioxidant activity of tea polyphenols in vivo: evidence from animal studies. J Nutr. 2003 Oct;133(10):3275S-84S. doi: 10.1093/jn/133.10.3275S[↩][↩]
- Williams RJ, Spencer JP, Rice-Evans C. Flavonoids: antioxidants or signalling molecules? Free Radic Biol Med. 2004 Apr 1;36(7):838-49. doi: 10.1016/j.freeradbiomed.2004.01.001[↩][↩][↩]
- Lotito SB, Frei B. Consumption of flavonoid-rich foods and increased plasma antioxidant capacity in humans: cause, consequence, or epiphenomenon? Free Radic Biol Med. 2006 Dec 15;41(12):1727-46. doi: 10.1016/j.freeradbiomed.2006.04.033[↩]
- Mira L, Fernandez MT, Santos M, Rocha R, Florêncio MH, Jennings KR. Interactions of flavonoids with iron and copper ions: a mechanism for their antioxidant activity. Free Radic Res. 2002 Nov;36(11):1199-208. doi: 10.1080/1071576021000016463[↩]
- Cheng IF, Breen K. On the ability of four flavonoids, baicilein, luteolin, naringenin, and quercetin, to suppress the Fenton reaction of the iron-ATP complex. Biometals. 2000 Mar;13(1):77-83. doi: 10.1023/a:1009229429250[↩]
- Cherrak S.A., Mokhtari-Soulimane N., Berroukeche F., Bensenane B., Cherbonnel A., Merzouk H., Elhabiri M. In Vitro Antioxidant versus Metal Ion Chelating Properties of Flavonoids: A Structure-Activity Investigation. PLoS ONE. 2016;11:e0165575. doi: 10.1371/journal.pone.0165575[↩]
- Leopoldini M., Russo N., Toscano M. The molecular basis of working mechanism of natural polyphenolic antioxidants. Food Chem. 2011;125:288–306. doi: 10.1016/j.foodchem.2010.08.012[↩]
- Kim EY, Ham SK, Shigenaga MK, Han O. Bioactive dietary polyphenolic compounds reduce nonheme iron transport across human intestinal cell monolayers. J Nutr. 2008 Sep;138(9):1647-51. doi: 10.1093/jn/138.9.1647[↩][↩]
- Thankachan P, Walczyk T, Muthayya S, Kurpad AV, Hurrell RF. Iron absorption in young Indian women: the interaction of iron status with the influence of tea and ascorbic acid. Am J Clin Nutr. 2008 Apr;87(4):881-6. doi: 10.1093/ajcn/87.4.881[↩][↩][↩][↩]
- Hurrell RF, Reddy M, Cook JD. Inhibition of non-haem iron absorption in man by polyphenolic-containing beverages. Br J Nutr. 1999 Apr;81(4):289-95.[↩][↩]
- Zijp IM, Korver O, Tijburg LB. Effect of tea and other dietary factors on iron absorption. Crit Rev Food Sci Nutr. 2000 Sep;40(5):371-98. doi: 10.1080/10408690091189194[↩][↩]
- Ma Q, Kim EY, Lindsay EA, Han O. Bioactive dietary polyphenols inhibit heme iron absorption in a dose-dependent manner in human intestinal Caco-2 cells. J Food Sci. 2011 Jun-Jul;76(5):H143-50. doi: 10.1111/j.1750-3841.2011.02184.x[↩][↩][↩][↩]
- Kim EY, Ham SK, Bradke D, Ma Q, Han O. Ascorbic acid offsets the inhibitory effect of bioactive dietary polyphenolic compounds on transepithelial iron transport in Caco-2 intestinal cells. J Nutr. 2011 May;141(5):828-34. doi: 10.3945/jn.110.134031[↩][↩]
- Afaq F, Mukhtar H. Botanical antioxidants in the prevention of photocarcinogenesis and photoaging. Exp Dermatol. 2006 Sep;15(9):678-84. doi: 10.1111/j.1600-0625.2006.00466.x[↩]
- Petrova A, Davids LM, Rautenbach F, Marnewick JL. Photoprotection by honeybush extracts, hesperidin and mangiferin against UVB-induced skin damage in SKH-1 mice. J Photochem Photobiol B. 2011 May 3;103(2):126-39. doi: 10.1016/j.jphotobiol.2011.02.020[↩]
- Sime S, Reeve VE. Protection from inflammation, immunosuppression and carcinogenesis induced by UV radiation in mice by topical Pycnogenol. Photochem Photobiol. 2004 Feb;79(2):193-8. doi: 10.1562/0031-8655(2004)079<0193:pfiiac>2.0.co;2[↩]
- Flavonoids and Skin Health. https://lpi.oregonstate.edu/mic/health-disease/skin-health/flavonoids[↩][↩][↩]
- Schiffer R, Neis M, Höller D, Rodríguez F, Geier A, Gartung C, Lammert F, Dreuw A, Zwadlo-Klarwasser G, Merk H, Jugert F, Baron JM. Active influx transport is mediated by members of the organic anion transporting polypeptide family in human epidermal keratinocytes. J Invest Dermatol. 2003 Feb;120(2):285-91. doi: 10.1046/j.1523-1747.2003.12031.x[↩]
- Arct J, Pytkowska K. Flavonoids as components of biologically active cosmeceuticals. Clin Dermatol. 2008 Jul-Aug;26(4):347-57. doi: 10.1016/j.clindermatol.2008.01.004[↩]
- Jackson RL, Greiwe JS, Schwen RJ. Ageing skin: oestrogen receptor β agonists offer an approach to change the outcome. Exp Dermatol. 2011 Nov;20(11):879-82. doi: 10.1111/j.1600-0625.2011.01362.x[↩]
- Pelletier G, Ren L. Localization of sex steroid receptors in human skin. Histol Histopathol. 2004 Apr;19(2):629-36. doi: 10.14670/HH-19.629[↩]
- Brand RM, Jendrzejewski JL. Topical treatment with (-)-epigallocatechin-3-gallate and genistein after a single UV exposure can reduce skin damage. J Dermatol Sci. 2008 Apr;50(1):69-72. doi: 10.1016/j.jdermsci.2007.11.008[↩]
- Widyarini S, Spinks N, Husband AJ, Reeve VE. Isoflavonoid compounds from red clover (Trifolium pratense) protect from inflammation and immune suppression induced by UV radiation. Photochem Photobiol. 2001 Sep;74(3):465-70. doi: 10.1562/0031-8655(2001)074<0465:icfrct>2.0.co;2[↩]
- Casetti F, Jung W, Wölfle U, Reuter J, Neumann K, Gilb B, Wähling A, Wagner S, Merfort I, Schempp CM. Topical application of solubilized Reseda luteola extract reduces ultraviolet B-induced inflammation in vivo. J Photochem Photobiol B. 2009 Sep 4;96(3):260-5. doi: 10.1016/j.jphotobiol.2009.07.003[↩][↩]
- Heinrich U, Moore CE, De Spirt S, Tronnier H, Stahl W. Green tea polyphenols provide photoprotection, increase microcirculation, and modulate skin properties of women. J Nutr. 2011 Jun;141(6):1202-8. doi: 10.3945/jn.110.136465[↩][↩][↩][↩][↩][↩][↩]
- Heinrich U, Neukam K, Tronnier H, Sies H, Stahl W. Long-term ingestion of high flavanol cocoa provides photoprotection against UV-induced erythema and improves skin condition in women. J Nutr. 2006 Jun;136(6):1565-9. doi: 10.1093/jn/136.6.1565[↩][↩][↩][↩]
- Neukam K, Stahl W, Tronnier H, Sies H, Heinrich U. Consumption of flavanol-rich cocoa acutely increases microcirculation in human skin. Eur J Nutr. 2007 Feb;46(1):53-6. doi: 10.1007/s00394-006-0627-6[↩][↩]
- Schroeter H, Heiss C, Balzer J, Kleinbongard P, Keen CL, Hollenberg NK, Sies H, Kwik-Uribe C, Schmitz HH, Kelm M. (-)-Epicatechin mediates beneficial effects of flavanol-rich cocoa on vascular function in humans. Proc Natl Acad Sci U S A. 2006 Jan 24;103(4):1024-9. doi: 10.1073/pnas.0510168103[↩][↩]
- Katz DL, Doughty K, Ali A. Cocoa and chocolate in human health and disease. Antioxid Redox Signal. 2011 Nov 15;15(10):2779-811. doi: 10.1089/ars.2010.3697[↩]
- Katiyar SK, Afaq F, Perez A, Mukhtar H. Green tea polyphenol (-)-epigallocatechin-3-gallate treatment of human skin inhibits ultraviolet radiation-induced oxidative stress. Carcinogenesis. 2001 Feb;22(2):287-94. doi: 10.1093/carcin/22.2.287[↩][↩]
- Katiyar SK, Matsui MS, Elmets CA, Mukhtar H. Polyphenolic antioxidant (-)-epigallocatechin-3-gallate from green tea reduces UVB-induced inflammatory responses and infiltration of leukocytes in human skin. Photochem Photobiol. 1999 Feb;69(2):148-53. https://doi.org/10.1111/j.1751-1097.1999.tb03267.x[↩][↩][↩]
- Katiyar SK, Perez A, Mukhtar H. Green tea polyphenol treatment to human skin prevents formation of ultraviolet light B-induced pyrimidine dimers in DNA. Clin Cancer Res. 2000 Oct;6(10):3864-9.[↩][↩][↩]
- Elmets CA, Singh D, Tubesing K, Matsui M, Katiyar S, Mukhtar H. Cutaneous photoprotection from ultraviolet injury by green tea polyphenols. J Am Acad Dermatol. 2001 Mar;44(3):425-32. doi: 10.1067/mjd.2001.112919[↩][↩]
- Camouse MM, Domingo DS, Swain FR, Conrad EP, Matsui MS, Maes D, Declercq L, Cooper KD, Stevens SR, Baron ED. Topical application of green and white tea extracts provides protection from solar-simulated ultraviolet light in human skin. Exp Dermatol. 2009 Jun;18(6):522-6. doi: 10.1111/j.1600-0625.2008.00818.x[↩]
- Vayalil PK, Elmets CA, Katiyar SK. Treatment of green tea polyphenols in hydrophilic cream prevents UVB-induced oxidation of lipids and proteins, depletion of antioxidant enzymes and phosphorylation of MAPK proteins in SKH-1 hairless mouse skin. Carcinogenesis. 2003 May;24(5):927-36. doi: 10.1093/carcin/bgg025. Retraction in: Carcinogenesis. 2018 May 3;39(5):738. doi: 10.1093/carcin/bgy027[↩]
- Meeran SM, Akhtar S, Katiyar SK. Inhibition of UVB-induced skin tumor development by drinking green tea polyphenols is mediated through DNA repair and subsequent inhibition of inflammation. J Invest Dermatol. 2009 May;129(5):1258-70. doi: 10.1038/jid.2008.354[↩]
- Meeran SM, Mantena SK, Elmets CA, Katiyar SK. (-)-Epigallocatechin-3-gallate prevents photocarcinogenesis in mice through interleukin-12-dependent DNA repair. Cancer Res. 2006 May 15;66(10):5512-20. doi: 10.1158/0008-5472.CAN-06-0218. Retraction in: Cancer Res. 2018 Dec 1;78(23):6709. doi: 10.1158/0008-5472.CAN-18-3092[↩]
- Meeran SM, Mantena SK, Katiyar SK. Prevention of ultraviolet radiation-induced immunosuppression by (-)-epigallocatechin-3-gallate in mice is mediated through interleukin 12-dependent DNA repair. Clin Cancer Res. 2006 Apr 1;12(7 Pt 1):2272-80. doi: 10.1158/1078-0432.CCR-05-2672[↩]
- Schwarz A, Maeda A, Gan D, Mammone T, Matsui MS, Schwarz T. Green tea phenol extracts reduce UVB-induced DNA damage in human cells via interleukin-12. Photochem Photobiol. 2008 Mar-Apr;84(2):350-5. doi: 10.1111/j.1751-1097.2007.00265.x[↩]
- Wei H, Saladi R, Lu Y, Wang Y, Palep SR, Moore J, Phelps R, Shyong E, Lebwohl MG. Isoflavone genistein: photoprotection and clinical implications in dermatology. J Nutr. 2003 Nov;133(11 Suppl 1):3811S-3819S. doi: 10.1093/jn/133.11.3811S[↩][↩][↩]
- Moore JO, Wang Y, Stebbins WG, Gao D, Zhou X, Phelps R, Lebwohl M, Wei H. Photoprotective effect of isoflavone genistein on ultraviolet B-induced pyrimidine dimer formation and PCNA expression in human reconstituted skin and its implications in dermatology and prevention of cutaneous carcinogenesis. Carcinogenesis. 2006 Aug;27(8):1627-35. doi: 10.1093/carcin/bgi367[↩]
- Katiyar SK, Korman NJ, Mukhtar H, Agarwal R. Protective effects of silymarin against photocarcinogenesis in a mouse skin model. J Natl Cancer Inst. 1997 Apr 16;89(8):556-66. doi: 10.1093/jnci/89.8.556[↩]
- Katiyar SK, Mantena SK, Meeran SM. Silymarin protects epidermal keratinocytes from ultraviolet radiation-induced apoptosis and DNA damage by nucleotide excision repair mechanism. PLoS One. 2011;6(6):e21410. doi: 10.1371/journal.pone.0021410[↩][↩]
- Cho JW, Cho SY, Lee SR, Lee KS. Onion extract and quercetin induce matrix metalloproteinase-1 in vitro and in vivo. Int J Mol Med. 2010 Mar;25(3):347-52. https://doi.org/10.3892/ijmm_00000351[↩]
- Fisher GJ, Kang S, Varani J, Bata-Csorgo Z, Wan Y, Datta S, Voorhees JJ. Mechanisms of photoaging and chronological skin aging. Arch Dermatol. 2002 Nov;138(11):1462-70. doi: 10.1001/archderm.138.11.1462[↩]
- Espley RV, Butts CA, Laing WA, Martell S, Smith H, McGhie TK, Zhang J, Paturi G, Hedderley D, Bovy A, Schouten HJ, Putterill J, Allan AC, Hellens RP. Dietary flavonoids from modified apple reduce inflammation markers and modulate gut microbiota in mice. J Nutr. 2014 Feb;144(2):146-54. doi: 10.3945/jn.113.182659[↩]
- Kim MC, Kim SJ, Kim DS, Jeon YD, Park SJ, Lee HS, Um JY, Hong SH. Vanillic acid inhibits inflammatory mediators by suppressing NF-κB in lipopolysaccharide-stimulated mouse peritoneal macrophages. Immunopharmacol Immunotoxicol. 2011 Sep;33(3):525-32. doi: 10.3109/08923973.2010.547500[↩]
- Lee SG, Kim B, Yang Y, Pham TX, Park YK, Manatou J, Koo SI, Chun OK, Lee JY. Berry anthocyanins suppress the expression and secretion of proinflammatory mediators in macrophages by inhibiting nuclear translocation of NF-κB independent of NRF2-mediated mechanism. J Nutr Biochem. 2014 Apr;25(4):404-11. doi: 10.1016/j.jnutbio.2013.12.001[↩]
- Mauray A, Felgines C, Morand C, Mazur A, Scalbert A, Milenkovic D. Bilberry anthocyanin-rich extract alters expression of genes related to atherosclerosis development in aorta of apo E-deficient mice. Nutr Metab Cardiovasc Dis. 2012 Jan;22(1):72-80. doi: 10.1016/j.numecd.2010.04.011[↩]
- Wang D, Wei X, Yan X, Jin T, Ling W. Protocatechuic acid, a metabolite of anthocyanins, inhibits monocyte adhesion and reduces atherosclerosis in apolipoprotein E-deficient mice. J Agric Food Chem. 2010 Dec 22;58(24):12722-8. doi: 10.1021/jf103427j[↩]
- Edirisinghe I, Banaszewski K, Cappozzo J, McCarthy D, Burton-Freeman BM. Effect of black currant anthocyanins on the activation of endothelial nitric oxide synthase (eNOS) in vitro in human endothelial cells. J Agric Food Chem. 2011 Aug 24;59(16):8616-24. doi: 10.1021/jf201116y. Epub 2011 Jul 28. Erratum in: J Agric Food Chem. 2013 Jan 30;61(4):1006.[↩]
- Hidalgo M, Martin-Santamaria S, Recio I, Sanchez-Moreno C, de Pascual-Teresa B, Rimbach G, de Pascual-Teresa S. Potential anti-inflammatory, anti-adhesive, anti/estrogenic, and angiotensin-converting enzyme inhibitory activities of anthocyanins and their gut metabolites. Genes Nutr. 2012 Apr;7(2):295-306. doi: 10.1007/s12263-011-0263-5[↩]
- Chen XQ, Wang XB, Guan RF, Tu J, Gong ZH, Zheng N, Yang JH, Zhang YY, Ying MM. Blood anticoagulation and antiplatelet activity of green tea (-)-epigallocatechin (EGC) in mice. Food Funct. 2013 Oct;4(10):1521-5. doi: 10.1039/c3fo60088b[↩]
- Ahmad A, Khan RM, Alkharfy KM. Effects of selected bioactive natural products on the vascular endothelium. J Cardiovasc Pharmacol. 2013 Aug;62(2):111-21. doi: 10.1097/FJC.0b013e3182927e47[↩]
- Wang X, Ouyang YY, Liu J, Zhao G. Flavonoid intake and risk of CVD: a systematic review and meta-analysis of prospective cohort studies. Br J Nutr. 2014 Jan 14;111(1):1-11. doi: 10.1017/S000711451300278X[↩]
- Wang ZM, Zhao D, Nie ZL, Zhao H, Zhou B, Gao W, Wang LS, Yang ZJ. Flavonol intake and stroke risk: a meta-analysis of cohort studies. Nutrition. 2014 May;30(5):518-23. doi: 10.1016/j.nut.2013.10.009[↩]
- Jacques PF, Cassidy A, Rogers G, Peterson JJ, Dwyer JT. Dietary flavonoid intakes and CVD incidence in the Framingham Offspring Cohort. Br J Nutr. 2015 Nov 14;114(9):1496-503. doi: 10.1017/S0007114515003141[↩][↩][↩]
- Vogiatzoglou A, Mulligan AA, Bhaniani A, Lentjes MAH, McTaggart A, Luben RN, Heiss C, Kelm M, Merx MW, Spencer JPE, Schroeter H, Khaw KT, Kuhnle GGC. Associations between flavan-3-ol intake and CVD risk in the Norfolk cohort of the European Prospective Investigation into Cancer (EPIC-Norfolk). Free Radic Biol Med. 2015 Jul;84:1-10. doi: 10.1016/j.freeradbiomed.2015.03.005[↩]
- Ried K, Sullivan TR, Fakler P, Frank OR, Stocks NP. Effect of cocoa on blood pressure. Cochrane Database Syst Rev. 2012 Aug 15;(8):CD008893. doi: 10.1002/14651858.CD008893.pub2. Update in: Cochrane Database Syst Rev. 2017 Apr 25;4:CD008893. doi: 10.1002/14651858.CD008893.pub3[↩][↩]
- Hooper L, Kay C, Abdelhamid A, Kroon PA, Cohn JS, Rimm EB, Cassidy A. Effects of chocolate, cocoa, and flavan-3-ols on cardiovascular health: a systematic review and meta-analysis of randomized trials. Am J Clin Nutr. 2012 Mar;95(3):740-51. doi: 10.3945/ajcn.111.023457[↩][↩]
- Khalesi S, Sun J, Buys N, Jamshidi A, Nikbakht-Nasrabadi E, Khosravi-Boroujeni H. Green tea catechins and blood pressure: a systematic review and meta-analysis of randomised controlled trials. Eur J Nutr. 2014 Sep;53(6):1299-311. doi: 10.1007/s00394-014-0720-1[↩]
- Guerrero L, Castillo J, Quiñones M, Garcia-Vallvé S, Arola L, Pujadas G, Muguerza B. Inhibition of angiotensin-converting enzyme activity by flavonoids: structure-activity relationship studies. PLoS One. 2012;7(11):e49493. doi: 10.1371/journal.pone.0049493[↩]
- Edwards RL, Lyon T, Litwin SE, Rabovsky A, Symons JD, Jalili T. Quercetin reduces blood pressure in hypertensive subjects. J Nutr. 2007 Nov;137(11):2405-11. doi: 10.1093/jn/137.11.2405[↩]
- Zahedi M, Ghiasvand R, Feizi A, Asgari G, Darvish L. Does Quercetin Improve Cardiovascular Risk factors and Inflammatory Biomarkers in Women with Type 2 Diabetes: A Double-blind Randomized Controlled Clinical Trial. Int J Prev Med. 2013 Jul;4(7):777-85. https://www.ncbi.nlm.nih.gov/pmc/articles/PMC3775217[↩]
- Brüll V, Burak C, Stoffel-Wagner B, Wolffram S, Nickenig G, Müller C, Langguth P, Alteheld B, Fimmers R, Naaf S, Zimmermann BF, Stehle P, Egert S. Effects of a quercetin-rich onion skin extract on 24 h ambulatory blood pressure and endothelial function in overweight-to-obese patients with (pre-)hypertension: a randomised double-blinded placebo-controlled cross-over trial. Br J Nutr. 2015 Oct 28;114(8):1263-77. doi: 10.1017/S0007114515002950[↩][↩]
- Cassidy A, Rogers G, Peterson JJ, Dwyer JT, Lin H, Jacques PF. Higher dietary anthocyanin and flavonol intakes are associated with anti-inflammatory effects in a population of US adults. Am J Clin Nutr. 2015 Jul;102(1):172-81. doi: 10.3945/ajcn.115.108555[↩][↩]
- Grassi D, Desideri G, Ferri C. Protective effects of dark chocolate on endothelial function and diabetes. Curr Opin Clin Nutr Metab Care. 2013 Nov;16(6):662-8. doi: 10.1097/MCO.0b013e3283659a51[↩]
- Sansone R, Rodriguez-Mateos A, Heuel J, Falk D, Schuler D, Wagstaff R, Kuhnle GG, Spencer JP, Schroeter H, Merx MW, Kelm M, Heiss C; Flaviola Consortium, European Union 7th Framework Program. Cocoa flavanol intake improves endothelial function and Framingham Risk Score in healthy men and women: a randomised, controlled, double-masked trial: the Flaviola Health Study. Br J Nutr. 2015 Oct 28;114(8):1246-55. doi: 10.1017/S0007114515002822[↩]
- Basu A, Fu DX, Wilkinson M, Simmons B, Wu M, Betts NM, Du M, Lyons TJ. Strawberries decrease atherosclerotic markers in subjects with metabolic syndrome. Nutr Res. 2010 Jul;30(7):462-9. doi: 10.1016/j.nutres.2010.06.016[↩]
- Kelley DS, Rasooly R, Jacob RA, Kader AA, Mackey BE. Consumption of Bing sweet cherries lowers circulating concentrations of inflammation markers in healthy men and women. J Nutr. 2006 Apr;136(4):981-6. doi: 10.1093/jn/136.4.981[↩]
- Moazen S, Amani R, Homayouni Rad A, Shahbazian H, Ahmadi K, Taha Jalali M. Effects of freeze-dried strawberry supplementation on metabolic biomarkers of atherosclerosis in subjects with type 2 diabetes: a randomized double-blind controlled trial. Ann Nutr Metab. 2013;63(3):256-64. doi: 10.1159/000356053[↩]
- Edirisinghe I, Banaszewski K, Cappozzo J, Sandhya K, Ellis CL, Tadapaneni R, Kappagoda CT, Burton-Freeman BM. Strawberry anthocyanin and its association with postprandial inflammation and insulin. Br J Nutr. 2011 Sep;106(6):913-22. doi: 10.1017/S0007114511001176[↩]
- Karlsen A, Retterstøl L, Laake P, Paur I, Bøhn SK, Sandvik L, Blomhoff R. Anthocyanins inhibit nuclear factor-kappaB activation in monocytes and reduce plasma concentrations of pro-inflammatory mediators in healthy adults. J Nutr. 2007 Aug;137(8):1951-4. doi: 10.1093/jn/137.8.1951[↩]
- Basu A, Lyons TJ. Strawberries, blueberries, and cranberries in the metabolic syndrome: clinical perspectives. J Agric Food Chem. 2012 Jun 13;60(23):5687-92. doi: 10.1021/jf203488k[↩][↩]
- Zhu Y, Ling W, Guo H, Song F, Ye Q, Zou T, Li D, Zhang Y, Li G, Xiao Y, Liu F, Li Z, Shi Z, Yang Y. Anti-inflammatory effect of purified dietary anthocyanin in adults with hypercholesterolemia: a randomized controlled trial. Nutr Metab Cardiovasc Dis. 2013 Sep;23(9):843-9. doi: 10.1016/j.numecd.2012.06.005[↩]
- Qin Y, Xia M, Ma J, Hao Y, Liu J, Mou H, Cao L, Ling W. Anthocyanin supplementation improves serum LDL- and HDL-cholesterol concentrations associated with the inhibition of cholesteryl ester transfer protein in dyslipidemic subjects. Am J Clin Nutr. 2009 Sep;90(3):485-92. doi: 10.3945/ajcn.2009.27814[↩]
- Zhu Y, Huang X, Zhang Y, Wang Y, Liu Y, Sun R, Xia M. Anthocyanin supplementation improves HDL-associated paraoxonase 1 activity and enhances cholesterol efflux capacity in subjects with hypercholesterolemia. J Clin Endocrinol Metab. 2014 Feb;99(2):561-9. doi: 10.1210/jc.2013-2845[↩]
- Curtis PJ, Kroon PA, Hollands WJ, Walls R, Jenkins G, Kay CD, Cassidy A. Cardiovascular disease risk biomarkers and liver and kidney function are not altered in postmenopausal women after ingesting an elderberry extract rich in anthocyanins for 12 weeks. J Nutr. 2009 Dec;139(12):2266-71. doi: 10.3945/jn.109.113126[↩]
- Grassi D, Desideri G, Di Giosia P, De Feo M, Fellini E, Cheli P, Ferri L, Ferri C. Tea, flavonoids, and cardiovascular health: endothelial protection. Am J Clin Nutr. 2013 Dec;98(6 Suppl):1660S-1666S. doi: 10.3945/ajcn.113.058313[↩]
- Förstermann U, Sessa WC. Nitric oxide synthases: regulation and function. Eur Heart J. 2012 Apr;33(7):829-37, 837a-837d. doi: 10.1093/eurheartj/ehr304[↩][↩]
- Ras RT, Streppel MT, Draijer R, Zock PL. Flow-mediated dilation and cardiovascular risk prediction: a systematic review with meta-analysis. Int J Cardiol. 2013 Sep 20;168(1):344-51. doi: 10.1016/j.ijcard.2012.09.047[↩]
- Liu Y, Li D, Zhang Y, Sun R, Xia M. Anthocyanin increases adiponectin secretion and protects against diabetes-related endothelial dysfunction. Am J Physiol Endocrinol Metab. 2014 Apr 15;306(8):E975-88. doi: 10.1152/ajpendo.00699.2013[↩][↩][↩]
- Zhu Y, Xia M, Yang Y, Liu F, Li Z, Hao Y, Mi M, Jin T, Ling W. Purified anthocyanin supplementation improves endothelial function via NO-cGMP activation in hypercholesterolemic individuals. Clin Chem. 2011 Nov;57(11):1524-33. doi: 10.1373/clinchem.2011.167361[↩]
- Ras RT, Zock PL, Draijer R. Tea consumption enhances endothelial-dependent vasodilation; a meta-analysis. PLoS One. 2011 Mar 4;6(3):e16974. doi: 10.1371/journal.pone.0016974[↩]
- Grassi D, Necozione S, Lippi C, Croce G, Valeri L, Pasqualetti P, Desideri G, Blumberg JB, Ferri C. Cocoa reduces blood pressure and insulin resistance and improves endothelium-dependent vasodilation in hypertensives. Hypertension. 2005 Aug;46(2):398-405. doi: 10.1161/01.HYP.0000174990.46027.70[↩]
- Grassi D, Desideri G, Necozione S, Ruggieri F, Blumberg JB, Stornello M, Ferri C. Protective effects of flavanol-rich dark chocolate on endothelial function and wave reflection during acute hyperglycemia. Hypertension. 2012 Sep;60(3):827-32. doi: 10.1161/HYPERTENSIONAHA.112.193995[↩]
- Davison K, Coates AM, Buckley JD, Howe PR. Effect of cocoa flavanols and exercise on cardiometabolic risk factors in overweight and obese subjects. Int J Obes (Lond). 2008 Aug;32(8):1289-96. doi: 10.1038/ijo.2008.66[↩]
- West SG, McIntyre MD, Piotrowski MJ, Poupin N, Miller DL, Preston AG, Wagner P, Groves LF, Skulas-Ray AC. Effects of dark chocolate and cocoa consumption on endothelial function and arterial stiffness in overweight adults. Br J Nutr. 2014 Feb;111(4):653-61. doi: 10.1017/S0007114513002912[↩]
- Flammer AJ, Sudano I, Wolfrum M, Thomas R, Enseleit F, Périat D, Kaiser P, Hirt A, Hermann M, Serafini M, Lévêques A, Lüscher TF, Ruschitzka F, Noll G, Corti R. Cardiovascular effects of flavanol-rich chocolate in patients with heart failure. Eur Heart J. 2012 Sep;33(17):2172-80. doi: 10.1093/eurheartj/ehr448[↩]
- Gómez-Guzmán M, Jiménez R, Sánchez M, Zarzuelo MJ, Galindo P, Quintela AM, López-Sepúlveda R, Romero M, Tamargo J, Vargas F, Pérez-Vizcaíno F, Duarte J. Epicatechin lowers blood pressure, restores endothelial function, and decreases oxidative stress and endothelin-1 and NADPH oxidase activity in DOCA-salt hypertension. Free Radic Biol Med. 2012 Jan 1;52(1):70-9. doi: 10.1016/j.freeradbiomed.2011.09.015[↩]
- Bachmair EM, Ostertag LM, Zhang X, de Roos B. Dietary manipulation of platelet function. Pharmacol Ther. 2014 Nov;144(2):97-113. doi: 10.1016/j.pharmthera.2014.05.008[↩][↩]
- Pearson DA, Paglieroni TG, Rein D, Wun T, Schramm DD, Wang JF, Holt RR, Gosselin R, Schmitz HH, Keen CL. The effects of flavanol-rich cocoa and aspirin on ex vivo platelet function. Thromb Res. 2002 May 15;106(4-5):191-7. doi: 10.1016/s0049-3848(02)00128-7[↩]
- Centers for Disease Control and Prevention CDC. National diabetes fact sheet: general information and national estimates on diabetes in the united states. Atlanta, GA: U.S. Department of health and human services, centre for disease control and prevention; 2008.[↩]
- Yang W, Lu J, Weng J, Jia W, Ji L, Xiao J, Shan Z, Liu J, Tian H, Ji Q, Zhu D, Ge J, Lin L, Chen L, Guo X, Zhao Z, Li Q, Zhou Z, Shan G, He J; China National Diabetes and Metabolic Disorders Study Group. Prevalence of diabetes among men and women in China. N Engl J Med. 2010 Mar 25;362(12):1090-101. doi: 10.1056/NEJMoa0908292[↩]
- Gillies CL, Abrams KR, Lambert PC, Cooper NJ, Sutton AJ, Hsu RT, Khunti K. Pharmacological and lifestyle interventions to prevent or delay type 2 diabetes in people with impaired glucose tolerance: systematic review and meta-analysis. BMJ. 2007 Feb 10;334(7588):299. doi: 10.1136/bmj.39063.689375.55[↩]
- Salas-Salvadó J, Bulló M, Babio N, Martínez-González MÁ, Ibarrola-Jurado N, Basora J, Estruch R, Covas MI, Corella D, Arós F, Ruiz-Gutiérrez V, Ros E; PREDIMED Study Investigators. Reduction in the incidence of type 2 diabetes with the Mediterranean diet: results of the PREDIMED-Reus nutrition intervention randomized trial. Diabetes Care. 2011 Jan;34(1):14-9. doi: 10.2337/dc10-1288. Epub 2010 Oct 7. Erratum in: Diabetes Care. 2018 Oct;41(10):2259-2260. doi: 10.2337/dc18-er10[↩][↩]
- Oberley LW. Free radicals and diabetes. Free Radic Biol Med. 1988;5(2):113-24. doi: 10.1016/0891-5849(88)90036-6[↩]
- Asmat U, Abad K, Ismail K. Diabetes mellitus and oxidative stress-A concise review. Saudi Pharm J. 2016 Sep;24(5):547-553. doi: 10.1016/j.jsps.2015.03.013[↩]
- Wang PY, Fang JC, Gao ZH, Zhang C, Xie SY. Higher intake of fruits, vegetables or their fiber reduces the risk of type 2 diabetes: A meta-analysis. J Diabetes Investig. 2016 Jan;7(1):56-69. doi: 10.1111/jdi.12376[↩]
- Meinilä J, Valkama A, Koivusalo SB, Stach-Lempinen B, Lindström J, Kautiainen H, Eriksson JG, Erkkola M. Healthy Food Intake Index (HFII) – Validity and reproducibility in a gestational-diabetes-risk population. BMC Public Health. 2016 Jul 30;16:680. doi: 10.1186/s12889-016-3303-7[↩][↩]
- Tuomilehto J, Hu G, Bidel S, Lindström J, Jousilahti P. Coffee consumption and risk of type 2 diabetes mellitus among middle-aged Finnish men and women. JAMA. 2004 Mar 10;291(10):1213-9. doi: 10.1001/jama.291.10.1213[↩][↩]
- Huxley R, Lee CM, Barzi F, Timmermeister L, Czernichow S, Perkovic V, Grobbee DE, Batty D, Woodward M. Coffee, decaffeinated coffee, and tea consumption in relation to incident type 2 diabetes mellitus: a systematic review with meta-analysis. Arch Intern Med. 2009 Dec 14;169(22):2053-63. doi: 10.1001/archinternmed.2009.439[↩]
- Knekt P, Kumpulainen J, Järvinen R, Rissanen H, Heliövaara M, Reunanen A, Hakulinen T, Aromaa A. Flavonoid intake and risk of chronic diseases. Am J Clin Nutr. 2002 Sep;76(3):560-8. doi: 10.1093/ajcn/76.3.560[↩]
- Rosengren A, Dotevall A, Wilhelmsen L, Thelle D, Johansson S. Coffee and incidence of diabetes in Swedish women: a prospective 18-year follow-up study. J Intern Med. 2004 Jan;255(1):89-95. doi: 10.1046/j.1365-2796.2003.01260.x[↩]
- Iso H, Date C, Wakai K, Fukui M, Tamakoshi A; JACC Study Group. The relationship between green tea and total caffeine intake and risk for self-reported type 2 diabetes among Japanese adults. Ann Intern Med. 2006 Apr 18;144(8):554-62. doi: 10.7326/0003-4819-144-8-200604180-00005[↩]
- Saeed M, Naveed M, Arif M, Kakar MU, Manzoor R, Abd El-Hack ME, Alagawany M, Tiwari R, Khandia R, Munjal A, Karthik K, Dhama K, Iqbal HMN, Dadar M, Sun C. Green tea (Camellia sinensis) and l-theanine: Medicinal values and beneficial applications in humans-A comprehensive review. Biomed Pharmacother. 2017 Nov;95:1260-1275. doi: 10.1016/j.biopha.2017.09.024[↩]
- Satoh T, Igarashi M, Yamada S, Takahashi N, Watanabe K. Inhibitory effect of black tea and its combination with acarbose on small intestinal α-glucosidase activity. J Ethnopharmacol. 2015 Feb 23;161:147-55. doi: 10.1016/j.jep.2014.12.009[↩]
- El-Demerdash FM, Yousef MI, El-Naga NI. Biochemical study on the hypoglycemic effects of onion and garlic in alloxan-induced diabetic rats. Food Chem Toxicol. 2005 Jan;43(1):57-63. doi: 10.1016/j.fct.2004.08.012[↩]
- Hanhineva K, Törrönen R, Bondia-Pons I, Pekkinen J, Kolehmainen M, Mykkänen H, Poutanen K. Impact of dietary polyphenols on carbohydrate metabolism. Int J Mol Sci. 2010 Mar 31;11(4):1365-402. doi: 10.3390/ijms11041365[↩]
- Babu PV, Liu D, Gilbert ER. Recent advances in understanding the anti-diabetic actions of dietary flavonoids. J Nutr Biochem. 2013 Nov;24(11):1777-89. doi: 10.1016/j.jnutbio.2013.06.003[↩]
- Zamora-Ros R, Forouhi NG, Sharp SJ, González CA, et al. The association between dietary flavonoid and lignan intakes and incident type 2 diabetes in European populations: the EPIC-InterAct study. Diabetes Care. 2013 Dec;36(12):3961-70. doi: 10.2337/dc13-0877[↩][↩][↩]
- Zamora-Ros R, Forouhi NG, Sharp SJ, et al. Dietary intakes of individual flavanols and flavonols are inversely associated with incident type 2 diabetes in European populations. J Nutr. 2014 Mar;144(3):335-43. doi: 10.3945/jn.113.184945[↩][↩]
- Wang X, Tian J, Jiang J, Li L, Ying X, Tian H, Nie M. Effects of green tea or green tea extract on insulin sensitivity and glycaemic control in populations at risk of type 2 diabetes mellitus: a systematic review and meta-analysis of randomised controlled trials. J Hum Nutr Diet. 2014 Oct;27(5):501-12. doi: 10.1111/jhn.12181[↩]
- Liu K, Zhou R, Wang B, Chen K, Shi LY, Zhu JD, Mi MT. Effect of green tea on glucose control and insulin sensitivity: a meta-analysis of 17 randomized controlled trials. Am J Clin Nutr. 2013 Aug;98(2):340-8. doi: 10.3945/ajcn.112.052746[↩]
- Zheng XX, Xu YL, Li SH, Hui R, Wu YJ, Huang XH. Effects of green tea catechins with or without caffeine on glycemic control in adults: a meta-analysis of randomized controlled trials. Am J Clin Nutr. 2013 Apr;97(4):750-62. doi: 10.3945/ajcn.111.032573[↩]
- Grassi D, Desideri G, Necozione S, Lippi C, Casale R, Properzi G, Blumberg JB, Ferri C. Blood pressure is reduced and insulin sensitivity increased in glucose-intolerant, hypertensive subjects after 15 days of consuming high-polyphenol dark chocolate. J Nutr. 2008 Sep;138(9):1671-6. doi: 10.1093/jn/138.9.1671[↩]
- Curtis PJ, Sampson M, Potter J, Dhatariya K, Kroon PA, Cassidy A. Chronic ingestion of flavan-3-ols and isoflavones improves insulin sensitivity and lipoprotein status and attenuates estimated 10-year CVD risk in medicated postmenopausal women with type 2 diabetes: a 1-year, double-blind, randomized, controlled trial. Diabetes Care. 2012 Feb;35(2):226-32. doi: 10.2337/dc11-1443[↩]
- Wedick NM, Pan A, Cassidy A, Rimm EB, Sampson L, Rosner B, Willett W, Hu FB, Sun Q, van Dam RM. Dietary flavonoid intakes and risk of type 2 diabetes in US men and women. Am J Clin Nutr. 2012 Apr;95(4):925-33. doi: 10.3945/ajcn.111.028894[↩]
- Hokayem M, Blond E, Vidal H, Lambert K, Meugnier E, Feillet-Coudray C, Coudray C, Pesenti S, Luyton C, Lambert-Porcheron S, Sauvinet V, Fedou C, Brun JF, Rieusset J, Bisbal C, Sultan A, Mercier J, Goudable J, Dupuy AM, Cristol JP, Laville M, Avignon A. Grape polyphenols prevent fructose-induced oxidative stress and insulin resistance in first-degree relatives of type 2 diabetic patients. Diabetes Care. 2013 Jun;36(6):1454-61. doi: 10.2337/dc12-1652[↩]
- Soltani R, Gorji A, Asgary S, Sarrafzadegan N, Siavash M. Evaluation of the Effects of Cornus mas L. Fruit Extract on Glycemic Control and Insulin Level in Type 2 Diabetic Adult Patients: A Randomized Double-Blind Placebo-Controlled Clinical Trial. Evid Based Complement Alternat Med. 2015;2015:740954. doi: 10.1155/2015/740954[↩]
- Li D, Zhang Y, Liu Y, Sun R, Xia M. Purified anthocyanin supplementation reduces dyslipidemia, enhances antioxidant capacity, and prevents insulin resistance in diabetic patients. J Nutr. 2015 Apr;145(4):742-8. doi: 10.3945/jn.114.205674[↩]
- Sokolov AN, Pavlova MA, Klosterhalfen S, Enck P. Chocolate and the brain: neurobiological impact of cocoa flavanols on cognition and behavior. Neurosci Biobehav Rev. 2013 Dec;37(10 Pt 2):2445-53. doi: 10.1016/j.neubiorev.2013.06.013[↩][↩]
- Vauzour D, Vafeiadou K, Rodriguez-Mateos A, Rendeiro C, Spencer JP. The neuroprotective potential of flavonoids: a multiplicity of effects. Genes Nutr. 2008 Dec;3(3-4):115-26. doi: 10.1007/s12263-008-0091-4[↩][↩][↩]
- Ramassamy C. Emerging role of polyphenolic compounds in the treatment of neurodegenerative diseases: a review of their intracellular targets. Eur J Pharmacol. 2006 Sep 1;545(1):51-64. doi: 10.1016/j.ejphar.2006.06.025[↩]
- Nurk E, Refsum H, Drevon CA, Tell GS, Nygaard HA, Engedal K, Smith AD. Intake of flavonoid-rich wine, tea, and chocolate by elderly men and women is associated with better cognitive test performance. J Nutr. 2009 Jan;139(1):120-7. doi: 10.3945/jn.108.095182[↩]
- Commenges D, Scotet V, Renaud S, Jacqmin-Gadda H, Barberger-Gateau P, Dartigues JF. Intake of flavonoids and risk of dementia. Eur J Epidemiol. 2000 Apr;16(4):357-63. doi: 10.1023/a:1007614613771[↩]
- Letenneur L, Proust-Lima C, Le Gouge A, Dartigues JF, Barberger-Gateau P. Flavonoid intake and cognitive decline over a 10-year period. Am J Epidemiol. 2007 Jun 15;165(12):1364-71. doi: 10.1093/aje/kwm036[↩][↩]
- Desideri G, Kwik-Uribe C, Grassi D, Necozione S, Ghiadoni L, Mastroiacovo D, Raffaele A, Ferri L, Bocale R, Lechiara MC, Marini C, Ferri C. Benefits in cognitive function, blood pressure, and insulin resistance through cocoa flavanol consumption in elderly subjects with mild cognitive impairment: the Cocoa, Cognition, and Aging (CoCoA) study. Hypertension. 2012 Sep;60(3):794-801. doi: 10.1161/HYPERTENSIONAHA.112.193060[↩][↩]
- Mastroiacovo D, Kwik-Uribe C, Grassi D, Necozione S, Raffaele A, Pistacchio L, Righetti R, Bocale R, Lechiara MC, Marini C, Ferri C, Desideri G. Cocoa flavanol consumption improves cognitive function, blood pressure control, and metabolic profile in elderly subjects: the Cocoa, Cognition, and Aging (CoCoA) Study–a randomized controlled trial. Am J Clin Nutr. 2015 Mar;101(3):538-48. doi: 10.3945/ajcn.114.092189[↩]
- Sorond FA, Lipsitz LA, Hollenberg NK, Fisher ND. Cerebral blood flow response to flavanol-rich cocoa in healthy elderly humans. Neuropsychiatr Dis Treat. 2008 Apr;4(2):433-40. https://www.ncbi.nlm.nih.gov/pmc/articles/PMC2518374[↩]
- Crews WD Jr, Harrison DW, Wright JW. A double-blind, placebo-controlled, randomized trial of the effects of dark chocolate and cocoa on variables associated with neuropsychological functioning and cardiovascular health: clinical findings from a sample of healthy, cognitively intact older adults. Am J Clin Nutr. 2008 Apr;87(4):872-80. doi: 10.1093/ajcn/87.4.872[↩]
- Massee LA, Ried K, Pase M, Travica N, Yoganathan J, Scholey A, Macpherson H, Kennedy G, Sali A, Pipingas A. The acute and sub-chronic effects of cocoa flavanols on mood, cognitive and cardiovascular health in young healthy adults: a randomized, controlled trial. Front Pharmacol. 2015 May 20;6:93. doi: 10.3389/fphar.2015.00093[↩]
- Pase MP, Scholey AB, Pipingas A, Kras M, Nolidin K, Gibbs A, Wesnes K, Stough C. Cocoa polyphenols enhance positive mood states but not cognitive performance: a randomized, placebo-controlled trial. J Psychopharmacol. 2013 May;27(5):451-8. doi: 10.1177/0269881112473791[↩]
- Scholey AB, French SJ, Morris PJ, Kennedy DO, Milne AL, Haskell CF. Consumption of cocoa flavanols results in acute improvements in mood and cognitive performance during sustained mental effort. J Psychopharmacol. 2010 Oct;24(10):1505-14. doi: 10.1177/0269881109106923[↩]
- Kent K, Charlton K, Roodenrys S, Batterham M, Potter J, Traynor V, Gilbert H, Morgan O, Richards R. Consumption of anthocyanin-rich cherry juice for 12 weeks improves memory and cognition in older adults with mild-to-moderate dementia. Eur J Nutr. 2017 Feb;56(1):333-341. doi: 10.1007/s00394-015-1083-y[↩]
- Alharbi MH, Lamport DJ, Dodd GF, Saunders C, Harkness L, Butler LT, Spencer JP. Flavonoid-rich orange juice is associated with acute improvements in cognitive function in healthy middle-aged males. Eur J Nutr. 2016 Sep;55(6):2021-9. doi: 10.1007/s00394-015-1016-9[↩]
- Kean RJ, Lamport DJ, Dodd GF, Freeman JE, Williams CM, Ellis JA, Butler LT, Spencer JP. Chronic consumption of flavanone-rich orange juice is associated with cognitive benefits: an 8-wk, randomized, double-blind, placebo-controlled trial in healthy older adults. Am J Clin Nutr. 2015 Mar;101(3):506-14. doi: 10.3945/ajcn.114.088518[↩]
- Casini ML, Marelli G, Papaleo E, Ferrari A, D’Ambrosio F, Unfer V. Psychological assessment of the effects of treatment with phytoestrogens on postmenopausal women: a randomized, double-blind, crossover, placebo-controlled study. Fertil Steril. 2006 Apr;85(4):972-8. doi: 10.1016/j.fertnstert.2005.09.048[↩]
- Kritz-Silverstein D, Von Mühlen D, Barrett-Connor E, Bressel MA. Isoflavones and cognitive function in older women: the SOy and Postmenopausal Health In Aging (SOPHIA) Study. Menopause. 2003 May-Jun;10(3):196-202. doi: 10.1097/00042192-200310030-00004[↩]
- Holland TM, Agarwal P, Wang Y, Leurgans SE, Bennett DA, Booth SL, Morris MC. Dietary flavonols and risk of Alzheimer dementia. Neurology. 2020 Apr 21;94(16):e1749-e1756. doi: 10.1212/WNL.0000000000008981[↩]
- Devore EE, Kang JH, Breteler MM, Grodstein F. Dietary intakes of berries and flavonoids in relation to cognitive decline. Ann Neurol. 2012 Jul;72(1):135-43. doi: 10.1002/ana.23594[↩]
- Delgado ME, Haza AI, Arranz N, García A, Morales P. Dietary polyphenols protect against N-nitrosamines and benzo(a)pyrene-induced DNA damage (strand breaks and oxidized purines/pyrimidines) in HepG2 human hepatoma cells. Eur J Nutr. 2008 Dec;47(8):479-90. doi: 10.1007/s00394-008-0751-6[↩]
- Erba D, Casiraghi MC, Martinez-Conesa C, Goi G, Massaccesi L. Isoflavone supplementation reduces DNA oxidative damage and increases O-β-N-acetyl-D-glucosaminidase activity in healthy women. Nutr Res. 2012 Apr;32(4):233-40. doi: 10.1016/j.nutres.2012.03.007[↩]
- Moon YJ, Wang X, Morris ME. Dietary flavonoids: effects on xenobiotic and carcinogen metabolism. Toxicol In Vitro. 2006 Mar;20(2):187-210. doi: 10.1016/j.tiv.2005.06.048[↩]
- Schwarz D, Kisselev P, Roots I. CYP1A1 genotype-selective inhibition of benzo[a]pyrene activation by quercetin. Eur J Cancer. 2005 Jan;41(1):151-8. doi: 10.1016/j.ejca.2004.08.011[↩]
- Suh Y, Afaq F, Johnson JJ, Mukhtar H. A plant flavonoid fisetin induces apoptosis in colon cancer cells by inhibition of COX2 and Wnt/EGFR/NF-kappaB-signaling pathways. Carcinogenesis. 2009 Feb;30(2):300-7. doi: 10.1093/carcin/bgn269[↩]
- Ravishankar D, Watson KA, Boateng SY, Green RJ, Greco F, Osborn HM. Exploring quercetin and luteolin derivatives as antiangiogenic agents. Eur J Med Chem. 2015 Jun 5;97:259-74. doi: 10.1016/j.ejmech.2015.04.056[↩]
- Santos BL, Oliveira MN, Coelho PL, Pitanga BP, da Silva AB, Adelita T, Silva VD, Costa Mde F, El-Bachá RS, Tardy M, Chneiweiss H, Junier MP, Moura-Neto V, Costa SL. Flavonoids suppress human glioblastoma cell growth by inhibiting cell metabolism, migration, and by regulating extracellular matrix proteins and metalloproteinases expression. Chem Biol Interact. 2015 Dec 5;242:123-38. doi: 10.1016/j.cbi.2015.07.014[↩]
- Devi K.P., Rajavel T., Nabavi S.F., Setzer W.N., Ahmadi A., Mansouri K., Nabavi S.M. Hesperidin: A promising anticancer agent from nature. Ind. Crops Prod. 2015;76:582–589. doi: 10.1016/j.indcrop.2015.07.051[↩]
- Ersoz M., Erdemir A., Duranoglu D., Uzunoglu D., Arasoglu T., Derman S., Mansuroglu B. Comparative evaluation of hesperetin loaded nanoparticles for anticancer activity against C6 glioma cancer cells. Artificial Cells Nanomed. Biotechnol. 2019;47:319–329. doi: 10.1080/21691401.2018.1556213[↩]
- Alsayari A., Muhsinah A.B., Hassan M.Z., Ahsan M.J., Alshehri J.A., Begum N. Aurone: A biologically attractive scaffold as anticancer agent. European J. Med. Chem. 2019;166:417–431. doi: 10.1016/j.ejmech.2019.01.078[↩][↩]
- Romagnolo DF, Selmin OI. Flavonoids and cancer prevention: a review of the evidence. J Nutr Gerontol Geriatr. 2012;31(3):206-38. doi: 10.1080/21551197.2012.702534[↩]
- Woo HD, Kim J. Dietary flavonoid intake and risk of stomach and colorectal cancer. World J Gastroenterol. 2013 Feb 21;19(7):1011-9. doi: 10.3748/wjg.v19.i7.1011[↩]
- Nimptsch K, Zhang X, Cassidy A, Song M, O’Reilly ÉJ, Lin JH, Pischon T, Rimm EB, Willett WC, Fuchs CS, Ogino S, Chan AT, Giovannucci EL, Wu K. Habitual intake of flavonoid subclasses and risk of colorectal cancer in 2 large prospective cohorts. Am J Clin Nutr. 2016 Jan;103(1):184-91. doi: 10.3945/ajcn.115.117507[↩]
- Woo HD, Kim J. Dietary flavonoid intake and smoking-related cancer risk: a meta-analysis. PLoS One. 2013 Sep 19;8(9):e75604. doi: 10.1371/journal.pone.0075604[↩][↩]
- Tang NP, Zhou B, Wang B, Yu RB, Ma J. Flavonoids intake and risk of lung cancer: a meta-analysis. Jpn J Clin Oncol. 2009 Jun;39(6):352-9. doi: 10.1093/jjco/hyp028[↩]
- Ollberding NJ, Lim U, Wilkens LR, Setiawan VW, Shvetsov YB, Henderson BE, Kolonel LN, Goodman MT. Legume, soy, tofu, and isoflavone intake and endometrial cancer risk in postmenopausal women in the multiethnic cohort study. J Natl Cancer Inst. 2012 Jan 4;104(1):67-76. doi: 10.1093/jnci/djr475[↩]
- Bandera EV, King M, Chandran U, Paddock LE, Rodriguez-Rodriguez L, Olson SH. Phytoestrogen consumption from foods and supplements and epithelial ovarian cancer risk: a population-based case control study. BMC Womens Health. 2011 Sep 23;11:40. doi: 10.1186/1472-6874-11-40[↩]
- Cassidy A, Huang T, Rice MS, Rimm EB, Tworoger SS. Intake of dietary flavonoids and risk of epithelial ovarian cancer. Am J Clin Nutr. 2014 Nov;100(5):1344-51. doi: 10.3945/ajcn.114.088708[↩]
- Gates MA, Vitonis AF, Tworoger SS, Rosner B, Titus-Ernstoff L, Hankinson SE, Cramer DW. Flavonoid intake and ovarian cancer risk in a population-based case-control study. Int J Cancer. 2009 Apr 15;124(8):1918-25. doi: 10.1002/ijc.24151[↩]
- Rossi M, Negri E, Lagiou P, Talamini R, Dal Maso L, Montella M, Franceschi S, La Vecchia C. Flavonoids and ovarian cancer risk: A case-control study in Italy. Int J Cancer. 2008 Aug 15;123(4):895-8. doi: 10.1002/ijc.23549[↩]
- Ko KP. Isoflavones: chemistry, analysis, functions and effects on health and cancer. Asian Pac J Cancer Prev. 2014;15(17):7001-10. doi: 10.7314/apjcp.2014.15.17.7001[↩]
- Dong JY, Qin LQ. Soy isoflavones consumption and risk of breast cancer incidence or recurrence: a meta-analysis of prospective studies. Breast Cancer Res Treat. 2011 Jan;125(2):315-23. doi: 10.1007/s10549-010-1270-8[↩][↩]
- Iwasaki M, Hamada GS, Nishimoto IN, Netto MM, Motola J Jr, Laginha FM, Kasuga Y, Yokoyama S, Onuma H, Nishimura H, Kusama R, Kobayashi M, Ishihara J, Yamamoto S, Hanaoka T, Tsugane S. Isoflavone, polymorphisms in estrogen receptor genes and breast cancer risk in case-control studies in Japanese, Japanese Brazilians and non-Japanese Brazilians. Cancer Sci. 2009 May;100(5):927-33. doi: 10.1111/j.1349-7006.2009.01118.x[↩]
- Wang Q, Li H, Tao P, Wang YP, Yuan P, Yang CX, Li JY, Yang F, Lee H, Huang Y. Soy isoflavones, CYP1A1, CYP1B1, and COMT polymorphisms, and breast cancer: a case-control study in southwestern China. DNA Cell Biol. 2011 Aug;30(8):585-95. doi: 10.1089/dna.2010.1195[↩]
- Hui C, Qi X, Qianyong Z, Xiaoli P, Jundong Z, Mantian M. Flavonoids, flavonoid subclasses and breast cancer risk: a meta-analysis of epidemiologic studies. PLoS One. 2013;8(1):e54318. doi: 10.1371/journal.pone.0054318[↩]
- Hwang YW, Kim SY, Jee SH, Kim YN, Nam CM. Soy food consumption and risk of prostate cancer: a meta-analysis of observational studies. Nutr Cancer. 2009;61(5):598-606. doi: 10.1080/01635580902825639[↩]
- Miyanaga N, Akaza H, Hinotsu S, Fujioka T, Naito S, Namiki M, Takahashi S, Hirao Y, Horie S, Tsukamoto T, Mori M, Tsuji H. Prostate cancer chemoprevention study: an investigative randomized control study using purified isoflavones in men with rising prostate-specific antigen. Cancer Sci. 2012 Jan;103(1):125-30. doi: 10.1111/j.1349-7006.2011.02120.x[↩]
- Milerová J, Cerovská J, Zamrazil V, Bílek R, Lapcík O, Hampl R. Actual levels of soy phytoestrogens in children correlate with thyroid laboratory parameters. Clin Chem Lab Med. 2006;44(2):171-4. doi: 10.1515/CCLM.2006.031[↩]
- Chandra AK, De N. Goitrogenic/antithyroidal potential of green tea extract in relation to catechin in rats. Food Chem Toxicol. 2010 Aug-Sep;48(8-9):2304-11. doi: 10.1016/j.fct.2010.05.064[↩]
- Egert S, Rimbach G. Which sources of flavonoids: complex diets or dietary supplements? Adv Nutr. 2011 Jan;2(1):8-14. doi: 10.3945/an.110.000026[↩]
- Cermak R. Effect of dietary flavonoids on pathways involved in drug metabolism. Expert Opin Drug Metab Toxicol. 2008 Jan;4(1):17-35. doi: 10.1517/17425255.4.1.17[↩][↩][↩]
- Cermak R, Wolffram S. The potential of flavonoids to influence drug metabolism and pharmacokinetics by local gastrointestinal mechanisms. Curr Drug Metab. 2006 Oct;7(7):729-44. doi: 10.2174/138920006778520570[↩][↩]
- Alvarez AI, Real R, Pérez M, Mendoza G, Prieto JG, Merino G. Modulation of the activity of ABC transporters (P-glycoprotein, MRP2, BCRP) by flavonoids and drug response. J Pharm Sci. 2010 Feb;99(2):598-617. doi: 10.1002/jps.21851[↩][↩]
- Morris ME, Zhang S. Flavonoid-drug interactions: effects of flavonoids on ABC transporters. Life Sci. 2006 Mar 27;78(18):2116-30. doi: 10.1016/j.lfs.2005.12.003[↩][↩]
- Li Y, Paxton JW. The effects of flavonoids on the ABC transporters: consequences for the pharmacokinetics of substrate drugs. Expert Opin Drug Metab Toxicol. 2013 Mar;9(3):267-85. doi: 10.1517/17425255.2013.749858[↩][↩][↩]
- Brand W, Schutte ME, Williamson G, van Zanden JJ, Cnubben NH, Groten JP, van Bladeren PJ, Rietjens IM. Flavonoid-mediated inhibition of intestinal ABC transporters may affect the oral bioavailability of drugs, food-borne toxic compounds and bioactive ingredients. Biomed Pharmacother. 2006 Nov;60(9):508-19. doi: 10.1016/j.biopha.2006.07.081[↩]
- Bailey DG, Dresser GK. Interactions between grapefruit juice and cardiovascular drugs. Am J Cardiovasc Drugs. 2004;4(5):281-97. doi: 10.2165/00129784-200404050-00002[↩][↩]
- Marzolini C, Paus E, Buclin T, Kim RB. Polymorphisms in human MDR1 (P-glycoprotein): recent advances and clinical relevance. Clin Pharmacol Ther. 2004 Jan;75(1):13-33. doi: 10.1016/j.clpt.2003.09.012[↩]
- Freedman JE, Parker C 3rd, Li L, Perlman JA, Frei B, Ivanov V, Deak LR, Iafrati MD, Folts JD. Select flavonoids and whole juice from purple grapes inhibit platelet function and enhance nitric oxide release. Circulation. 2001 Jun 12;103(23):2792-8. doi: 10.1161/01.cir.103.23.2792[↩]
- Keevil JG, Osman HE, Reed JD, Folts JD. Grape juice, but not orange juice or grapefruit juice, inhibits human platelet aggregation. J Nutr. 2000 Jan;130(1):53-6. doi: 10.1093/jn/130.1.53[↩]
- Polagruto JA, Schramm DD, Wang-Polagruto JF, Lee L, Keen CL. Effects of flavonoid-rich beverages on prostacyclin synthesis in humans and human aortic endothelial cells: association with ex vivo platelet function. J Med Food. 2003 Winter;6(4):301-8. doi: 10.1089/109662003772519840[↩]
- Murphy KJ, Chronopoulos AK, Singh I, Francis MA, Moriarty H, Pike MJ, Turner AH, Mann NJ, Sinclair AJ. Dietary flavanols and procyanidin oligomers from cocoa (Theobroma cacao) inhibit platelet function. Am J Clin Nutr. 2003 Jun;77(6):1466-73. doi: 10.1093/ajcn/77.6.1466[↩]
- Bailey DG, Dresser G, Arnold JM. Grapefruit-medication interactions: forbidden fruit or avoidable consequences? CMAJ. 2013 Mar 5;185(4):309-16. doi: 10.1503/cmaj.120951[↩][↩][↩]