Contents
What is methemoglobinemia
Methemoglobinemia (MetHb) is a blood disorder in which an abnormal amount of methemoglobin is produced and the body cannot reuse hemoglobin because it is damaged. Hemoglobin (Hb) is the protein in red blood cells that carries and distributes oxygen to the body. With methemoglobinemia, the hemoglobin can carry oxygen, but is not able to release it effectively to body tissues.
Methemoglobinemia can be either inherited (congenital) or acquired 1. Methemoglobinemia arises when the iron (Fe) in hemoglobin is converted from the ferrous (Fe+2) to the ferric (Fe+3) state 2. Approximately less than 1% of hemoglobin is present in methemoglobin form. The presence of methemoglobin can impart a bluish tinge to the skin color. Methemoglobinemia can be triggered by exposure to the topical anesthetic agent dapsone, nitroglycerin, or other strong oxidizing agents. Inherited methemoglobinemia type 1 and 2 is an autosomal recessive condition that is caused by a mutation in the gene for cytochrome b5 reductase enzyme 2. Inherited methemoglobinemia is extremely rare. The lack of enzymatic activity by cytochrome b5 reductase causes decreased reduction of methemoglobin 2.
Inherited methemoglobinemia is a rare disorder caused by a deficiency of the enzyme methemoglobin reductase, by the synthesis of an abnormal enzyme, or by the presence of an abnormal hemoglobin (hemoglobin M). Although cyanotic from birth, patients who have inherited methemoglobinemia are otherwise well. The fact that the patient was not known to be cyanotic in the past decreases the likelihood of an inherited form of methemoglobinemia in this case. Acquired methemoglobinemia results from exposure to chemicals that oxidize the ferrous iron in hemoglobin to the ferric state at a rate that exceeds the reducing capacity of the methemoglobin reductase enzyme in erythrocytes 3.
What is hemoglobin
Hemoglobin (Hb or Hgb) is the iron-containing protein found in all red blood cells (RBCs) that gives red blood cells their characteristic red color and it carries oxygen (O2) throughout the body. Hemoglobin enables red blood cells to bind to oxygen in the lungs and carry oxygen to tissues and organs throughout your body. Hemoglobin also helps transport a small portion of carbon dioxide (CO2), a product of cell metabolism, from tissues and organs to the lungs, where it is exhaled 4.
Hemoglobin is made up of heme, which is the iron-containing portion, and globin chains, which are proteins. The globin protein consists of chains of amino acids, the “building blocks” of proteins. One portion of hemoglobin called heme is the molecule with iron at the center (see Figure 1). Another portion is made of up four protein chains called globins. Depending on their structure, the globin chains are designated as alpha (α), beta (ß), delta (δ), and gamma (γ). Each of the four globin chains holds a heme group containing one iron atom. .
Not all hemoglobin is the same. Different types of hemoglobin are classified according to the type of globin chains they contain. The type of globin chains present is important in hemoglobin’s ability to transport oxygen.
Normal hemoglobin types include:
- Hemoglobin A (Hb A) – this is the predominant type of hemoglobin (Hb) in adults, makes up about 95%-98% of hemoglobin found in adults; Hb A contains two alpha (α) protein chains and two beta (ß) protein chains.
- Hemoglobin A2 (Hb A2) – makes up about 2-3.5% of hemoglobin (Hb) found in adults; it has two alpha (α) and two delta (δ) protein chains.
- Hemoglobin F (Hb F, fetal hemoglobin) – makes up to 1%-2% of hemoglobin (Hb) found in adults; it has two alpha (α) and two gamma (γ) protein chains. Hemoglobin F (Hb F, fetal hemoglobin) is the primary hemoglobin produced by a developing baby (fetus) during pregnancy. Its production usually falls to a low level within a year after after birth and reaches adult level within 1-2 years.
Under normal circumstances, adult blood contains 4 types of hemoglobin: oxygenated hemoglobin or oxyhemoglobin, hemoglobin that contains no oxygen (reduced hemoglobin) or deoxyhemoglobin, hemoglobin transporting carbon dioxide or carboxyhemoglobin, and oxidized hemoglobin or methemoglobin. The last 2 are usually found in small amounts in blood.
Under normal circumstances, most of the hemoglobin iron exists in the ferrous (Fe+2) state, and a small fraction of the hemoglobin present in erythrocytes undergoes oxidation. As a result of this process, some of the hemoglobin iron is converted to the ferric (Fe+3) state, forming methemoglobin. Oxidized hemoglobin cannot bind or carry oxygen. The physiologic level of methemoglobin is less than or equal to 1% of total hemoglobin concentration, and when greater than 1% of hemoglobin is oxidized to methemoglobin, a hemoglobin deficiency occurs. In normal blood, the methemoglobin reductase system maintains methemoglobin in equilibrium with deoxygenated hemoglobin 5.
Figure 1. Red blood cell hemoglobin
Figure 2. Structure of hemoglobin
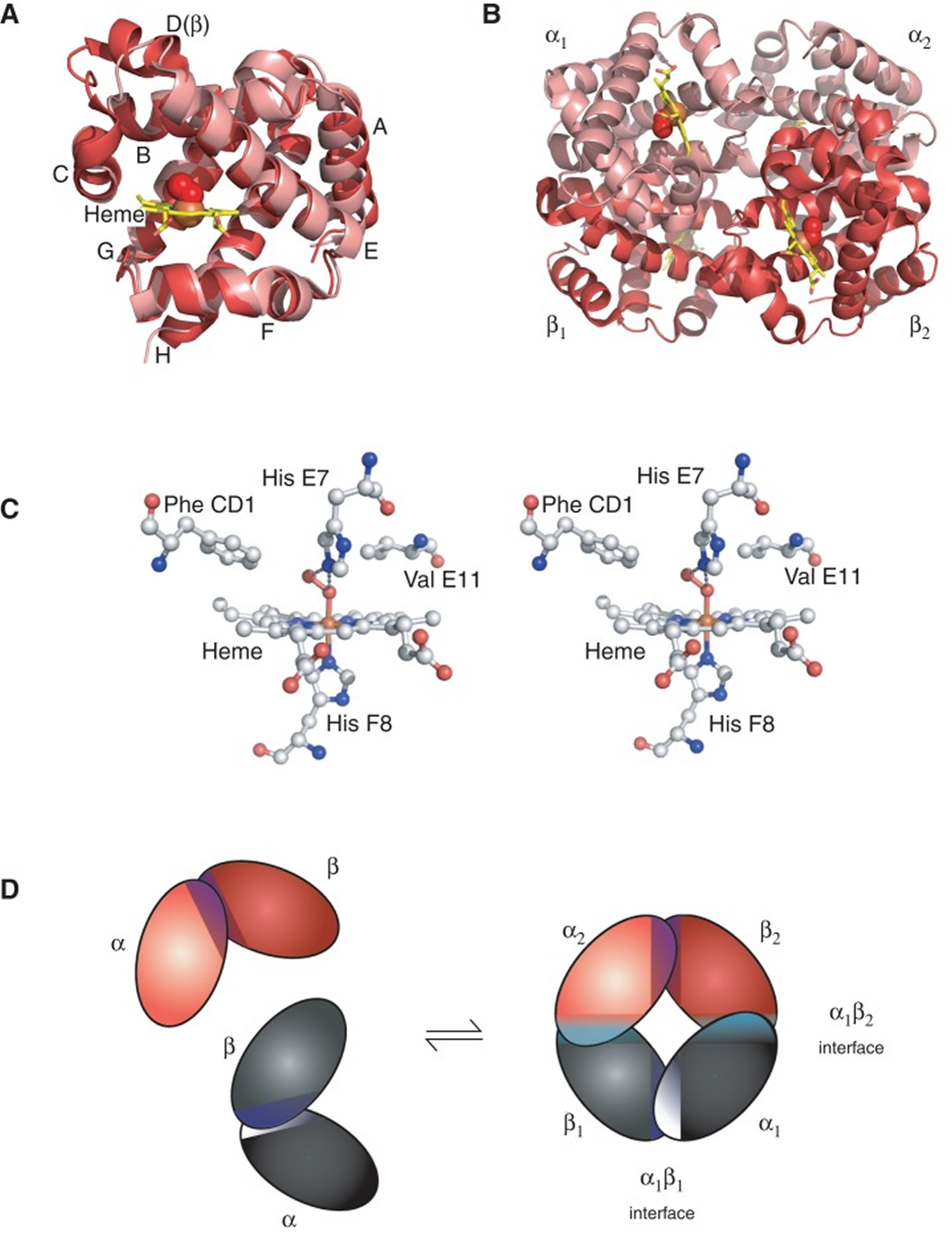
The structure of hemoglobin (Hb). (A) The α (pink) and β (red) hemoglobin (Hb) subunits have conserved α-helical folds. Helices are labeled A–H from the amino terminus. The α subunit lacks helix D. (B) The high O2 affinity R state quaternary structure of hemoglobin (Hb) with O2 (red spheres) bound at all four heme sites (protoporphyrin-IX as yellow sticks, with central iron atom as orange sphere). (C) Stereo (wall-eye) diagram of the heme pocket of β showing the proximal (F8) and distal (E7) histidines and selected residues in the distal heme pocket that influence ligand binding and autoxidation. (D) hemoglobin (Hb) tetramer is assembled from two identical αβ dimers (shown in red and gray for clarity). In the tetramer, each subunit makes contact with the unlike chain through a high affinity dimerization α1β1 interface and a lower affinity α1β2 dimer–tetramer interface (cyan).
What causes methemoglobinemia
Methemoglobinemia can be:
- Passed down through families (inherited or congenital methemoglobinemia)
- Caused by exposure to certain medicines, chemicals, or foods (acquired methemoglobinemia)
There are two forms of inherited methemoglobinemia. The first form is passed on by both parents. The parents usually do not have the condition themselves. They carry the gene that causes the condition. It occurs when there is a problem with an enzyme called cytochrome b5 reductase (reduced nicotinamide adenine dinucleotide (NADH) cytochrome b5 reductase).
There are two types of inherited methemoglobinemia:
- Type 1 (also called erythrocyte cytochrome b5 reductase deficiency) occurs when red blood cells lack the enzyme.
- Type 2 (also called generalized cytochrome b5 reductase deficiency) occurs when the enzyme doesn’t work in the body.
In the type 1 form, the soluble form of the enzyme is deficient only in erythrocytes, and cyanosis is the only symptom 6. Type 2 hereditary methemoglobinemia is due to deficiency of the membrane-bound form of the enzyme, which is located in the outer mitochondrial membrane and the endoplasmic reticulum of somatic cells. Type 2 hereditary methemoglobinemia is a rare disease characterized by deficiency of the enzyme in all tissues and manifesting with severe developmental abnormalities, severe mental retardation, and neurologic impairment, which often lead to premature death 7. Heterozygotes with NADH cytochrome b5 reductase deficiency do not usually manifest signs of methemoglobinemia. However, under the stress of oxidant drugs, severe cyanosis may develop because of methemoglobinemia. Neurologic abnormalities do not respond to methylene blue therapy.
The second form of inherited methemoglobinemia is called hemoglobin M (HbM) disease. It is caused by defects in the hemoglobin protein itself. Hemoglobin M (HbM) disease is inherited in an autosomal dominant pattern. Only one parent needs to pass on the abnormal gene for the child to inherit the disease. In most of the hemoglobin M, tyrosine has been substituted for either the proximal or the distal histidine 8. This results in reduced capacity of the enzymatic machinery of the erythrocyte to efficiently reduce the iron to the divalent form and thus predisposes to methemoglobinemia. These hemoglobin variants are associated with cyanosis, which is present from early life. In the case of the α-chain variants, it is present from birth, whereas the β-chain hemoglobin variants produce cyanosis only after the first few months of life as adult hemoglobin synthesis becomes established.
Acquired methemoglobinemia is more common than the inherited forms. Acquired methemoglobinemia occurs in some people after they are exposed to certain chemicals, toxins and medicines, including:
- Anesthetics such as benzocaine
- Nitrobenzene
- Certain antibiotics (including dapsone and chloroquine)
- Nitrites (used as additives to prevent meat from spoiling)
Drugs or toxins that can cause methemoglobinemia 8:
- Acetanilid
- Alloxan
- Aniline
- Arsine
- Benzene derivatives
- Benzocaine
- Bivalent copper
- Bismuth subnitrate
- Bupivacaine hydrochloride
- Chlorates
- Chloroquine
- Chromates
- Clofazimine
- Dapsone
- Dimethyl sulfoxide
- Dinitrophenol
- Exhaust fumes
- Ferricyanide
- Flutamide
- Hydroxylamine
- Lidocaine hydrochloride
- Metoclopramide hydrochloride
- Methylene blue
- Naphthalene
- Nitrates
- Nitric oxide
- Nitrites
- Nitrofuran
- Nitroglycerin
- Sodium nitroprusside
- Paraquat
- Phenacetin
- Phenazopyridine hydrochloride
- Phenol
- Phenytoin
- Prilocaine hydrochloride
- Primaquine phosphate
- Rifampin
- Silver nitrate
- Sodium valproate
- Smoke inhalation
- Sulfasalazine
- Sulfonamides
- Trinitrotoluene
Footnote: *Certain drugs are more likely to cause methemoglobinemia than others. These are dapsone, local anesthetics, phenacetin, and antimalarial drugs. Screening everybody for methemoglobinemia before exposing them to these drugs is impractical because of the rarity of the condition and because a growing number of drugs are implicated in its causation.
Table 1. Agents known to induce methemoglobinemia
Some of these oxidize hemoglobin directly to form methemoglobin; others do it indirectly by reducing free oxygen to the free radical O2-, which in turn oxidizes hemoglobin to methemoglobin. Outbreaks of methemoglobinemia have occurred due to nitrite poisoning from water contamination 9.
Large amounts of nitric oxide are released in patients with sepsis. Nitric oxide is converted to methemoglobin and nitrate. It has been reported that methemoglobin levels are significantly higher in patients with sepsis than in nonseptic patients 10.
Methemoglobinemia has been reported in young infants (<6 months) in whom severe metabolic acidosis develops from diarrhea and dehydration 11. Young infants may be particularly susceptible to this complication because of their low stomach acid production, large number of nitrite-reducing bacteria, and the relatively easy oxidation of fetal hemoglobin. Small infants have lower erythrocyte levels of cytochrome b reductase 12. Higher intestinal pH of infants may promote the growth of gram-negative organisms that convert dietary nitrates to nitrites.
Methemoglobinemia has been reported in diarrhea induced by hypersensitivity to cow’s milk proteins 13. It has also been reported in association with renal tubular acidosis 14.
Benzocaine-induced methemoglobinemia
A wide variety of agents are known to induce methemoglobinemia (see Table 1), including lidocaine. Benzocaine has been reported to cause methemoglobinemia when applied to infants as an ointment or a rectal suppository 15 and when used topically to the perineal area 16. It has also been associated with methemoglobinemia after its use as a lubricant on endotracheal, bronchoscopic, and esophageal tubes 17. The particular preparation of benzocaine used in this case contained 20% benzocaine (Hurricane) 18.
Methemoglobinemia has also been observed after the use of other local anesthetics. Prilocaine has been implicated most frequently 19. Cyanosis can develop after administration of a dose as low as 500 mg for major nerve block 20. Methemoglobinemia has been also reported after topical use of a eutectic mixture of the local anesthetics lidocaine and prilocaine in children 21. Other commonly used drugs known to cause methemoglobinemia are phenacetin, acetaminophen, vasodilators, sulfonamides, and dapsone.
The onset of methemoglobinemia may be immediate or delayed.
Methemoglobinemia prevention
Genetic counseling is suggested for couples with a family history of methemoglobinemia and are considering having children.
Babies 6 months or younger are more likely to develop methemoglobinemia. Therefore, homemade baby food purees made from vegetables containing high levels of natural nitrates, such as carrots, beetroots, or spinach should be avoided.
Methemoglobinemia symptoms
Symptoms of acquired methemoglobinemia include:
- Bluish coloring of the skin
- Headache
- Giddiness
- Altered mental state
- Fatigue
- Shortness of breath
- Lack of energy
Symptoms of type 1 methemoglobinemia include:
- Bluish coloring of the skin
Symptoms of type 2 methemoglobinemia include:
- Developmental delay
- Failure to thrive
- Intellectual disability
- Seizures
Symptoms of hemoglobin M disease include:
- Bluish coloring of the skin
Methemoglobinemia possible complications
Complications of methemoglobinemia include:
- Shock
- Seizures
- Death
Methemoglobinemia diagnosis
A baby with methemoglobinemia will have a bluish skin color (cyanosis) at birth or shortly afterward. Your health care provider will perform blood tests to diagnose the condition. Tests may include:
- Checking the oxygen level in the blood (pulse oximetry)
- Blood test to check levels of gases in the blood (arterial blood gas analysis)
The arterial blood gas shows the partial pressure of dissolved oxygen in the blood as well as the saturation of hemoglobin.
Blood containing high concentrations of methemoglobin appears chocolate brown. Subjects with methemoglobinemia may have normal partial pressures of oxygen, despite life-threatening methemoglobinemia. The oxygen saturation values, measured by a pulse oximeter, are falsely elevated. The pulse oximeter measures the absorption of light at only two wavelengths which correspond to that of oxyhemoglobin and deoxyhemoglobin. The drawback of measuring only two wavelengths is that it can create a misleading picture when evaluating a patient with cyanosis secondary to methemoglobinemia. This is because abnormal hemoglobin is not picked up by the pulse oximetry; therefore, in methemoglobinemia, the pulse oximetry reading is falsely elevated. Co-oximetry provides a more accurate evaluation of oxygen saturation because it measures the absorption of light at four different wavelengths corresponding to oxyhemoglobin, deoxyhemoglobin, carboxyhemoglobin, and methemoglobin. For a patient presenting with cyanosis in which abnormal hemoglobin is suspected, co-oximetry is a useful diagnostic tool.
Another abnormal hemoglobin that can cause congenital cyanosis is hemoglobin M. This hemoglobin is formed as a result of mild structural changes in the alpha and beta chains that keep it in an oxidized ferric state, thereby reducing the oxygen-carrying capacity. A routine hemoglobin electrophoresis can best detect this.
Cyanosis without cardiorespiratory change is the cardinal sign of methemoglobinemia 1. For definitive diagnosis, spectrophotometry is used. Methemoglobin is not stable in samples of blood and should be measured promptly. Otherwise the measured level may be lower than the values at the time cyanosis was observed.
Cyanosis with a gray-brown hue that is unresponsive to oxygen occurs when the fraction of hemoglobin existing as methemoglobin exceeds 15%. Most patients are asymptomatic until the methemoglobin fraction is greater than 20% to 30%. At this point fatigue, headache, tachycardia, dizziness, and weakness develop. At fractions greater than 45%, dyspnea, bradycardia, hypoxia, metabolic acidosis, seizures, coma, and cardiac arrhythmias may occur. Fractions above 70% are rapidly fatal 5.
In methemoglobinemia due to drug exposure, an elevated level of methemoglobin is found, but the activity of NADH cytochrome b5 reductase is normal. In hereditary type 2 methemoglobinemia, the enzyme’s activity is less than 20% of normal. Hemoglobin M may be differentiated from methemoglobin formed from hemoglobin A by its absorption spectrum in the range of 450 to 750 nm. Electrophoresis at pH 7.1 is most useful for the separation of hemoglobin M 22.
Methemoglobinemia treatment
People with hemoglobin M disease don’t have symptoms. So, they may not need treatment.
The course of hereditary methemoglobinemia type 1 is benign, but these patients should not be administered oxidant drugs 8. Treatment may be required for cosmetic reasons or for an inadvertent use of oxidant drugs.
For methemoglobinemia due to drug exposure, traditional first-line therapy consists of an infusion of methylene blue, whose action depends on the availability of reduced nicotinamide adenine nucleotide phosphate (NADPH) within the red blood cells. Intravenous administration of methylene blue (1–2 mg/kg) as a 1% solution over 5 minutes quickly relieves cyanosis due to methemoglobinemia. Intravenous methylene blue is indicated for methemoglobin fractions over 30% and at lower fractions in patients with anemia or cardiovascular disease. The dose may be repeated if no clinical response is observed within 1 hour. A dose greater than 7 mg/kg of methylene blue by itself can cause methemoglobinemia 23. Supplemental oxygen should also be administered. After an acute exposure to an oxidizing agent, treatment should be considered when the methemoglobin is 30% in an asymptomatic patient and 20% in a symptomatic patient 24. Patients with anemia or cardiorespiratory problems should be treated at lower levels of methemoglobin. Methemoglobinemia due to hemoglobin M does not respond to ascorbic acid or methylene blue.
Nicotinamide adenine dinucleotide phosphate (NADPH) helps in the conversion of methylene blue to leucomethylene blue. This end product helps reduce methemoglobin to normal hemoglobin 2. Dextrose should be given 25 because the major source of NADH in the red blood cells is the catabolism of sugar through glycolysis. Dextrose is also necessary to form NADPH through the hexose monophosphate shunt, which is necessary for methylene blue to be effective.
Methylene blue is an oxidant; its metabolic product leukomethylene blue is the reducing agent. Therefore, large doses of methylene blue may result in higher levels of methylene blue rather than the leukomethylene blue, which will result in hemolysis and, paradoxically, methemoglobinemia in patients with glucose-6-phosphate dehydrogenase (G6PD) deficiency 26. Patients with G6PD deficiency also may not produce sufficient NADPH to reduce methylene blue to leukomethylene blue; thus, methylene blue therapy may be ineffective in these patients 26.
Methylene blue may be unsafe in people who have or may be at risk for glucose-6-phosphate dehydrogenase (G6PD) deficiency. They should not take this medicine. If you or your child has G6PD deficiency, always tell your provider before getting treatment.
Some drugs, such as dapsone, benzocaine, and aniline, produce a rebound methemoglobinemia, in which methemoglobin levels increase 4 to 12 hours after successful methylene blue therapy 27.
N-Acetylcysteine, cimetidine, and ketoconazole are experimental therapies in the treatment of methemoglobinemia that have shown some promising results 28. Exchange transfusion is reserved for patients in whom methylene blue therapy is ineffective.
Ascorbic acid may also be used to reduce the level of methemoglobin, but its action is slower than that of methylene blue. Ascorbic acid, 300 to 600 mg orally daily divided into 3 or 4 doses, is helpful 29.
Alternative treatments include hyperbaric oxygen therapy, red blood cell transfusion and exchange transfusions.
In most cases of mild acquired methemoglobinemia, no treatment is needed. But you should avoid the medicine or chemical that caused the problem. Severe cases may need a blood transfusion.
Methemoglobinemia prognosis
People with inherited methemoglobinemia type 1 and hemoglobin M disease often do well. Inherited methemoglobinemia type 2 is more serious. It often causes death within the first few years of life.
People with acquired methemoglobinemia often do very well once the medicine, food, or chemical that caused the problem is identified and avoided.
- Hegedus F, Herb K. Benzocaine-induced methemoglobinemia. Anesth Prog. 2005;52(4):136-9. https://www.ncbi.nlm.nih.gov/pmc/articles/PMC1586795/[↩][↩][↩]
- Adeyinka A, Kondamudi NP. Cyanosis. [Updated 2018 Oct 27]. In: StatPearls [Internet]. Treasure Island (FL): StatPearls Publishing; 2018 Jan-. Available from: https://www.ncbi.nlm.nih.gov/books/NBK482247[↩][↩][↩][↩]
- Mansouri A, Lurie AA. Concise review: methemoglobinemia. Am J Hematol. 1993;42:7–12.[↩]
- Thom CS, Dickson CF, Gell DA, Weiss MJ. Hemoglobin Variants: Biochemical Properties and Clinical Correlates. Cold Spring Harbor Perspectives in Medicine. 2013;3(3):a011858. doi:10.1101/cshperspect.a011858. https://www.ncbi.nlm.nih.gov/pmc/articles/PMC3579210/[↩]
- Wright RO, Lewander WJ, Woolf AD. Methemoglobinemia: etiology, pharmacology, and clinical management. Ann Emerg Med. 1999;34:646–656.[↩][↩]
- Gibson QH. The reduction of methemoglobin in red blood cells and studies on the cause of idiopathic methemoglobinemia. Biochem J 1948;42: 13-23.[↩]
- Worster-Drought C, White JC, Sargent F. Familial, idiopathic methaemoglobinaemia associated with mental deficiency and neurological abnormalities. Br Med J 1953;2: 114-118.[↩]
- Rehman HU. Methemoglobinemia. West J Med. 2001;175(3):193-6. https://www.ncbi.nlm.nih.gov/pmc/articles/PMC1071541/[↩][↩][↩]
- Askew GL, Finelli L, Genese CA, Sorhage FE, Sosin DM, Spitalny KC. Boilerbaisse: an outbreak of methemoglobinemia in New Jersey in 1992. Pediatrics 1994;94: 381-384.[↩]
- Ohashi K, Yukioka H, Hayashi M, Asada A. Elevated methemoglobin in patients with sepsis. Acta Anaesthesiol Scand 1998;42: 713-716.[↩]
- Pollack ES, Pollack CV. Incidence of subclinical methemoglobinemia in infants with diarrhea. Ann Emerg Med 1994;24: 652-656.[↩]
- Hjelt K, Lund JT, Scherling B, et al. Methaemoglobinaemia among neonates in a neonatal intensive care unit. Acta Paediatr 1995;84: 365-370.[↩]
- Catalan Munoz M, Carrasco Sanchez P, Gentles MG, et al. Methemoglobinemia, acidemia and diarrhea induced by hypersensitivity to cow’s milk proteins [in Spanish]. An Esp Pediatr 1996;44: 295-296.[↩]
- Sager S, Grayson GH, Feig SA. Methemoglobinemia associated with acidosis of probable renal origin. J Pediatr 1995;126: 59-61.[↩]
- Wright RO, Lewander WJ, Woolf AD. Methemoglobinemia: etiology, pharmacology, and clinical management. Ann Emerg Med. 1999;34:646–656[↩]
- Ferraro-Borgida MJ, Mulhern SA, DeMeo MO, Bayer MJ. Methemoglobinemia from perineal application of an anesthetic cream. Ann Emerg Med. 1996;27:785–788.[↩]
- Novaro GM, Aronow HD, Militello MA, Garcia MJ, Sabik EM. Benzocaine-induced methemoglobinemia: experience from a high-volume transesophageal echocardiography laboratory. J Am Soc Echocardiogr. 2003;16:170–175.[↩]
- Spielman FJ, Anderson JA, Terry WC. Benzocaine-induced methemoglobinemia during general anesthesia. J Oral Maxillofac Surg. 1984;42:740–743.[↩]
- Klos CP, Hays GL. Prilocaine-induced methemoglobinemia in a child with Shwachman syndrome. J Oral Maxillofac Surg. 1985;43:621–623[↩]
- Warren RE, Van de Mark TB, Weinberg S. Methemoglobinemia induced by high doses of prilocaine. Oral Surg. 1974;37:866.[↩]
- Sinisterra S, Miravet E, Alfonso I, Soliz A, Papazian O. Methemoglobinemia in an infant receiving nitric oxide after use of eutectic mixture of local anesthetic. J Pediatr. 2002;141:285–286.[↩]
- Beutler E. Methemoglobinemia and other causes of cyanosis. In: Beutler E, Lichtman MA, Coller BS, Kipps TJ, eds. Williams Hematology. 5th ed. New York: McGraw-Hill; 1994: 654-662.[↩]
- Methemoglobinemia and hemolysis after enteral administration of methylene blue in a preterm infant: relevance for pediatric surgeons. Allegaert K, Miserez M, Lerut T, Naulaers G, Vanhole C, Devlieger H. J Pediatr Surg. 2004 Jan; 39(1):E35-7.[↩]
- Price D. Methemoglobinemia. In: Goldfrank LR, Flomenbaum NE, Lewin NA, Weisman RS, Howland MA, Hoffman RS, eds. Goldfrank’s Toxicologic Emergencies. 6th ed. Old Tappan, NJ: Appleton & Lange; 1998: 1507-1523.[↩]
- Regulatory factors in methylene blue catalysis in erythrocytes. Roigas H, Zoellner E, Jacobasch G, Schultze M, Rapoport S. Eur J Biochem. 1970 Jan; 12(1):24-30.[↩]
- Studies of the efficacy and potential hazards of methylene blue therapy in aniline-induced methaemoglobinaemia. Harvey JW, Keitt AS. Br J Haematol. 1983 May; 54(1):29-41.[↩][↩]
- Glucose-6-phosphate dehydrogenase deficiency. Beutler E. N Engl J Med. 1991 Jan 17; 324(3):169-74.[↩]
- Wright RO, Magnani B, Shannon MW, Woolf AD. N-Acetylcysteine reduces methemoglobin in vitro. Ann Emerg Med 1996;28: 499-503.[↩]
- Bolyai JZ, Smith RP, Gray CT. Ascorbic acid and chemically induced methemoglobinemias. Toxical Appl Pharmacol 1972;21: 176-185.[↩]