Contents
- What are antioxidants
- Can antioxidant supplements help prevent cancer?
- Should people with cancer take antioxidant supplements?
- Can antioxidant supplements prevent age-related macular degeneration (AMD)?
- Can antioxidant supplements prevent heart disease?
- Can antioxidant supplements prevent lung disease?
- Can antioxidant supplements prevent cognitive decline?
- Can antioxidant supplements prevent early death?
- Antioxidant Foods
- Antioxidant Supplements
- Are antioxidant supplements safe?
What are antioxidants
Antioxidants also known as “free radical scavengers” are natural or man-made chemicals that interact with and neutralize “free radicals”, preventing them from causing damage or delay some types of cell damage. “Free radicals” are highly reactive chemicals with voracious appetite for electrons (small negatively charged particles found in atoms), stealing them from any nearby substances that will yield them that have the potential to harm cells. This electron theft can radically alter the “loser’s” structure or function. “Free radicals” are created when an atom or a molecule (a chemical that has two or more atoms) either gains or loses an electron (a small negatively charged particle found in atoms). “Free radicals” come in many shapes, sizes, and chemical configurations. “Free radicals” damage can change the instructions coded in a strand of DNA. “Free radicals” that contain the element oxygen also called “reactive oxygen species” or “ROS” are the most common type of free radicals produced in living tissue 1, 2. Reactive oxygen species (ROS) are highly reactive chemicals containing oxygen that react easily with other molecules, resulting in potentially damaging modifications. An excessive chronic amount of free radicals in the body causes a condition called “oxidative stress”. Oxidative stress is a condition in which the effects of free radicals, reactive oxygen and reactive nitrogen species exceed the ability of antioxidant systems to neutralize them, which may damage cells and lead to chronic diseases like cancer, diabetes, autoimmune diseases, atherosclerosis, and heart disease and the aging process 3, 4, 5, 6. Free radicals are formed naturally in your body as byproducts of turning food into energy and play an important role in many normal cellular processes 1, 2. For examples, reactive oxygen species (ROS) can function as normal second messengers modulating many signal transduction pathways important in cell growth 7 and also play an essential role in killing many pathogens during the process of macrophage phagocytosis 8. Free radicals are also formed after exercising or exposure to cigarette smoke, alcohol, air pollution, environmental toxins, some metals, high-oxygen atmospheres, sunlight, ultraviolet rays from the sun or tanning beds and and substances found in processed foods. When ionizing radiation hits an atom or a molecule in a cell, an electron may be lost, leading to the formation of a free radical. The production of abnormally high levels of free radicals is the mechanism by which ionizing radiation kills cells. At high concentrations, free radicals can be hazardous to your body and damage all major components of cells, including DNA, proteins, and cell membranes. The damage to cells caused by free radicals, especially the damage to DNA, may play a role in the development of cancer and other health conditions 3, 1, 2. For example, free radicals can make a circulating low-density lipoprotein (LDL) sometimes called “bad cholesterol” more likely to get trapped in an artery wall. Or free radicals can alter a cell’s membrane, changing the flow of what enters the cell and what leaves it.
Cigarette smoking is a major source of “oxidative stress” and is a major risk factor for atherosclerosis and cancer, particularly lung cancer 9. Women who smoke during pregnancy correlates with many neonatal health problems 10. Oxygen therapy for premature and/or very low birth weight infants contributes to retinopathy of prematurity 11. Premature infants are at increased risk for oxygen toxicity. The retinal microvasculature is initially inhibited then accelerated once supplemental oxygen is removed 11. Reactive oxygen species (ROS) production is a risk factor for age-related macular degeneration (AMD). The most common type of AMD is dry AMD and treatment consists of close observation with supplementation of antioxidants (AREDS or AREDS2 trials) 12, 13, 14, 15, 16, 17. Some of the bacteria that colonize the gastrointestinal tract, primarily the large intestine, can produce an abundance of reactive oxygen species (ROS) possibly contributing to colorectal cancer and inflammatory bowel disease (IBD) 18, 19. Reactive oxygen species (ROS) also has a role in neurological disease. Point mutations in copper-zinc-SOD1 are associated with the familial form of amyotrophic lateral sclerosis (ALS) 20. There are things you can do to help fight “free radicals” and reduce the damage they cause in your body. You can stop smoking, be sun smart, and eat healthy. Antioxidants may also help.
There is extensive evidence that people who eat more vegetables and fruits, foods that are rich sources of antioxidants have lower risks of chronic diseases. For example:
- A 2017 review of 95 observational studies, with more than 2 million total participants, showed that people who had higher intakes of fruits and vegetables had lower risks of cardiovascular disease and cancer 21.
- A 2023 study from the United Kingdom in which 72,160 people were followed for an average of 9 years showed that higher intakes of fruits and vegetables were associated with a lower risk of cataracts 22, 23.
Antioxidants include:
- Carotenoids. Beta-carotene, lycopene and lutein are well-known carotenoids in the fight to reduce the damage from free radicals. Foods high in carotenoids may be effective in helping to reduce the chance of developing certain cancers and may help decrease your risk of macular degeneration. Foods high in carotenoids include red, orange, deep-yellow and some dark-green leafy vegetables; these include sweet potatoes, spinach, carrots, tomatoes, Brussels sprouts, winter squash and broccoli.
- Lutein. Lutein is a type of carotenoids 24. Lutein is related to beta-carotene and vitamin A. Many people think of lutein as “the eye vitamin”. Lutein and zeaxanthin are the two major carotenoids found in the human eye (macula and retina). Dietary lutein and zeaxanthin are selectively taken up into the macula of the eye, where they absorb up to 90% of blue light protecting the eye tissues from sunlight damage and help maintain optimal visual function. Foods rich in lutein include egg yolks, spinach, kale, corn, orange pepper, kiwi fruit, grapes, zucchini, and squash. Lutein is commonly taken by mouth to prevent eye diseases, including cataracts and a macular disease that leads to vision loss in older adults called age-related macular degeneration or AMD. Evidence is lacking to suggest a role for lutein and zeaxanthin in the management of other eye conditions, including cataracts, diabetic retinopathy, and retinopathy of maturity.
- Lycopene. Lycopene is a type carotenoids 24. Lycopene is related to beta-carotene and gives some vegetables and fruits (e.g., tomatoes) a red color. Lycopene is a powerful antioxidant that might help protect cells from damage. Lycopene is found in tomato, watermelon, red orange, pink grapefruit, apricot, rose hip, and guava. Lycopene is used for high blood pressure, high cholesterol, cancer, and many other conditions, but there is no good scientific evidence to support most of these uses. To date, most small-scale intervention studies have found little-to-no benefit of lycopene supplements in reducing the incidence or severity of prostate cancer in high-risk patients.
- Selenium. Selenium is often thought to be a dietary antioxidant, but the antioxidant effects of selenium are most likely due to the antioxidant activity of proteins that have selenium as an essential component (i.e., selenoproteins or selenium-containing proteins), and not to selenium itself 25.
- Vitamin A. Vitamin A is a generic term that refers to fat-soluble compounds found as preformed vitamin A (retinol and retinyl esters) in animal products and as provitamin A carotenoids in fruit and vegetables 26, 27, 28. Provitamin A carotenoids are plant pigments that include beta-carotene, alpha-carotene, and beta-cryptoxanthin that can be converted by the body into retinol 29, 30. The three active forms of vitamin A in the body are retinol, retinal, and retinoic acid 27, 28. The body converts provitamin A carotenoids into vitamin A in the intestine via the beta-carotene monooxygenase type 1 BCMO1 enzyme 31, 29, although conversion rates may have genetic variability 32, 33, 34. Other carotenoids in food, such as lycopene, lutein, and zeaxanthin, are not converted into vitamin A and are referred to as non-provitamin A carotenoids; they might have other important activities not involving vitamin A formation 29. Vitamin A is involved in immune function, cellular communication, growth and development, and male and female reproduction 29, 35, 36. Vitamin A supports cell growth and differentiation, playing a critical role in the normal formation and maintenance of the heart, lungs, eyes, and other organs 29, 35. Vitamin A is also critical for vision as an essential component of rhodopsin, the light-sensitive protein in the retina that responds to light entering the eye, and because it supports the normal differentiation and functioning of the conjunctival membranes and cornea 35, 26.
- Vitamin C. Vitamin C also known as L-ascorbic acid, is a water-soluble vitamin 37, 38. Unlike most mammals and other animals, humans do not have the ability to synthesize vitamin C and must obtain it from the diet 37, 38. Vitamin C is an essential cofactor in numerous enzymatic reactions, e.g., in the biosynthesis of collagen, carnitine, and neurotransmitters, and vitamin C is also involved in protein metabolism and the regulation of gene expression 39, 40. Vitamin C is also an important physiological antioxidant 41 and has been shown to regenerate other antioxidants within the body, including alpha-tocopherol (vitamin E) 42. Ongoing research is examining whether vitamin C, by limiting the damaging effects of free radicals through its antioxidant activity, might help prevent or delay the development of certain cancers, cardiovascular disease, and other diseases in which oxidative stress plays a causal role. Vitamin C offers a wide variety of health benefits. These benefits include protecting your body from infection and damage to body cells, helping produce collagen (the connective tissue that holds bones and muscles together) and helping in the absorption of iron. To take advantage of these benefits, eat foods rich in vitamin C such as citrus fruits (including oranges, grapefruits and tangerines), strawberries, sweet peppers, tomatoes, broccoli and potatoes.
- Vitamin E. Vitamin E is the collective name for a group of fat-soluble compounds (alpha-, beta-, gamma-, and delta-tocopherol and alpha-, beta-, gamma-, and delta-tocotrienol) with distinctive antioxidant activities 43. Alpha-tocopherol (α-tocopherol) is the only form that is recognized to meet human requirements 44. Research has demonstrated the broad role of vitamin E in promoting health. The main role of vitamin E is as an antioxidant. Research has looked at its possible role in helping to protect your body from cell damage that can lead to cancer, heart disease and cataracts as we age. Vitamin E also may work with other antioxidants such as vitamin C to offer protection from some chronic diseases. Vitamin E is found in vegetable oils, wheat germ, whole-grains and fortified cereals, seeds, nuts and peanut butter.
Your body makes some of the antioxidants you need. Additional antioxidants come from foods, such as fruits, vegetables, and grains. Vegetables and fruits are rich sources of antioxidants. There is good evidence that eating a diet with lots of vegetables and fruits is healthy and lowers risks of certain diseases 45, 22. But it isn’t clear whether this is because of the antioxidants, something else in the foods, or other factors.
Antioxidants are also available as dietary supplements. However, high-dose antioxidant supplements may be linked to health risks in some cases. For example, high doses of beta-carotene may increase the risk of lung cancer in smokers. High doses of vitamin E may increase risks of prostate cancer and one type of stroke 46, 47. The The Selenium and Vitamin E Cancer Prevention Trial (SELECT) evaluated the potential effect of “vitamin E” and/or selenium supplementation on prostate cancer 46, 47. The SELECT study found that neither vitamin E nor selenium supplementation decreased the incidence of prostate cancer or any other cancer followed in the trial 46, 47. Moreover, a subsequent follow-up to the initial Selenium and Vitamin E Cancer Prevention Trial (SELECT) trial found a significantly increased risk of developing prostate cancer in men taking vitamin E. The SELECT trial showed that not all antioxidants are good for human health. Whether or not other forms of vitamin E, such as tocotrienols, have an anticancer effect has yet to be determined. While antioxidants are thought to increase degradation of reactive oxygen species (ROS), an Iowa Women’s Health Study found an increased risk of death in elderly women who used supplements with antioxidant activity 48. Therefore, the clinical role of dietary antioxidants or antioxidant supplements in preventing the diseases associated with oxidative stress remains uncertain.
Antioxidant supplements may also interact with some medicines. To minimize the drug interacttion, tell your doctor about any antioxidants you use.
In summary, except for age-related macular degeneration (AMD), there is currently no evidence that antioxidant supplements have a positive impact on diseases.
There are several possible reasons why antioxidant supplements may not prevent cardiovascular disease and cancer even though antioxidant-rich vegetables and fruits are associated with reduced risk:
- The beneficial health effects of a diet high in vegetables and fruits or other antioxidant-rich foods may be due to other substances in these foods or other aspects of people’s lifestyles that are associated with eating these foods. For example, in a study of adults living in rural areas in the United States, eating at least five servings of fruits and vegetables daily was associated with several other factors that might affect health, such as getting at least moderate physical activity and having had a routine medical exam in the past year.
- Antioxidants consumed as purified chemicals might act differently than those consumed in foods, which contain complex mixtures of substances.
- The high doses of antioxidants in dietary supplements may have different effects than the smaller amounts in foods.
If you are considering a dietary supplement, first get information on it from reliable sources. Keep in mind that dietary supplements may interact with medications or other supplements and may contain ingredients not listed on the label. Your doctor and pharmacist can advise you. If you are pregnant or breastfeeding a child, or if you are considering giving a child a dietary supplement, it is especially important to consult your (or your child’s) doctor.
Can antioxidant supplements help prevent cancer?
In laboratory and animal studies, the presence of increased levels of exogenous antioxidants has been shown to prevent the types of free radical damage that have been associated with cancer development. Therefore, scientists have investigated whether taking dietary antioxidant supplements can help lower the risk of developing or dying from cancer in humans. Many observational studies, including case–control studies and cohort studies, have been conducted to investigate whether the use of dietary antioxidant supplements is associated with reduced risks of cancer in humans. Overall, these studies have yielded mixed results 49. To date, nine randomized controlled trials of dietary antioxidant supplements for cancer prevention have been conducted worldwide 50. The results of these nine trials are summarized in Table 1 below. The current evidence does not support antioxidant supplements can prevent cancer. In fact, high doses of one antioxidant such as beta-carotene may increase the risk of lung cancer.
These nine randomized controlled clinical trials did not provide evidence that dietary antioxidant supplements are beneficial in primary cancer prevention 50. In addition, in 2022 a systematic review of the available evidence regarding the use of vitamin and mineral supplements for the prevention of chronic diseases, including cancer, conducted for the United States Preventive Services Task Force (an independent panel of experts that makes evidence-based recommendations about disease prevention) likewise found no clear evidence of benefit in preventing cancer and recommended against the use of beta-carotene or vitamin E supplements for cancer prevention 51, 52. The United States Preventive Services Task Force also concluded that the evidence is insufficient to make recommendations about supplements of other single nutrients or pairs of nutrients. These recommendations are consistent with the Task Force’s previous recommendations, issued in 2014.
It is possible that the lack of benefit in clinical studies can be explained by differences in the effects of the tested antioxidants when they are consumed as purified chemicals as opposed to when they are consumed in foods, which contain complex mixtures of antioxidants, vitamins, and minerals 53. Therefore, acquiring a more complete understanding of the antioxidant content of individual foods, how the various antioxidants and other substances in foods interact with one another, and factors that influence the uptake and distribution of food-derived antioxidants in the body are active areas of ongoing cancer prevention research.
Table 1. Antioxidant supplements for cancer prevention trials
Trial name, country (reference) | Intervention | Study subjects | Results |
---|---|---|---|
Linxian General Population Nutrition Intervention Trial, China 54, 55 | 15 milligrams (mg) beta-carotene, 30 mg alpha-tocopherol, and 50 micrograms (µg) selenium daily for 5 years | Healthy men and women at increased risk of developing esophageal cancer and gastric cancer | Initial: no effect on risk of developing either cancer; decreased risk of dying from gastric cancer only Later: no effect on risk of dying from gastric cancer Later: no effect on risk of dying from gastric cancer |
Alpha-Tocopherol/Beta-Carotene Cancer Prevention Study (ATBC), Finland 56, 57, 58, 59, 60 | Alpha-tocopherol (50 mg per day) and/or beta-carotene (20 mg per day) supplements for 5 to 8 years | Middle-aged male smokers | Initial: increased incidence of lung cancer for those who took beta-carotene supplements Later: no effect of either supplement on incidence of urothelial, pancreatic, colorectal, renal cell, or upper aerodigestive tract cancers |
Carotene and Retinol Efficacy Trial (CARET), United States 61, 62, 63 | Daily supplementation with 15 mg beta-carotene and 25,000 International Units (IU) retinol | People at high risk of lung cancer because of a history of smoking or exposure to asbestos | Initial: increased risk of lung cancer and increased death from all causes—trial ended early Later: higher risks of lung cancer and all-cause mortality persisted; no effect on risk of prostate cancer |
Physicians’ Health Study I (PHS I), United States 64 | Beta-carotene supplementation (50 mg every other day for 12 years) | Male physicians | No effect on cancer incidence, cancer mortality, or all-cause mortality in either smokers or non-smokers |
Women’s Health Study (WHS), United States 65, 66 | Beta-carotene supplementation (50 mg every other day), vitamin E supplementation (600 IU every other day), and aspirin (100 mg every other day) | Women ages 45 and older | Initial: no benefit or harm associated with 2 years of beta-carotene supplementation Later: no benefit or harm associated with 2 years of vitamin E supplementation |
Supplémentation en Vitamines et Minéraux Antioxydants (SU.VI.MAX) Study, France 67, 68, 69, 70 | Daily supplementation with vitamin C (120 mg), vitamin E (30 mg), beta-carotene (6 mg), and the minerals selenium (100 µg) and zinc (20 mg) for a median of 7.5 years | Men and women | Initial: lower total cancer and prostate cancer incidence and all-cause mortality among men only; increased incidence of skin cancer among women only Later: no evidence of protective effects in men or harmful effects in women within 5 years of ending supplementation |
Heart Outcomes Prevention Evaluation–The Ongoing Outcomes (HOPE–TOO) Study, International 71 | Daily supplementation with alpha-tocopherol (400 IU) for a median of 7 years | People diagnosed with cardiovascular disease or diabetes | No effect on cancer incidence, death from cancer, or the incidence of major cardiovascular events |
Selenium and Vitamin E Cancer Prevention Trial (SELECT), United States 46, 47 | Daily supplementation with selenium (200 µg), vitamin E (400 IU), or both | Men ages 50 and older | Initial: no reduction in incidence of prostate or other cancers—trial stopped early Later: more prostate cancer cases among those who took vitamin E alone |
Physicians’ Health Study II (PHS II), United States 72 | 400 IU vitamin E every other day, 500 mg vitamin C every day, or a combination of the two | Male physicians ages 50 years and older | No reduction in incidence of prostate cancer or other cancers |
Should people with cancer take antioxidant supplements?
Several randomized controlled trials, some including only small numbers of patients, have investigated whether taking antioxidant supplements during cancer treatment alters the effectiveness or reduces the toxicity of specific therapies 73. Although these trials had mixed results, some found that people who took antioxidant supplements during cancer therapy had worse outcomes, especially if they were smokers 73.
In some preclinical studies, antioxidants have been found to promote tumor growth and spread (metastasis) in tumor-bearing mice and to increase the ability of circulating tumor cells to metastasize 74, 75, 76. Until more is known about the effects of antioxidant supplements in cancer patients, these supplements should be used with caution 50. Cancer patients should inform their doctors about their use of any dietary supplement 50.
Antioxidant supplements haven’t been shown to prevent age-related macular degeneration (AMD) in people who don’t already have this disease, but certain supplements containing a combination of antioxidants and zinc have been shown to slow the progression of age-related macular degeneration (AMD).
- A 2023 Cochrane review of 19 randomized controlled trials compared antioxidant vitamin/mineral supplements (multivitamin, vitamin E, lutein, zeaxanthin, zinc) with placebo or no intervention in people with age-related macular degeneration (AMD) 77. The participants were generally well-nourished. The study found that people taking the vitamins were less likely to progress to late-stage AMD and vision loss 77. However, the study authors noted that taking lutein and zeaxanthin alone or vitamin E alone did not have a beneficial effect on these eye conditions 77.
- For people who already have age-related macular degeneration (AMD), supplements containing a combination of antioxidants and zinc may slow the progression of the disease. The evidence for this comes from two large studies sponsored by the Age-Related Eye Disease Study (AREDS) and Age-Related Eye Disease Study 2 (AREDS2).
- AREDS evaluated the effects of a dietary supplement containing high doses of vitamins C and E, beta-carotene, zinc, and copper on the progression of AMD 78, 79. Almost 4,800 people participated in this study, including 3,640 who had AMD. Among people with intermediate AMD, the supplement reduced the risk of developing advanced AMD by about 25 percent.
- A follow-up trial to the AREDS, the AREDS2, examined lutein and zeaxanthin supplementation on late age-related macular degeneration (AMD) in about 4,200 men and women for up to five years 80 . Participants were randomly assigned to groups that received the original formula or various modified versions. The modifications included removing beta-carotene and adding lutein and zeaxanthin (two carotenoids that are found in the eye). Because the link between beta-carotene and an increased risk of lung cancer in smokers was known before this study started, current smokers were not assigned to groups that received beta-carotene; only nonsmokers and former smokers were included in those groups. After 10 years of follow-up, lutein and zeaxanthin proved to be more effective than beta-carotene in reducing the risk of progression to advanced AMD. Beta-carotene increased lung cancer risk among former smokers, but lutein and zeaxanthin did not.
- The Selenium and Vitamin E Cancer Prevention Trial (SELECT) Eye Endpoints Study 81, which followed 11,267 men for a mean of five years, did not find that vitamin E and selenium supplements, in combination or alone, protected from age-related cataracts.
Can antioxidant supplements prevent heart disease?
Vitamin E, beta-carotene, and other antioxidants supplements aren’t the silver bullet against heart disease and stroke that researchers were hoping for 82. A modest effect of vitamin E has been found in some studies but more research is needed. In the Women’s Health Study, 39,876 initially healthy women took 600 IU of natural source vitamin E or a placebo every other day for 10 years 83. At the study’s end, the rates of major cardiovascular events and cancer were no lower among those taking vitamin E than those taking the placebo 83. These data do not support recommending vitamin E supplementation for cardiovascular disease or cancer prevention among healthy women 83.
Earlier large vitamin E trials conducted among individuals with previously diagnosed coronary disease or at high risk for it, generally showed no benefit. In the Heart Outcomes Prevention Evaluation (HOPE) trial, the rates of major cardiovascular events were essentially the same in the vitamin E (21.5%) and placebo (20.6%) groups, although participants taking vitamin E had higher risks of heart failure and hospitalization for heart failure 71. In the Gruppo Italiano per lo Studio della Sopravvivenza nell’Infarto Miocardico (GISSI) trial, the results were mixed but mostly showed no preventive effects after more than three years of treatment with vitamin E among 11,000 heart attack survivors 84. However, some studies suggest potential benefits among certain subgroups. A recent trial of vitamin E in Israel, for example, showed a marked reduction in coronary heart disease among people with type 2 diabetes who have a common genetic predisposition for greater oxidative stress 85. Beta-carotene, meanwhile, did not provide any protection against heart disease or stroke, as demonstrated by the Physicians’ Health Study 64. In the Supplementation en Vitamines et Mineraux Antioxydants (SU.VI.MAX) study 67, 13,017 French men and women took a single daily capsule that contained 120 mg vitamin C, 30 mg vitamin E, 6 mg beta-carotene, 100 mcg selenium, and 20 mg zinc, or a placebo, for seven and a half years. The vitamins had no effect on overall rates of cardiovascular disease 67. In the Women’s Antioxidant Cardiovascular Study 86, vitamin E, vitamin C, and beta-carotene had similar effects as a placebo on heart attack, stroke, coronary revascularization, and cardiovascular death, although there was a modest and significant benefit for vitamin E among women with existing cardiovascular disease.
Can antioxidant supplements prevent lung disease?
A 2014 CARDIA study from the Journal of Respiratory Research 87 found that different isoforms of vitamin E called tocopherols had opposing effects on lung function. The study analyzed data from the Coronary Artery Risk Development in Young Adults (CARDIA) cohort and measured serum levels of alpha- and gamma-tocopherol in 4,526 adults 87. Lung function was tested using spirometric parameters: higher parameters are indicative of increased lung function, while lower parameters are indicative of decreased lung function. The study found that higher serum levels of alpha-tocopherol (α-tocopherol) were associated with higher spirometric parameters and that high serum levels of gamma-tocopherol (γ-tocopherol) were associated with lower spirometric parameters 87. Though the study was observational in nature, it confirmed the mechanistic pathway of alpha- and gamma-tocopherol in mice studies 88.
Can antioxidant supplements prevent cognitive decline?
The Physicians’ Health Study II, a randomized trial giving 50 mg beta-carotene supplements or a placebo to 5,956 men older than 65 years, found that longer-term supplementation for at least 15 years provided cognitive benefits 89. The Prevention of Alzheimer’s Disease by Vitamin E and Selenium (PREADViSE) trial followed more than 3,700 men ages 60 and older for six years 90. It did not find that antioxidant supplements of vitamin E or selenium, alone or in combination, protected against dementia compared with a placebo 90.
Can antioxidant supplements prevent early death?
A meta-analysis of 68 antioxidant supplement trials found that taking beta-carotene and vitamin A and E supplements increased the risk of dying 91. Although healthy participants were included in 21 of the trials, most of the studies included people who already had some type of serious illness. It was also difficult to compare interventions because the types of supplements, the dosages taken, and the length of time they were taken varied widely 91. The same authors conducted another systematic review of 78 randomized clinical trials on antioxidant supplements including beta-carotene, vitamin A, vitamin C, vitamin E, and selenium (alone or in combination) 92. Again, the majority of trials included people with various established diseases. The study found that both people who were healthy and those with diseases taking beta-carotene and vitamin E supplements had a higher rate of death 92. The duration of the studies varied widely from one month to 12 years, with varying dosages 92.
Antioxidant Foods
One possible reason why many studies on antioxidant supplements do not show a health benefit is because antioxidants tend to work best in combination with other nutrients, plant chemicals, and even other antioxidants. Therefore, the best way to get additional antioxidants is by eating a healthy diet. Healthy diet includes a mix of colorful fruits and vegetables. Doctors recommend eating a balanced diet that include fresh fruits, vegetables, whole grains, seeds, and nuts. A lot of fresh fruits and vegetables have natural antioxidants. It also contains important minerals, fiber, and other vitamins. For example, a cup of fresh strawberries contains about 80 mg of vitamin C, a nutrient classified as having high antioxidant activity. But a supplement containing 500 mg of vitamin C (667% of the Recommended Dietary Allowance (RDA), RDA is the average daily level of intake sufficient to meet the nutrient requirements of nearly all (97%–98%) healthy individuals) does not contain the plant chemicals (polyphenols) naturally found in strawberries like proanthocyanins and flavonoids, which also possess antioxidant activity and may team up with vitamin C to fight disease. Polyphenols also have many other chemical properties besides their ability to serve as antioxidants. Therefore, eating healthy can help lower your risk of certain diseases. Epidemiological prospective studies show that higher intakes of antioxidant-rich fruits, vegetables, and legumes are associated with a lower risk of chronic oxidative stress-related diseases like cardiovascular diseases, cancer, and deaths from all causes 93, 94, 95. A plant-based diet is believed to protect against chronic oxidative stress-related diseases 6. It is not clear if this protective effect is due to the antioxidants, other substances in the foods, or a combination of both.
The following are nutrients with antioxidant activity and the foods in which they are found:
- Vitamin A. Concentrations of preformed vitamin A are highest in liver, fish, eggs, and dairy products 29. Most dietary provitamin A in the U.S. diet comes from leafy green vegetables, orange and yellow vegetables, tomato products, fruits, and some vegetable oils 29, 96, 97. Vitamin A is routinely added to some foods, including milk and margarine 29, 35. Some ready-to-eat cereals are also fortified with vitamin A.
- Vitamin C is in most fruits and vegetables. Fruits such as berries, oranges, kiwis, lemons, cantaloupes, grapefruits, honeydews, strawberries and papayas provide essential antioxidants. Vegetables such as broccoli, bell peppers, tomatoes, cauliflower, bell peppers (all colors), Brussels sprouts, leafy greens (turnip, mustard, beet, collards), snow peas, and kale are also great choices.
- Vitamin E is in some nuts and seeds. For example, almonds, sunflower seeds, hazelnuts, and peanuts contain vitamin E. It can also be found in green leafy vegetables, such as avocado, Swiss chard, leafy greens (beet, mustard, turnip), spinach (boiled), red peppers and kale, as well as soybean, sunflower, corn, and canola oils.
- Carotenoids (beta-carotene and lycopene): Apricots, asparagus, beets, broccoli, cantaloupe, carrots, bell peppers, kale, mangos, turnip and collard greens, oranges, peaches, pink grapefruit, pumpkin, winter squash, spinach, sweet potato, tangerines, tomatoes, and watermelon
- Beta-carotene is in brightly colored fruits and vegetables. Eat fruits such as peaches, apricots, papayas, mangoes, and cantaloupes. Eat vegetables such as carrots, peas, broccoli, squash, and sweet potatoes. It also is in some green leafy vegetables, such as beet greens, spinach, and kale.
- Lycopene is in many pink and red fruits and vegetables. This includes pink grapefruits, watermelon, apricots, and tomatoes.
- Lutein is in green leafy vegetables such as spinach, collard greens, and kale. You also can find it in broccoli, corn, peas, papayas, and oranges.
- Selenium is in pasta, bread, and grains, including corn, wheat, barley, and brown rice. You can find it in animal products, like beef, fish, shellfish, turkey, and chicken. You also can find it in many nuts, Brazil nuts, legumes, eggs, and cheeses.
- Zinc: Beef, poultry, oysters, shrimp, sesame seeds, pumpkin seeds, chickpeas, lentils, cashews, fortified cereals Phenolic compounds: Quercetin (apples, red wine, onions), catechins (tea, cocoa, berries), resveratrol (red and white wine, grapes, peanuts, berries), coumaric acid (spices, berries), anthocyanins (blueberries, strawberries).
Each antioxidant has a different chemical makeup. Each one provides different health benefits. Too much of one antioxidant can be harmful. Talk to your doctor before changing your diet or taking supplements.
Table 2. High Antioxidant Foods
Antioxidant content mmol/100 g | |
---|---|
African baobab tree, leaves dry, crushed | 48.1 |
Amla (Indian gooseberry), dried | 261.5 |
Apples | 0.4 |
Apples, dried | 3.8 |
Apricots, dried | 3.1 |
Artichoke | 3.5 |
Bilberries, dried | 48.3 |
Black olives | 1.7 |
Blueberry jam | 3.5 |
Broccoli, cooked | 0.5 |
Chilli, red and green | 2.4 |
Curly kale | 2.8 |
Dates, dried | 1.7 |
Dog rose, products of dried hip | 69.4 |
Dog rose, wild, dried | 78.1 |
Dog rose, wild, fresh | 24.3 |
Fruit from the African baobab tree | 10.8 |
Mango, dried | 1.7 |
Moringa Stenopetala, dried leaves, stem | 11.9 |
Moringa Stenopetala, fresh leaves, stem | 3.7 |
Okra/gumbo from Mali, dry, flour | 4.2 |
Oranges | 0.9 |
Papaya | 0.6 |
Plums, dried | 3.2 |
Pomegranate | 1.8 |
Prunes | 2.4 |
Strawberries | 2.1 |
Zereshk, red sour berries | 27.3 |
Table 3. Nuts, legumes and grains antioxidant content
Antioxidant content mmol/100 g | |
---|---|
Barley, pearl and flour | 1 |
Beans | 0.8 |
Bread, with fiber/whole meal | 0.5 |
Buckwheat, white flour | 1.4 |
Buckwheat, whole meal flour | 2 |
Chestnuts, with pellicle | 4.7 |
Crisp bread, brown | 1.1 |
Maize, white flour | 0.6 |
Millet | 1.3 |
Peanuts, roasted, with pellicle | 2 |
Pecans, with pellicle | 8.5 |
Pistachios | 1.7 |
Sunflower seeds | 6.4 |
Walnuts, with pellicle | 21.9 |
Wheat bread, toasted | 0.6 |
Whole wheat bread, toasted | 1 |
Table 4. Spices and herbs antioxidant content
Antioxidant content mmol/100 g | |
---|---|
Allspice, dried ground | 100.4 |
Basil, dried | 19.9 |
Bay leaves, dried | 27.8 |
Cinnamon sticks and whole bark | 26.5 |
Cinnamon, dried ground | 77 |
Clove, dried, whole and ground | 277.3 |
Dill, dried ground | 20.2 |
Estragon, dried ground | 43.8 |
Ginger, dried | 20.3 |
Mint leaves, dried | 116.4 |
Nutmeg, dried ground | 26.4 |
Oregano, dried ground | 63.2 |
Rosemary, dried ground | 44.8 |
Saffron, dried ground | 44.5 |
Saffron, dried whole stigma | 17.5 |
Sage, dried ground | 44.3 |
Thyme, dried ground | 56.3 |
Table 5. Beverages antioxidant content
Antioxidant content mmol/100 g | |
---|---|
Apple juice | 0.27 |
Black tea, prepared | 1 |
Cocoa with milk | 0.37 |
Coffee, prepared filter and boiled | 2.5 |
Cranberry juice | 0.92 |
Espresso, prepared | 14.2 |
Grape juice | 1.2 |
Green tea, prepared | 1.5 |
Orange juice | 0.64 |
Pomegranate juice | 2.1 |
Prune juice | 1 |
Red wine | 2.5 |
Tomato juice | 0.48 |
Antioxidant Supplements
The studies so far are inconclusive but generally don’t provide strong evidence that antioxidant supplements have a substantial impact on disease. There is a question if a nutrient with antioxidant activity can cause the opposite effect with pro-oxidant activity if too much is taken. This is why using an antioxidant supplement with a single isolated substance may not be an effective strategy for everyone. Differences in the amount and type of antioxidants in foods versus those in supplements might also influence their effects. For example, there are eight chemical forms of vitamin E present in foods. However, vitamin E supplements typically only include one form, alpha-tocopherol. Epidemiological prospective studies show that higher intakes of antioxidant-rich fruits, vegetables, and legumes are associated with a lower risk of chronic oxidative stress-related diseases like cardiovascular diseases, cancer, and deaths from all causes 93, 94, 95. A plant-based diet is believed to protect against chronic oxidative stress-related diseases 6. It is not clear if this protective effect is due to the antioxidants, other substances in the foods, or a combination of both.
Polyphenols
Polyphenols are naturally occurring compounds such as phenolic acids, flavonoids, stilbenes, and lignans found largely in the fruits, vegetables, cereals and beverages 98, 99, 100, 101, 102, 103. Fruits like grapes, apple, pear, cherries and berries contains up to 200–300 mg polyphenols per 100 grams fresh weight 104. The products manufactured from these fruits, also contain polyphenols in significant amounts. Cereals, dry legumes, chocolate and beverages, such as tea, coffee, or wine also contribute to the polyphenolic intake 105, 106. Typically a glass of red wine or a cup of tea or coffee contains about 100 mg polyphenols.
Polyphenols are secondary metabolites of plants and are generally involved in defense against ultraviolet radiation or aggression by pathogens and may also contribute to the bitterness, astringency of the food. Researchers have explored that these molecules are very good antioxidants and may neutralize the destructive reactivity of undesired reactive oxygen/nitrogen species produced as byproduct during metabolic processes in the body. Epidemiological studies have revealed that polyphenols provide a significant protection against development of several chronic diseases such as cardiovascular diseases (CVDs), cancer, diabetes, infections, aging, asthma, etc 104.
Towards the end of 20th century, epidemiological studies and associated meta-analyses strongly suggested that long term consumption of diets rich in plant polyphenols offered some protection against development of cancers, cardiovascular diseases, diabetes, osteoporosis and neurodegenerative diseases 107, 108. Whether acting in the gastrointestinal tract or in the liver, the potent antioxidant effects of polyphenols are widely accepted as health promoting 109, 110. Antivira, antibacterial, anti-inflammatory, neuroprotective, and anticarcinogenic effects have also been attributed to polyphenols 111, 112, 113, 114. Polyphenols and other food phenolics are the subject of increasing scientific interest because of their possible beneficial effects on human health.
Polyphenols such as resveratrol, epigallocatechin gallate (EGCG), and curcumin have been acknowledged for having beneficial effects on cardiovascular health, while some have also been shown to be protective in aging.
Figure 1. Polyphenols
Footnote: Main categories of polyphenols. Polyphenols are classified as phenolic acids, flavonoids, stilbenes, and lignans based on their structure and the numbering of carbons.
[Source 99 ]Figure 2. Polyphenols Health Benefits
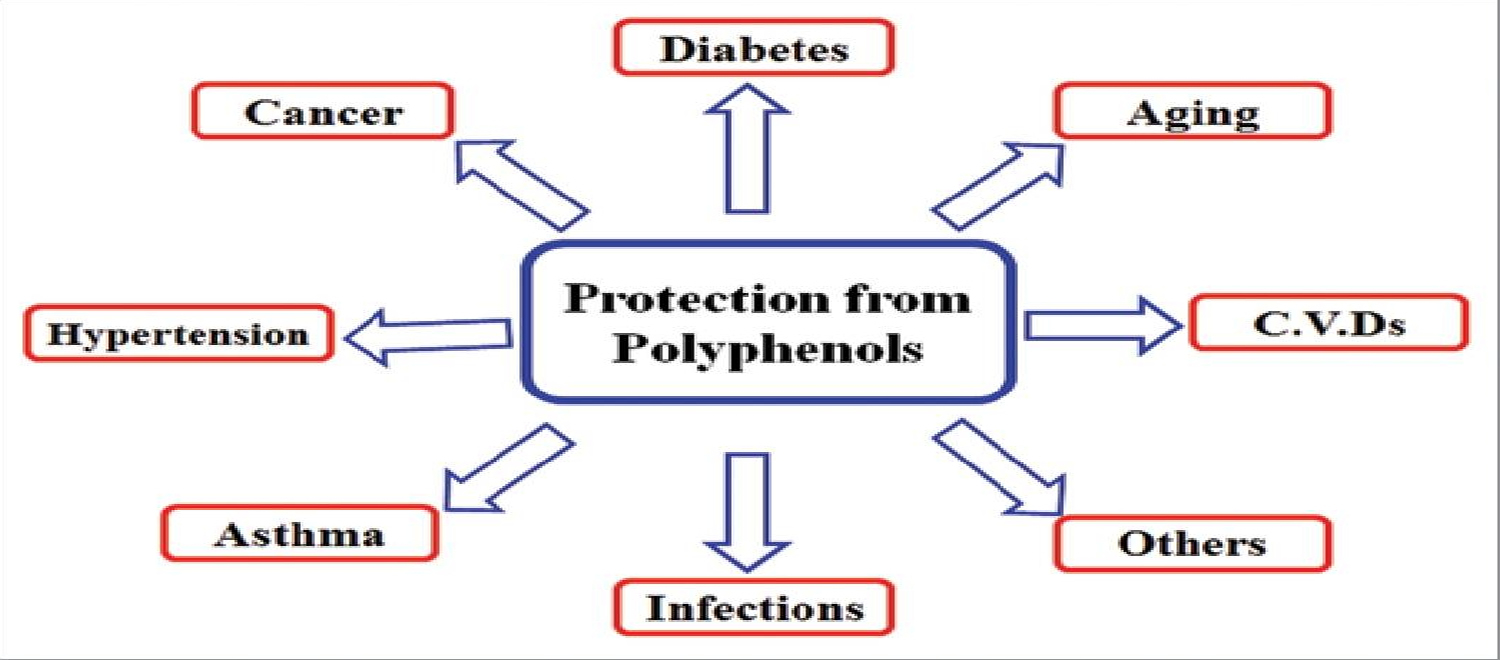
Table 6. Polyphenols Rich Foods
Source (serving size) | Polyphenol content | ||
---|---|---|---|
By weight or volume | By serving | ||
mg/kg fresh weight (or mg/L) | mg/serving | ||
Hydroxybenzoic acids | Blackberry (100 g) | 80–270 | 8–27 |
Protocatechuic acid | Raspberry (100 g) | 60–100 | 6–10 |
Gallic acid | Black currant (100 g) | 40–130 | 4–13 |
p-Hydroxybenzoic acid | Strawberry (200 g) | 20–90 | 4–18 |
Hydroxycinnamic acids | Blueberry (100 g) | 2000–2200 | 200–220 |
Caffeic acid | Kiwi (100 g) | 600–1000 | 60–100 |
Chlorogenic acid | Cherry (200 g) | 180–1150 | 36–230 |
Coumaric acid | Plum (200 g) | 140–1150 | 28–230 |
Ferulic acid | Aubergine (200 g) | 600–660 | 120–132 |
Sinapic acid | Apple (200 g) | 50–600 | 10–120 |
Pear (200 g) | 15–600 | 3–120 | |
Chicory (200 g) | 200–500 | 40–100 | |
Artichoke (100 g) | 450 | 45 | |
Potato (200 g) | 100–190 | 20–38 | |
Corn flour (75 g) | 310 | 23 | |
Flour: wheat, rice, oat (75 g) | 70–90 | 5–7 | |
Cider (200 mL) | 10–500 | 2–100 | |
Coffee (200 mL) | 350–1750 | 70–350 | |
Anthocyanins | Aubergine (200 g) | 7500 | 1500 |
Cyanidin | Blackberry (100 g) | 1000–4000 | 100–400 |
Pelargonidin | Black currant (100 g) | 1300–4000 | 130–400 |
Peonidin | Blueberry (100 g) | 250–5000 | 25–500 |
Delphinidin | Black grape (200 g) | 300–7500 | 60–1500 |
Malvidin | Cherry (200 g) | 350–4500 | 70–900 |
Rhubarb (100 g) | 2000 | 200 | |
Strawberry (200 g) | 150–750 | 30–150 | |
Red wine (100 mL) | 200–350 | 20–35 | |
Plum (200 g) | 20–250 | 4–50 | |
Red cabbage (200 g) | 250 | 50 | |
Flavonols | Yellow onion (100 g) | 350–1200 | 35–120 |
Quercetin | Curly kale (200 g) | 300–600 | 60–120 |
Kaempferol | Leek (200 g) | 30–225 | 6–45 |
Myricetin | Cherry tomato (200 g) | 15–200 | 3–40 |
Broccoli (200 g) | 40–100 | 8–20 | |
Blueberry (100 g) | 30–160 | 3–16 | |
Black currant (100 g) | 30–70 | 3–7 | |
Apricot (200 g) | 25–50 | 5–10 | |
Apple (200 g) | 20–40 | 4–8 | |
Beans, green or white (200 g) | 10–50 | 2–10 | |
Black grape (200 g) | 15–40 | 3–8 | |
Tomato (200 g) | 2–15 | 0.4–3.0 | |
Black tea infusion (200 mL) | 30–45 | 6–9 | |
Green tea infusion (200 mL) | 20–35 | 4–7 | |
Red wine (100 mL) | 2–30 | 0.2–3 | |
Flavones | Parsley (5 g) | 240–1850 | 1.2–9.2 |
Apigenin | Celery (200 g) | 20–140 | 4–28 |
Luteolin | Capsicum pepper (100 g) | 5–10 | 0.5–1 |
Flavanones | Orange juice (200 mL) | 215–685 | 40–140 |
Hesperetin | Grapefruit juice (200 mL) | 100–650 | 20–130 |
Naringenin | Lemon juice (200 mL) | 50–300 | 10–60 |
Eriodictyol | |||
Isoflavones | Soy flour (75 g) | 800–1800 | 60–135 |
Daidzein | Soybeans, boiled (200 g) | 200–900 | 40–180 |
Genistein | Miso (100 g) | 250–900 | 25–90 |
Glycitein | Tofu (100 g) | 80–700 | 8–70 |
Tempeh (100 g) | 430–530 | 43–53 | |
Soy milk (200 mL) | 30–175 | 6–35 | |
Monomeric flavanols | Chocolate (50 g) | 460–610 | 23–30 |
Catechin | Beans (200 g) | 350–550 | 70–110 |
Epicatechin | Apricot (200 g) | 100–250 | 20–50 |
Cherry (200 g) | 50–220 | 10–44 | |
Grape (200 g) | 30–175 | 6–35 | |
Peach (200 g) | 50–140 | 10–28 | |
Blackberry (100 g) | 130 | 13 | |
Apple (200 g) | 20–120 | 4–24 | |
Green tea (200 mL) | 100–800 | 20–160 | |
Black tea (200 mL) | 60–500 | 12–100 | |
Red wine (100 mL) | 80–300 | 8–30 | |
Cider (200 mL) | 40 | 8 |
Table 7. Plant, fruit and vegetable-based Polyphenols separated according to differences in chemical structure
Phenolic Family | Dietary Sources | Agri-Food Residue or Non-Edible Sources |
---|---|---|
Phenolic acid | ||
Hydroxycinnamic acid | Almonds, cereals, cherries, citrus juices and fruits, coffee, corn flour, peaches, plums, potato, rice flour, spinach, tomatoes, and wheat flour. | Apple pomace, artichoke wastewaters, banana peel, citrus peels, olive mill wastewaters, and spent coffee grounds. |
Hydroxybenzoic acid | Black currant, blackberry, cereals, coffee, cowpea, oilseeds, raspberry, and wheat flour. | Citrus peels, grape pomace, residual brewing yeast, squash shells and seeds. |
Flavonoids | ||
Flavonols | Apples, apricot, arugula, beans, capers, cloves, leeks, lettuce, onions, saffron, and tomatoes. | Apples peels, banana peels, grape pomace and seeds, guava peels and seeds, onion peels, olive leaves and pomace. |
Flavones | Artichoke, black olive, celery, citrus fruits, oregano, peanut, parsley, pepper, spinach. | Artichokes steams, camu-camu peels and peanut skin and shell |
Isoflavones | Red clover, soybeans, soymilk and soy flour. | Soy processing waste and peanut skins and shells. |
Flavanols | Grapes, apples, tomatoes, leeks, lettuces, curly kale, berries, onions, red grapes, beans, green and black, cider, tea, red wine. | Appel peel, grape seeds, peels and pomace and tea by-products. |
Anthocyanins | Eggplant, grape, plums, pomegranate, raspberries, red and back-currants, red cabbage, red wine, strawberries, and radish. | Grape skins and seeds, grape pomace and floral tepals (saffron). |
Flavanones | Citrus juices, citrus fruits, peppermint, fennel and rosemary. | Banana peels, citrus seeds, peels and pomace and residual brewing yeast. |
Stilbenes | Almonds, grapes, red wine and berries. | Avocado peels, grape skins, seeds, pomace and stems. |
Lignans | Broccoli, flaxseed, kale, lentil, sesame seeds, tea, wine and wheat. | Coffee, soybeans and wine residues. |
Tannins | ||
Hydrolizable tannins | Pomegranates, raspberries and tea | Pomegranate peels and seeds and tea by-products. |
Condense tannins | Apples, chestnut, grapes, pears, peaches, and hazelnuts. | Grape seeds |
Phlorotannins | – | Brown seaweed |
Complex tannins | – | Cork by-products (e.g., tree bark) |
Resveratrol
Resveratrol (3,5,4’-trihydroxystilbene) is a natural plant-derived polyphenol found naturally in red grape skins, grape juice, mulberries, blueberries, blackberries, huckleberries, pomegranates, almonds, pistachios, peanuts, dark chocolate, tea and Japanese knotweed (Polygonum cuspidatum) 116, 117, 118, 119, 120, 121, 122. It seems the roots of Japanese knotweed (Polygonum cuspidatum), a plant which is widely used in Chinese medicine, has abundant resveratrol 123, 124. The root extracts of field-grown Japanese knotweed (Polygonum cuspidatum) are currently used to manufacture most of the resveratrol sold on the global market 125. In plants, resveratrol is produced as a defense mechanism, where it has a role in defense against ultraviolet (UV) related injuries, diseases, physical damage and pathogenic attacks 126, 127. Resveratrol was first isolated from the root of white hellebore lily (Veratrum grandiflorum O. Loes) in 1940 128, and detected in Vitis vinifera grapevines in 1976 129. The discovery of resveratrol in red wine in 1992 was used to explain “French Paradox”, assumed to be responsible for low rates of coronary heart disease death in some areas of France, despite the elevated intake of fat and cholesterol through the daily diet 130, 119, 131. However, a definitive correlation between resveratrol in red wine and low rates of coronary heart disease death may not be there as several other factors could have influenced the “French paradox” such as dietary habits and genetics as well 132.
Resveratrol is a member of the stilbene family with two phenol rings that are joined by an ethylene bridge 133, 134. Due to the presence of the central ethylene moiety in its structure, resveratrol has two possible stereoisomers: cis-resveratrol and trans-resveratrol 135. The naturally occurring resveratrol is usually its trans-isomer (E-configuration) and the majority of reported health benefits are attributed to the trans-resveratrol form 136. When exposed to UV and visible light, trans-resveratrol converts to cis-resveratrol (Z) by photo-isomerization 137. Cis-resveratrol is not well studied despite the findings from in vivo intestinal epithelial model studies that trans-resveratrol converts to cis-resveratrol in vivo 138 and that cis-resveratrol is also a biologically active form 139. The glucose-bound form of resveratrol, piceid also called Polydatin, is the major resveratrol derivative in food sources and it is converted to trans-resveratrol by hydrolysis 136. As a result of its hydroxyl groups and double C-C bond, resveratrol is sensitive to light, oxygen, alkaline pH and increased temperature 140.
Resveratrol has been shown to possess a variety of health-promoting effects, including anti-inflammatory, immunomodulating activity, liver-protective, neuroprotective, and antioxidant activities 141, 142, 143, 144, 145, 146. In addition, resveratrol is known to protect liver cells from oxidative stress by increasing the activity of an antioxidant enzyme and altering the gene expressions 118. Resveratrol is also reported to affect various antioxidant enzymes, affecting their expression and activity 147, 148, 149 and improve urinary protein excretion, kidney oxidative stress, and kidney dysfunction 150. In the recent years, resveratrol received renewed interest as several findings implicated resveratrol could increase cell survival and slow aging in yeast and later in mice by activating a “longevity” gene known as SIRT1 capable of mimicking the effects of calorie restriction, and regulating longevity in lower organisms 151, 152, 153. But the dose of resveratrol administered in experiments is always much higher than you’d normally consume in a daily diet. You would need to drink a hundred to a thousand glasses of red wine to equal the doses that improve health in mice 154. Resveratrol has been revealed to sensitize numerous resistant cancer cells to anticancer drugs through overcoming chemoresistance mechanisms 155 and promoting the efficacy of anticancer drugs at a low dosage 156. It has been reported that resveratrol has cytotoxic effects against numerous tumor cells, such as lymphoid, myeloid, breast, cervix, skin, ovary, prostate, stomach, colon, liver, and thyroid carcinoma cells 157, 158, 159.
Given the worldwide increase in age-related metabolic diseases and obesity, resveratrol supplement has been promoted as a treatment for many conditions — including aging. The first real interest in this compound came when in 1992 resveratrol was postulated to explain some of the cardio-protective effects of red wine 160. Five years later, in 1997, Jang and colleagues reported resveratrol to work as a chemo-preventive agent, by the ability to inhibit carcinogenesis at multiple stages in lab mice 161. Meanwhile, anti-inflammatory and anti-oxidant properties were identified for resveratrol 162, 163.
Resveratrol supplements have not been well studied in people. Scientists don’t really know what benefits and risks resveratrol might have as clinical studies on resveratrol toxicity and adverse effects are relatively scarce 164, 165. Also, when taking resveratrol with anticoagulant and antiplatelet drugs, it could enhance both bruising and bleeding risk due to resveratrol ability to hinder human platelet aggregation in vitro 164. On the other hand, it is speculated that higher resveratrol doses could compete with other polyphenols for transporters, reducing both their uptake and potential synergistic effects 166.
Nonetheless, resveratrol has become a popular supplement. People use it for many different conditions. Some take resveratrol supplements to try to prevent or treat serious diseases, like cancer or heart disease. Others hope that they will slow the aging process. For now, these uses are unsupported by evidence.
Figure 3. Resveratrol chemical structure
Footnote: The chemical structure of two geometric isomers of resveratrol: cis-resveratrol (Z) and trans-resveratrol (E). Trans-resveratrol can undergo to cis-resveratrol when exposed to UV irradiation. Trans-resveratrol is dominant in terms of its prevalence and different biological activities are attributed, namely in inducing cellular responses such as cell cycle arrest, differentiation, apoptosis, and to enhance cancer cells anti-proliferation 167.
[Source 132 ]Table 8. Food sources of Resveratrol
Food source | Resveratrol amount |
---|---|
Red grape wine | 0.27 mg/100 mL |
Rose grape wine | 0.12 mg/100 mL |
White grape wine | 0.04 mg/100 mL |
Muscadine grape red wine | 1.41–4.41 mg/100 mL |
Lingonberry | 3 mg/100 g FW |
Cranberry | 1.92 mg/100 g FW |
Redcurrant | 1.57 mg/100 g FW |
Bilberry | 0.67 mg/100 g FW |
Strawberry | 0.35 mg/100 g FW |
Black grapes | 0.15 mg/100 g FW |
Green grapes | 0.02 mg/100 g FW |
Dark chocolate | 0.04 g/100 g |
Pistachio | 0.11 mg/100 g |
Peanut | 0.04 mg/100 g |
Glutathione
Glutathione (GSH) is a tripeptide comprised of three amino acids, cysteine, glycine, and glutamic acid, is a critical component of the body’s primary antioxidant defense mechanism that is found in relatively high concentrations in most cells 168, 169, 170, 171, 172, 173, 174. Glycine, cysteine, and glutamic acid combine to form glutathione (GSH) in a two-step biochemical reaction. First, cysteine is conjoined with glutamate through the action of glutamate cysteine ligase to produce gamma-glutamylcysteine, which proceeds to link with glycine via glutathione synthase 175. Therefore, the human body requires all three amino acids (glycine, cysteine, and glutamic acid) and adequate enzymatic function to make sufficient quantities of glutathione 176. Cysteine is a sulfur amino acid, which might imply that consuming sulfur-rich foods, especially those containing the sulfur amino acids, may also support glutathione synthesis 177, 178. Cysteine is frequently identified as rate-limiting, which provides the rationale of why N-acetylcysteine (NAC) is frequently studied and suggested as a supplement for glutathione support 176, yet a review of the data indicates its use may be inconclusive or indeterminate 179.
Glutathione occurs in 2 free forms: the reduced (GSH) thiol and the oxidized (GSSG) disulfide forms (oxidized glutathione is actually 2 reduced glutathiones bound together at the sulfur atoms) 180, 172. The ratio of reduced glutathione (GSH) and the oxidized glutathione (GSSG) determines cell redox status of cells 172. Under physiological conditions, more than 98% of total glutathione occurs in the reduced GSH form 181, 182. Healthy cells at rest have a GSH/GSSG ratio >100 while the ratio drops to 1 to 10 in cells exposed to oxidant stress 172. Glutathione is called an intracellular antioxidant (a free radical scavenger and a detoxifying agent) because of its role in protecting cells from the damaging effects of unstable and harmful oxygen-containing molecules that are generated during energy production 183, 184, 185, 186. Glutathione plays a pivotal role against free radicals and oxidative stress, by maintaining redox balance, enhancing metabolic detoxification, and regulating the immune system 172, 187. Glutathione directly scavenges diverse oxidants: superoxide anion, hydroxyl radical, nitric oxide, and carbon radicals. Glutathione catalytically detoxifies: hydroperoxides, peroxynitrites, and lipid peroxides 188. Another way glutathione protects cells from oxidants is through recycling of vitamins C and E 172.
Glutathione is also important as a cofactor (a compound that is essential for the activity of an enzyme) for the enzyme glutathione peroxidase, in the uptake of amino acids, and in the synthesis of leukotrienes. As a substrate for glutathione S-transferase, glutathione reacts with a number of harmful chemical species, such as halides, epoxides and free radicals, to form harmless inactive products 189, 190. In red blood cells, these reactions prevent oxidative damage through the reduction of methemoglobin and peroxides. Glutathione is also involved in the formation and maintenance of disulfide bonds in proteins and in the transport of amino acids across cell membranes 191. Glutathione also plays a role in processing medications and cancer-causing compounds (carcinogens), and building DNA, proteins, and other important cellular components 192, 189, 182. The ability to upregulate glutathione synthesis in response to demands is hypothesized to be an important determinant of cell survival and provides protection of cells from many forms of stress 193. Evidence from several rat 194, 195, dog 196, 197 and human studies 198, 199, 200, 201, 202 suggests that glutathione levels in organs, red blood cells and plasma decline with age. During aging, glutathione levels appear to decline in a number of tissues, thereby putting cells at increased risk of succumbing to stress 193. The evidence for such a decline is strongest in the brain where glutathione loss is implicated in both Parkinson’s disease and in neuronal injury following stroke 193. Age-related increases in oxidative stress have been associated with chronic inflammation, heart and blood vessel (cardiovascular) disease and neurodegenerative disease, osteoarthritis and type 2 diabetes in humans 203, 204, 205.
Glutathione is ubiquitous in mammalian cells ranging in 1–10 mM concentrations 176. The largest amount of glutathione is found in the liver (5 – 10 mM), lining of the lungs, kidneys, heart and brain. Glutathione is necessary for the liver to detoxify toxic substances. Glutathione, especially in the liver, binds with toxic chemicals in order to detoxify them. It is also important as a hydrophilic molecule that is added to lipophilic toxins and waste in the liver during biotransformation before they can become part of the bile. Glutathione is also needed for the detoxification of methylglyoxal, a toxin produced as a by-product of metabolism. This detoxification reaction is carried out by the glyoxalase system. Glyoxalase I catalyzes the conversion of methylglyoxal and reduced glutathione to S-D-Lactoyl-glutathione. Glyoxalase II catalyzes the hydrolysis of S-D-Lactoyl-glutathione to glutathione and D-lactate.
Studies in aged humans and rats have shown that dietary supplementation with the precursors of glutathione, cysteine and/or glycine, can restore glutathione synthesis and reduce levels of oxidative stress. Sekhar et.al. 202 observed that significantly lower concentrations of red blood cell glutathione in elderly human subjects, compared with younger control subjects, could be re-established by dietary glycine (1·33 mM/kg/day) and cysteine (0·81 mM/kg/day) supplementation for 14 days. Not only was red blood cell glutathione significantly increased, but plasma reactive oxygen metabolites, plasma F2-isoprostanes (F2-IsoPs) and lipid peroxides were significantly reduced 202. A recent intervention study by Kumar et al. 206 supplementing 100 mg/kg/day glycine and cysteine, provided as N-acetylcysteine (100 mg/kg/day), improved glutathione deficiency, oxidative stress, mitochondrial dysfunction, inflammation and physical function. Perturbations to glutathione homoeostasis have also been explored in disease models. Linking HIV to glutathione deficiency, Nguyen et al. 207 explored supplementation with both glutathione precursors in HIV infected male patients. The authors observed 2 weeks of oral supplementation of 1·33 mM/kg/day glycine and 0·81 mM/kg/day cysteine restored glutathione synthesis, improved mitochondrial fat and carbohydrate oxidation, insulin sensitivity, body composition, muscle strength and dyslipidemia 207.
Critical roles of glutathione 172, 187
- Direct chemical neutralization of singlet oxygen, hydroxyl radicals, and superoxide radicals
- Cofactor for several antioxidant enzymes
- Regeneration of vitamins C and E
- Neutralization of free radicals produced by Phase I liver metabolism of chemical toxins
- One of approximately 7 liver Phase 2 reactions, which conjugate the activated intermediates produced by Phase 1 to make them water soluble for excretion by the kidneys
- Transportation of mercury out of cells and the brain
- Regulation of cellular proliferation and apoptosis
- Vital to mitochondrial function and maintenance of mitochondrial DNA (mtDNA)
Considering how important glutathione is to health, many researchers have looked for ways to increase intracellular and intramitochondrial levels. The good news is that there are several effective strategies. The first, of course, is to decrease the need for glutathione, which means decreasing toxic load. The most obvious is limiting alcohol consumption 208. The strategy is to directly administer glutathione. This can be done orally, topically, intravenously (IV), intranasally, or in nebulized form. Glutathione administered intravenously, inhaled, and ingested intranasally increases systemic glutathione levels 209. Intravenous glutathione has a short half-life but has shown at least short-term efficacy in several diseases. Oral glutathione administration is controversial; while most research shows that oral glutathione does not increase red blood cell glutathione, there are a few studies that show efficacy 210. Oral and transdermal liposomal glutathione show promise, but research is early 211.
Cysteine availability is the rate-limiting step in the body production of glutathione 172. While oral cysteine does not make it through the digestive track, supplemental cysteine in the form of whey or N-acetylcysteine (NAC) is effective at raising glutathione levels. 1000 mg/day of N-acetylcysteine (NAC) will substantially increase glutathione in virtually all patients 212. Although N-acetylcysteine (NAC) is promising as a supplement to both boost glutathione levels and potentially mitigate some of the issues related to oxidative stress 213, 214, the research is not conclusive and some of the findings are disease specific 215, 216, 217, 218. There have also been studies with no significant impact by taking N-acetylcysteine (NAC). Moreover, it has been suggested that N-acetylcysteine (NAC) may work synergistically with other supplemental nutrients. For example, it has been postulated that glycine may be as important as cysteine when it comes to glutathione production, especially when concurrently supplemented with N-acetylcysteine (NAC) 175. While further studies are required, it may be the better approach to supplement with both cysteine and glycine to see a boost in glutathione, especially among those who may not have adequate quantities of the amino acids or require higher levels of glutathione. It is also important to note that N-acetylcysteine (NAC) has antioxidant properties in addition to being able to provide cysteine for glutathione synthesis 170. It is unclear if the effects of N-acetylcysteine (NAC) on oxidative stress are due to these antioxidant properties or due to increased glutathione synthesis 170.
In one study 219 on five people with mild to moderate Parkinson’s disease and three controls, a high dose of N-acetylcysteine (NAC) (3000 mg taken orally twice daily) for a period of four weeks led to an increase of cysteine levels and antioxidant measures, with no commensurate improvement in oxidative stress measurements (4-hydroxynonenal and malondialdehyde) nor did it increase the level of glutathione in the brain. Additionally, some of the participants experienced an exacerbation of Parkinson’s symptoms that were alleviated upon stopping the N-acetylcysteine (NAC) supplementation 219. Another study 220 looking at those with neurodegenerative disorders found that a single intravenous dose of N-acetylcysteine (NAC) led to an increase of the blood reduced glutathione (GSH)/oxidized glutathione (GSSG) ratio and levels of glutathione in the brain. Those who had the greatest percent change in that ratio also had a greater percent change in their levels of glutathione in their brain. While the study was too small and of too short duration to make any conclusions about the role of N-acetylcysteine (NAC) in these conditions, what is notable is that through intravenous administration of N-acetylcysteine (NAC), brain levels could be altered.
A 12-week clinical trial 221 with children (n = 31) with autism administered 60 mg/kg/day in three doses (maximum dose of 4200 mg/day) found that although there was no significant impact on the social impairment associated with autism, there was a significant impact on boosting the glutathione levels in the children. Similar to other studies, more needs to be explored as to how N-acetylcysteine (NAC) influences glutathione levels, factors that modify individual response to supplementation and how symptomatology interrelates. GST polymorphisms may play a role in the efficacy of N-acetylcysteine (NAC). In one study 222 investigating the impact of N-acetylcysteine (NAC) on noise-induced hearing loss in men (n = 53) taking 1200 mg per day for 14 days led to a significant reduction of noise-induced temporary threshold shift, or the amount of hearing loss after a period of heightened noise exposure in a work setting relative to baseline, or pre-shift levels. When the participants were grouped according to their GST genotypes, the researchers found that only those with the null genotypes experienced a significant effect from taking N-acetylcysteine (NAC).
For the rare patient who reacts to N-acetylcysteine (NAC), S-adenosylmethionine (SAMe) can be used 223. Do not use methionine as it will increase homocysteine 172. Supplementing with N-acetylcysteine (NAC) (600 mg/day for 4 weeks) decreases gamma-glutamyl transferase (GGT) 25%, suggesting that increasing de novo synthesis decreases the need for gamma-glutamyl transferase (GGT) recycling 224. For those looking for a nonsupplemental solution, 500 mL of alcohol-free beer per day raises red blood cell glutathione by 29% 225. There are many other examples of foods that increase glutathione. For example, 83 g/day of almonds increases glutathione in smokers by 16% and decreases their DNA damage by 29% 226. Lastly, people who meditate regularly have 20% higher levels of glutathione 227.
Diseases associated with glutathione depletion 173, 228, 172:
- Neurodegenerative disorders (Alzheimer’s disease, Parkinson’s disease, multiple sclerosis and Huntington’s disease, amyotrophic lateral sclerosis, Friedreich’s ataxia) 229, 219, 230, 231, 232
- Mental health disorders (schizophrenia and bipolar disorder) 233
- Pulmonary disease (COPD, asthma, and acute respiratory distress syndrome [ARDS])
- Immune diseases (autoimmune disease), human immunodeficiency virus (HIV) and acquired immune deficiency syndrome (AIDS) 234
- Cardiovascular diseases (hypertension, myocardial infarction, cholesterol oxidation) 235
- Chronic age-related diseases (cataracts, macular degeneration, hearing impairment, and glaucoma)
- Liver disease 236
- Diabetes and uncontrolled diabetes 237, 238
- Cystic fibrosis 239
- Aging process 240. A representative study of elderly found that higher glutathione levels were associated with higher levels of physical health, fewer illnesses, and higher levels of self-rated health 241. As might be expected, glutathione status has been found to parallel telomerase activity, an important indicator of lifespan 242. This depletion of glutathione also shows up as progressive loss of mitochondrial function due to accumulation of damage to mitochondrial DNA (mtDNA) 243. The ability of animal species to protect their mitochondrial DNA (mtDNA) is directly proportional to longevity 244.
- Cancer 245
- Infertility in both men and women 246
- Systemic lupus erythematosus (SLE) 247
Direct administration and promotion of production of glutathione have been used effectively in a wide range of diseases: Parkinson’s, peripheral obstructive arterial disease, cystic fibrosis, emphysema, COPD, preterm infants autism, contrast-induced nephropathy, chronic otitis media, lead exposure, nail biting, nonalcoholic fatty liver disease, exercise-induced fatigue 248, 249, 250, 251, 252, 211, 253, 254, 255, 256, 257..
Glutathione is known as a substrate in both conjugation reactions and reduction reactions, catalyzed by glutathione S-transferase enzymes in cytosol, microsomes, and mitochondria. However, it is also capable of participating in non-enzymatic conjugation with some chemicals, as in the case of N-Acetyl-P-Benzoquinone Imine (NAPQI), the reactive cytochrome P450-reactive metabolite formed by acetaminophen, that becomes toxic when glutathione is depleted by an overdose of acetaminophen. Glutathione in this capacity binds to N-Acetyl-P-Benzoquinone Imine (NAPQI) as a suicide substrate and in the process detoxifies it, taking the place of cellular protein thiol groups which would otherwise be covalently modified; when all glutathione has been spent, NAPQI begins to react with the cellular proteins, killing the cells in the process 258. The preferred treatment for an overdose of acetaminophen is the administration (usually in atomized form) of N-acetylcysteine (NAC), which is used by cells to replace spent oxidized glutathione (GSSG) and renew the usable reduced glutathione (GSH) pool.
Glutathione conjugates to drugs to make them more soluble for excretion, is a cofactor for some enzymes, is involved in protein disulfide bond rearrangement and reduces peroxides.
Glutathione is also important in red and white blood cell formation and throughout the immune system.
Glutathione participates in leukotriene synthesis and is a cofactor for the enzyme glutathione peroxidase.
Glutathione is produced exclusively in the cytosol and actively pumped into mitochondria. Glutathione is made available in cells in 3 ways (Figures 2, 3 and 4) 172:
- Three conditionally essential amino acids, glycine, cysteine, and glutamic acid combine to form glutathione (GSH) via a 2-step process catalyzed by the enzymes glutamate cysteine ligase (GCL) and glutathione synthetase (requires ATP). First, cysteine is conjoined with glutamate through the action of glutamate cysteine ligase (GCL) to produce gamma-glutamylcysteine, which proceeds to link with glycine via glutathione synthase 175. Therefore, the human body requires all three amino acids (glycine, cysteine, and glutamic acid) and adequate enzymatic function to make sufficient quantities of glutathione 176. Cysteine is a sulfur amino acid, which might imply that consuming sulfur-rich foods, especially those containing the sulfur amino acids, may also support glutathione de novo synthesis 177, 178.
- Regeneration of oxidized glutathione (GSSG) to reduced glutathione (GSH) by glutathione reductase (requires NADPH).
- Recycling of cysteine from conjugated glutathione via GGTP (requires NADPH).
All 3 glutathione synthesis pathways require energy. The rate of glutathione synthesis, regeneration, and recycling is determined primarily by 3 factors (Figure 3) 259:
- De novo glutathione synthesis is primarily controlled by the cellular level of the amino acid cysteine, the availability of which is the rate-limiting step.
- The enzymes glutamate cysteine ligase (GCL) activity is in part regulated by glutathione feedback inhibition.
- If glutathione is depleted due to oxidative stress, inflammation, or exposure to xenobiotics, de novo synthesis of glutathione is upregulated primarily by increasing availability of cysteine through recycling of oxidized glutathione (GSSG).
Under physiological conditions, glutathione is mainly present in the cytoplasm (inside cells) in the reduced form (GSH), which is also the biologically active form. Reduced glutathione (GSH) is less easily oxidized than its precursors, cysteine and gamma-glutamylcysteine; the fully oxidized form with a disulfide between two identical glutathione molecules (GSSG) represents less than 1% of the total glutathione pool in the cell 260. Reduced glutathione (GSH) concentration in human cells typically ranges from 0.1 to 10 mmol/L, being most focused in the liver (up to 10 mmol/L), spleen, kidney, lens of the eyes, red blood cells, and white blood cells 261, 262, wherein its depletion and/or altered level are associated with various diseases, including cancer, cardiovascular, inflammatory, immune, metabolic, and neurodegenerative diseases 173. Maintaining optimal reduced glutathione (GSH):oxidized glutathione (GSSG) ratios in the cell is critical to survival; hence, tight regulation of this system is important 263.
Natural dietary sources of glutathione include fresh fruits, vegetables, and nuts. Tomatoes, avocados, oranges, walnuts and asparagus are some of the most common foods that help to increase levels of glutathione in the body. Whey protein is another rich source of glutathione and has been used to enhance systemic glutathione levels in cystic fibrosis 264.
Glutathione is synthesized by glutathione synthetase from glutamine and cysteine in a two-step process involving the enzymes glutathione synthatase and glutamate-cysteine ligase 265. Like cysteine, glutathione contains the crucial thiol (-SH) group that makes it an effective antioxidant 266. There are virtually no living organisms on this planet-animal or plant whose cells don’t contain some glutathione 266. Scientists have speculated that glutathione was essential to the very development of life on earth 266. People who are born with glutathione synthetase deficiency prevents their body from producing glutathione 267, 268. People with glutathione synthetase deficiency do not have enough of the molecule called glutathione synthetase, which helps the body produce glutathione. People with glutathione synthetase deficiency can have mild, moderate, or severe disease. The signs and symptoms of glutathione synthetase deficiency may include anemia, the buildup of too much acid in the body (metabolic acidosis), frequent infections, and symptoms caused by problems in the brain including seizures, intellectual disability, and loss of coordination (ataxia).
Glutathione synthetase deficiency is a very rare autosomal recessive disorder that has only been described in more than 80 individuals worldwide 269, 270. Glutathione synthetase deficiency is caused by genetic changes (pathogenic variants or mutations) in the GSS gene 192. The GSS gene provides instructions for making glutathione synthetase enzyme. The glutathione synthetase enzyme is involved in a process called the gamma-glutamyl cycle, which takes place in most of the body’s cells. The gamma-glutamyl cycle is necessary for producing a molecule called glutathione. Mutations in the GSS gene prevent cells from making adequate levels of glutathione, leading to the signs and symptoms of glutathione synthetase deficiency.
Glutathione synthetase deficiency is inherited in an autosomal recessive pattern, which means both copies of the GSS gene in each cell have mutations. Each parent is a carrier which means they have a pathogenic variant in only one copy of the GSS gene. The parents (carriers of an autosomal recessive disease) of an individual with glutathione synthetase deficiency each carry one copy of the mutated gene, but they typically do not show signs and symptoms of the condition. When two carriers of an autosomal recessive disease have children, there is a 25% (1 in 4) chance to have a child who has the disease.
Mild glutathione synthetase deficiency usually results in the destruction of red blood cells (hemolytic anemia) 192, 271. In addition, affected individuals may release large amounts of a compound called 5-oxoproline in their urine (5-oxoprolinuria) 271. This compound builds up when glutathione is not processed correctly in cells. As 5-oxoproline is a highly acidic compound, it causes metabolic acidosis 272.
Individuals with moderate glutathione synthetase deficiency may experience symptoms beginning shortly after birth including hemolytic anemia, 5-oxoprolinuria, and elevated acidity in the blood and tissues (metabolic acidosis) 192.
In addition to the features present in moderate glutathione synthetase deficiency, individuals affected by the severe form of glutathione synthetase deficiency may experience neurological symptoms. These problems may include seizures; pathological electroretinograms and retinal pigmentation; a generalized slowing down of physical reactions, movements, and speech (psychomotor retardation); intellectual disability; spasticity, intention tremors and a loss of coordination (ataxia) 192, 273. Some people with severe glutathione synthetase deficiency also develop recurrent bacterial infections 271.
The diagnosis of a metabolic disorder such as glutathione synthetase deficiency may be suspected when a doctor observes signs of glutathione synthetase deficiency including metabolic acidosis. A doctor may order tests to confirm the diagnosis including enzyme assays, urine analysis, and genetic testing 268. Prenatal diagnosis of glutathione synthetase deficiency is possible by measuring 5-oxoproline in amniotic fluid, or by enzyme analysis in cultured amniocytes or chorionic villi samples 274, 275, 276 or a presumptive diagnosis can be made by detecting elevation of 5-oxoproline in newborn screen blood spots using tandem mass-spectrometry or massive excretion of 5-oxoproline (up to 1 g/kg per day) in the urine 277. Early diagnosis and treatment is thought to correlate with a better long term outcome 278.
Treatment for glutathione synthetase deficiency may include sodium bicarbonate to treat metabolic acidosis and taking high doses of vitamin C and vitamin E for protection against oxidative stress 268, 270, 279. Njalsson et al 280 demonstrated that early initiation of vitamin C and vitamin E could prevent the moderate disease from progressing to severe disease. In a study of 41 patients with glutathione synthetase deficiency, only 1/18 of the severely affected patients were started on vitamin therapy early in life as compared to 6/17 moderately affected patients. With this data and the fact that there is no significant difference between enzymatic activity in the moderate and severe disease, they posit that early initiation of vitamins could prevent or slow down progression of the disease 280. There were no specific comments on length of follow-up of this cohort however.
Selenium is another agent used in glutathione synthetase deficiency patients to prevent oxidative stress. Selenium is found to have strong antioxidant properties through formation of selenoproteins, which are thought to protect against reactive oxygen species 281.
Avoidance of foods and drugs known to cause hemolytic crisis in G6PD deficiency is also important as these same triggers can cause hemolytic crisis in glutathione synthetase deficiency 282. Vitamin E is also used to prevent granulocyte dysfunction, which could cause recurrent infections 283, 284. Patients with moderate and severe glutathione synthetase deficiency disease typically require treatment for metabolic acidosis. In acute crisis, IV bicarbonate is given for immediate correction, and for long term management, citrate (citric acid, potassium citrate, and sodium citrate) or trometamol (THAM) are given to maintain a normal serum bicarbonate level 265.
Glutathione synthetase deficiency prognosis depends on the type of mutation, severity of metabolic acidosis, associated bacterial infections and the quality of supportive therapy. A long-term follow up study of 28 patients with glutathione synthetase deficiency has indicated that the factors most predictive of survival and long-term outcome are early diagnosis and early supplementation with vitamins C and E 271.
Figure 4. Glutathione structure
Footnote: Structure of the tripeptide glutathione (GSH) (reduced form). Glutamic acid (Glu) is linked in a gamma (γ) peptide linkage (via its gamma-carboxyl group) to cysteine (Cys), which in turn forms an alpah (α) peptide linkage with glycine (Gly).
[Source 285 ]Figure 5. Gamma-glutamyl cycle
Footnotes: Glutathione (GSH) is synthesized by glutathione synthetase from glutamine and cysteine in a two-step process involving the enzymes glutathione synthatase and glutamate-cysteine ligase.
[Source 265 ]Figure 6. Synthesis and recycling of glutathione
Footnotes: One-carbon metabolism and glutathione (GSH) synthesis and metabolism. Folic acid is reduced to tetrahydrofolate (THF) and subsequently converted to 5-methyl THF. In a reaction catalyzed by methionine synthetase (MTR), the methyl group of 5‑methyl-THF can be transferred to homocysteine, generating methionine. Methionine is activated to form S‑adenosylmethionine (SAM), the universal methyl donor. The by-product of methylation reactions, S‑adenosylhomocysteine (SAH), is hydrolyzed to homocysteine. Homocysteine is either used to regenerate methionine or is directed to the transsulfuration pathway. Reduced glutathione (GSH) is a product of the transsulfuration pathway. Reduced glutathione (GSH) can serve as a continuous source of cysteine, which is extremely unstable, via the gamma‑glutamyl cycle. Reduced glutathione (GSH) is exported from the cell, and the enzyme gamma‑glutamyltransferase (GGT) transfers the gamma-glutamyl moiety of glutathione (GSH) to an amino acid, often cystine, producing cysteinylglycine and gamma-glutamyl amino acid. The gamma-glutamyl amino acid can be transported back into the cell and ultimately metabolized to glutamate. Cysteinylglycine is converted to cysteine and glycine by dipeptidase (DP). Cysteine is unstable extracellularly and can oxidize to cystine; both cysteine and cystine can be imported back into the cell for glutathione (GSH) production.
Abbreviations: B12 = vitamin B12; BHMT = betaine homocysteine methyltransferase; DMA = dimethylarsinic acid; InAs = inorganic acids; MMA = monomethylarsonic acid; GSSG = oxidized glutathione; GSH = reduced glutathione
[Source 286 ]Figure 7. The glutathione redox cycle
Footnote: The glutathione redox cycle demonstrating the inter-conversion of oxidized glutathione (GSSG) and reduced glutathione (GSH). Glutathione exists in two interconvertible forms, reduced glutathione (GSH) and oxidized glutathione (GSSG). Reduced glutathione (GSH) is the predominant intracellular form, which acts as a strong antioxidant and defends against toxic compounds and xenobiotics. In this process, reduced glutathione (GSH) is constantly oxidized to GSSG (oxidized glutathione) by the enzyme glutathione peroxidase. To maintain the intracellular redox balance, reduced glutathione (GSH) is replenished through the reduction of GSSG (oxidized glutathione) by glutathione reductase enzyme.
[Source 287 ]Table 9. Glutathione rich fruits and vegetables
Food | Glutathione | N-Acetylcysteine (NAC) | Cysteine |
---|---|---|---|
Asparagus | 349 ± 26 | 46 ± 1 | 122 ± 1 |
Avocado | 339 ± 10 | ND | 4 ± 1 |
Banana | ND | ND | 7 ± 0 |
Broccoli | 4 ± 1 | ND | ND |
Carrot | 4 ± 0 | ND | ND |
Cauliflower | 6 ± 1 | ND | 7 ± 1 |
Cucumber | 123 ± 38 | 6 ± 1 | 11 ± 3 |
Grapefruit | 13 ± 3 | 4 ± 0 | 15 ± 2 |
Green Beans | 230 ± 2 | ND | 67 ± 11 |
Green Pepper | 8 ± 1 | 12 ± 2 | 9 ± 1 |
Green Squash | 47 ± 11 | ND | 6 ± 1 |
Lemon | 5 ± 0 | 4 ± 0 | 6 ± 0 |
Mango | 59 ± 6 | ND | 10 ± 0 |
Orange | 5 ± 11 | ND | 41 ± 2 |
Papaya | 136 ± 12 | ND | 58 ± 5 |
Parsley | 17 ± 9 | 9 ± 1 | 8 ± 1 |
Potato | 5 ± 0 | ND | ND |
Red Pepper | 42 ± 2 | 25 ± 4 | 349 ± 18 |
Spinach | 313 ± 33 | ND | 84 ± 2 |
Strawberry | 39 ± 8 | 5 ± 1 | 59 ± 5 |
Tomato | 64 ± 10 | 3 ± 1 | 55 ± 3 |
Yellow Squash | 39 ± 8 | ND | 27 ± 6 |
Footnote: Numbers represent nM/g wet weight (mean ± SD of three samples)
ND = not detectable
[Source 170 ]Table 10. Nutrients and foods for support of glutathione levels
Nutrient and Foods | Recommended Dosage |
---|---|
Alpha lipoic-acid | 300 mg 3× day; 200–600 mg/day 288 |
Brassica vegetables | 250 g/day |
Curcumin | Doses up to 12 g/day safe; 1–2 g/day found to benefit antioxidant capacity; increased bioavailability with piperine 289 |
Fruit and vegetable juices | 300–400 mL/day |
Glutathione (Liposomal) | 500–1000 mg/day 290 |
Glutathione (Oral) | 500–1000 mg/day 291, 292 |
Glycine | 100 mg/kg/day 293 |
Green tea | 4 cups/day |
N-acetylcysteine (NAC) | 600–1200 mg/day in divided doses, but up to 6000 mg/day have been shown effective in studies 219, 179, 222, 294 |
Omega-3 fatty acids | 4000 mg/day 295 |
Salmon | 150 g twice a week 296 |
Selenium | 247 μg/day of selenium enriched yeast; 100–200 ug/day. Anything above 400 ug/day watch for toxicity 297, 294 |
Vitamin C | 500–2000 mg/day 298, 299 |
Vitamin E | 100–400 IU/day 300, 301 |
Whey Protein | 40 g/day 302 |
Quercetin
Quercetin is a polyphenolic flavonoid widely distributed in many plants and fruits including red grapes, citrus fruit, tomato, broccoli and other leafy green vegetables, and a number of berries, including raspberries and cranberries 303, 304, 305, 306, 307, 308, 309, 310, 311. In addition, quercetin is also found in certain plants of medicinal use such as St. John’s wort (Hypericum perforatum) and elderberry (Sambucus canadensis). Some foods contain large amounts of this compound, as listed in Table 1 below; for example, buckwheat contains up to 23 mg of quercetin per 100 g, and bee pollen contains almost 21 mg per 100 g.
Quercetin acutally belongs to a type of flavonols, which is a class of flavonoids that have the 3-hydroxyflavone backbone (3-hydroxy-2-phenylchromen-4-one) and present in a wide variety of herbs including licorice 312, 313. Quercetin is a licorice flavonoid and its IUPAC name is 2-(3,4-dihydroxyphenyl)-3,5,7-trihydroxy-4H-chromen-4-one with a molecular weight of 302.24 g/mol and a melting point of 316 °C, and its molecular formula is C15H10O7. Quercetin consists of five hydroxyl groups, which determine its biological activity 314, 315. Naturally, quercetin is distributed in the form of derivatives, either in glycosidic form, mainly attached to the C-3 carbon with glucose, rhamnose, and rutinose, or linked to others, and it is very rarely distributed as an aglycone 316. On the other hand, quercetin in its aglycone form is a bright lemon-yellow powder, insoluble in water but soluble in alcohol and lipids. In addition, the aglycone has been reported to have a minor effect in vivo and poor bioavailability in human plasma after oral intake of quercetin 317, 318. The most common form of quercetin is rutin (quercetin glycoside), which is usually glycosylated, formed by the addition of a glycoside group (a sugar, such as glucose, rhamnose, or rutin) instead of an -OH group (usually at the 3-position) 308.
Several studies demonstrated that quercetin had diverse bioactive effects such as antiviral activity, anti-asthmatic activity, anti-oxidant, monoamine-oxidase inhibitor, anti-tumor and anti-inflammatory activity and quercetin has been studied extensively as a chemoprevention agent in several cancer models 319, 320, 321. Quercetin has been used in medicine to decrease capillary fragility. It has also been used in dyes and as a veterinary drug 322. However, there is no reliable clinical evidence that quercetin has a protective effect on human cancer.
Quercetin itself (aglycone quercetin), as opposed to quercetin glycosides, is not a normal dietary component. Quercitin glycosides are converted to phenolic acids as they pass through the gastrointestinal tract. Quercetin has neither been confirmed scientifically as a specific therapeutic for any condition nor been approved by any regulatory agency. The U. S. Food and Drug Administration has not approved any health claims for quercetin. Nevertheless, the interest in dietary flavonoids has grown after the publication of several epidemiological studies showing an inverse correlation between dietary consumption of flavonols and flavones and reduced incidence and mortality from cardiovascular disease and cancer. In recent years, a large amount of experimental and some clinical data have accumulated regarding the effects of flavonoids on the endothelium under physiological and pathological conditions. The meta-analysis of seven prospective cohort studies concluded that the individuals in the top third of dietary flavonol intake are associated with a reduced risk of mortality from coronary heart disease as compared with those in the bottom third, after adjustment for known risk factors and other dietary components. A limited number of intervention studies with flavonoids and flavonoid containing foods and extracts has been performed in several pathological conditions.
Flavonoids are a large group of compounds (>4000) that occur naturally in fruits, vegetables, nuts, seeds, flowers and other plant matter and as such they are an integral part of the human diet 323. They all share a common three-ring structure but are subdivided into flavonols, flavons, flavanols and flavanons according to their substituents 324. Epidemiological studies indicate that diets rich in flavonoids are associated with reduced incidences of several chronic diseases including cardiovascular disease, asthma, type II diabetes and certain types of cancer 325. The cardioprotective properties of flavonoids are multi-faceted involving antioxidant, anti-hypercholesterolaemia, anti-inflammatory and inhibition of platelet aggregation effects 325. The antioxidant property of flavonoids was thought, until relatively recently, to underlie the majority of their protective cellular effects. However, it is becoming increasingly apparent that flavonoids also influence cellular function by modulating the activity of many enzymes including the inhibition of protein kinases and lipid kinases 326.
The inhibitory effect of flavonoids on protein kinase activity is primarily due to their ability to function as competitive inhibitors of the ATP-binding domain located in the active site of these enzymes 326. Protein kinases directly inhibited by flavonoids include protein kinase C (PKC), myosin light chain kinase (MLCK) and MEK1 326. MEK1 is an upstream kinase responsible for the activation of extracellular signal-regulated kinase 1/2 (ERK1/2), a member of the mitogen-activated protein kinase (MAPK) family of protein kinases 327. This family also includes p38 MAPK and JNK signalling pathways, both of which are targets for modulation by flavonoids 328. It is also notable that flavonoids such as quercetin also inhibit (via competitive inhibition of the ATP-binding site) the lipid kinase PI-3Kγ 329, which plays a prominent role in the activation of protein kinase B (PKB; also known as Akt).
The MEK/ERK1/2 and the PI-3K/PKB pathways are important signalling pathways which mediate cell survival and cardioprotection against ischaemia/reperfusion injury 330. In contrast, p38 MAPK and JNK cascades are typically associated with inflammation and cell death; however, there is also evidence that they mediate cardioprotection 330. Hence, the cardioprotective effects of flavonoids may relate to their ability to modulate MAPK and PI-3K/PKB signalling cascades. Indeed, recent studies have shown that grape seed proanthocyanidin extract protects cardiomyocytes from ischaemia/reperfusion injury via PKB activation 331. Similarly, quercetin (the most abundant dietary flavonoid) protected H9c2 cardiomyoblasts from H2O2-induced cell death via enhancement of ERK1/2 and PI-3K/PKB signalling 332. In contrast, isorhamnetin (a flavonol isolated from sea buckthorn) prevented H2O2-induced activation of apoptosis in H9c2 cells through ERK1/2 inactivation 333.
Figure 8. Quercetin chemical structure
Footnotes: Quercetin chemical structure. The figure shows the chemical characteristics that give Quercetin its antioxidant capacity, the most important of which is the catechol or dehydroxylated B-ring structure (yellow). Other important characteristics are the presence of unsaturation in the C-ring (red) and the presence of a 4-oxo in the C-ring (green); the oxygen in position 4 and the hydroxyl in position 5 allow it the capacity to chelate ions such as Cu++ and Fe++ (blue). On the other hand, in total, quercetin has 5 functional hydroxyl groups with the potential to be conjugated and that differ in their chemical reactivity following the order of reactivity 3 > 7 > 3′ > 4′ >> 5. The phenolic hydroxyl groups of quercetin act as donors of electrons, and these are responsible for the capture activity of free radicals, in particular the catechol structure with 2 hydroxyl groups in the neighboring positions, which is notably superior to other arrangements in electron donation.
[Source 308 ]Table 11. Foods containing quercetin
Food Source | Quercetin Content (mg/100 g) |
---|---|
FRUITS | |
Apple with skin (Malus domestica) | 4.42 |
Acerola (Malpighia emarginata) | 4.74 |
Arctic bramble (Rubus arcticus) | 9.1 |
Blueberry (Vaccinium caesariense) | 7.67 |
Cranberry (Vaccinium macrocarpon) | 14.84 |
Elderberry (Sambucus spp.) | 26.77 |
Fig (Ficus carica) | 5.47 |
Plum (Prunus domestica) | 12.45 |
Sea buckthorn (Hippophae rhamnoides) | 7.4 |
Wolfberry (Lycium barbarum) | 13.6 |
Common juniper (Juniperus communis) | 46.61 |
Prickly pear (Opuntia spp.) | 4.86 |
VEGETABLES | |
Sowthistle (Sonchus oleraceus) | 16 |
Arugula (Eruca sativa) | 7.92 |
Sparrow grass (Asparagus officinalis) | 13.98 |
Swiss chard (Beta vulgaris) | 7.5 |
Green chicory (Cichorium intybus) | 6.49 |
Coriander (Coriandrum sativum) | 52.9 |
Golden poppy (Eschscholzia californica) | 26.3 |
Drumstick tree (Moringa oleifera) | 16.65 |
Fennel (Foeniculum vulgare) | 48.8 |
Leaf cabbage (Brassica oleracea) | 7.71 |
Red lettuce (Lactuca sativa) | 7.61 |
Mustard greens (Brassica juncea) | 8.8 |
Okra (Abelmoschus esculentus) | 20.97 |
Onions (Allium cepa) | 20.3 |
Perennial wall-rocket (Diplotaxis tenuifolia) | 66.19 |
New Mexico chile (Capsicum annuum) | 15 |
Sweet potato (Ipomoea batatas) | 16.94 |
SPICES AND HERBS | |
Caper bush (Capparis spinosa) | 180.77 |
Dill (Anethum graveolens) | 55.15 |
Oregano (Origanum vulgare) | 7.3 |
Tarragon (Artemisia dracunculus) | 11 |
Turmeric (Curcuma longa) | 4.92 |
Buckwheat (Fagopyrum esculentum) | 15.38 |
Alpha lipoic acid
Alpha-lipoic acid also called lipoic acid, thioctic acid or 1,2-dithiolane-3-pentanoic acid (pentanoic acid), is a naturally occurring short chain fatty acid which contains a sulfur (thiol) bond, synthesized in small amounts by plants and animals including humans, with antioxidant and potential chemopreventive activities 334, 335, 336, 337. Alpha lipoic acid is a common ingredient in multivitamin tablets and in dietary supplements and is even included in many pet foods 338, 339, 340. Food sources of alpha-lipoic acid include red meat, beets, carrots, potatoes, spinach, and broccoli.
Chemically, alpha lipoic acid is a short-chain fatty acid with a disulfide group in its dithiolane ring and a chiral carbon resulting in R and S enantiomers. Alpha-lipoic acid is available naturally in foods as the R-alpha-lipoic acid and is bound to lysine in proteins 341, 342. Alpha lipoic acid in supplements is available as a racemic mixture of R-alpha lipoic acid (the R form) and S-alpha lipoic acid (the S form) also referred to as d,l-lipoic acid and is not bonded to protein 342. R-alpha-lipoic acid (the R form) is the biologically active form that is endogenously produced by your body, while the S form (S-alpha-lipoic acid) is produced from chemical manufacture and is not biologically active 343. Only R-alpha-lipoic acid is bound to a specific lysine residue in at least one of the proteins in each multienzyme complex, thus making R-alpha-lipoic acid essential as a cofactor (a compound that is essential for the activity of an enzyme) for several mitochondrial multienzyme complexes that catalyze critical reactions related to the catabolism (breakdown) of amino acids and the production of energy 344, 345, 346. Alpha lipoic acid, when exogenously administered, is readily absorbed from the gut and has been clinically used in Europe for the treatment of diabetic neuropathy (nerve damage caused by diabetes) 347.
Though in body synthesis appears to supply all the necessary alpha lipoic acid needed for its role in intermediary metabolism, alpha lipoic acid can also be absorbed intact from dietary sources and can be found in almost all foods, but slightly more so in organ meats (kidney, heart, liver), spinach, broccoli, peas, brussel sprouts, and rice bran 348. While the direct roles of alpha lipoic acid as a cofactor (a compound that is essential for the activity of an enzyme) are well understood, less is known about the precise metabolic functions of orally supplied alpha lipoic acid. Alpha-lipoic acid can also be made in the laboratory. Alpha-lipoic acid is biosynthesized by cleavage of linoleic acid and is a coenzyme of oxoglutarate dehydrogenase (ketoglutarate dehydrogenase complex).
An upper limit for alpha lipoic acid consumption in humans has not been conclusively established 338. However, a dosage of 20–50 mg daily is recommended for general antioxidant support 349. The use of alpha lipoic acid is currently approved for the treatment of diabetic neuropathy in Germany and is available by prescription 350, 351. It is important to note that many of the studies that examined the efficacy of alpha lipoic acid in the treatment of diabetic neuropathy have been primarily conducted by one German research group and funded by the manufacturer of alpha lipoic acid in Germany 352. The recommended dosage of alpha lipoic acid for the treatment of diabetes is 300–600 mg daily. Alpha lipoic acid is available as an over the counter dietary supplement in the United States 353.
The amounts of alpha lipoic acid available in dietary supplements vary between 50–600 mg and could be as much as 1000 times higher than the amounts consumed from food intake 338. Taking alpha lipoic acid with food is known to decrease its bioavailability; hence it is recommended that alpha lipoic acid be taken half an hour to an hour prior to a meal 341, 354. Alpha lipoic acid in food does not cause detectable increase of free alpha lipoic acid in human plasma or in cells 355, 341. However, high oral doses (≥50 mg) of free alpha lipoic acid increase levels of free lipoic acid in human plasma and cells 341, 356. Human studies have shown that approximately 30–40% of an orally administered racemic mixture of R- and S-alph lipoic acid is absorbed 341, 356. All published human clinical trials involving alpha lipoic acid supplementation have used racemic mixture of R-alpha lipoic acid (the R form) and S-alpha lipoic acid (the S form) 357, 358, 359, 360, 361, 362, 363, 354, 364, 356. Following oral administration with racemic alpha lipoic acid, the peak concentrations of R-alpha lipoic acid in plasma were found to be 40–50% more than those of S-alpha lipoic acid, indicating that R-alpha lipoic acid may be more efficiently absorbed than S-alpha lipoic acid, but both isomers were metabolized and eliminated rapidly 341, 365, 366. A study involving 19 healthy adults suggests that the bioavailability of the R and S-isoforms of alpha lipoic acid may vary with age and gender 367. Concentrations of alpha-lipoic acid in plasma typically peak within an hour and then decline rapidly 341, 356, 366. Alpha lipoic acid is swiftly reduced to dihydrolipoic acid (DHLA) in cells, and in vitro studies show that dihydrolipoic acid (DHLA) is then quickly exported from cells 367.
Two forms of alpha-lipoic acid are oxidized lipoic acid and reduced dihydrolipoic acid (DHLA) 368. Both are capable of scavenging a variety of reactive oxygen species (ROS). At the cellular level, alpha lipoic acid is reduced to dihydrolipoic acid (DHLA), which is a potent antioxidant that can neutralize free radicals. Dihydrolipoic acid (DHLA) has a number of cellular actions including free radical scavenging and modulating oxidative stress and inflammatory pathways 369, 370, 343, 371, 372, 373. Furthermore, alpha-lipoic acid simultaneously regenerates other antioxidant factors, such as vitamins C and E, increasing glutathione synthesis 374. Alpha-lipoic acid is generally involved in keto acid oxidative decarboxylation processes and is a growth factor for some organisms 375.
Alpha-lipoic acid acts as a free radical scavenger and assists in repairing oxidative damage and regenerates endogenous antioxidants, including vitamins C and E and glutathione. Alpha lipoic acid also promotes glutathione synthesis. In addition, alpha-lipoic acid exerts metal chelating capacities and functions as a cofactor for energy production in the mitochondria in various mitochondrial enzyme complexes involved in the decarboxylation of alpha-keto acids and thus serves a critical role in mitochondrial energy metabolism 376.
Alpha lipoic acid is marketed in the US as an over-the-counter nutritional antioxidant supplement, alone or in combination with other antioxidants. In medicine, alpha lipoic acid has been shown to reduce symptoms of diabetic polyneuropathy, and several clinical trials established some efficacy and an excellent safety profile in this patient population 377. Meta-analyses of randomized controlled trials suggest that infusion of 300 to 600 mg/day of alpha lipoic acid for two to four weeks significantly reduced the symptoms of diabetic neuropathy to a clinically meaningful degree 378, 379. Regarding the efficacy of oral alpha lipoic acid supplementation, an initial short-term study in 24 patients with type 2 diabetes mellitus found that the symptoms of peripheral neuropathy improved in those who took 600 mg of alpha lipoic acid three times a day for three weeks compared to those who took a placebo 380. A larger clinical trial randomly assigned more than 500 patients with type 2 diabetes and symptomatic peripheral neuropathy to one of the following treatments: (i) 600 mg/day of intravenous alpha lipoic acid for three weeks followed by 1,800 mg/day of oral lipoic acid for six months, (ii) 600 mg/day of intravenous alpha lipoic acid for three weeks followed by oral placebo for six months, or (iii) intravenous placebo for three weeks followed by oral placebo for six months 381. Evidence of improvements in sensory and motor deficits — assessed by physicians — could be observed after three weeks of intravenous alpha lipoic acid therapy, yet not at the end of six months of oral alpha lipoic acid therapy. However, another randomized, double-blind, placebo-controlled trial in 181 patients with diabetic neuropathy found that oral supplementation with either 600 mg/day, 1,200 mg/day, or 1,800 mg/day of alpha lipoic acid for five weeks significantly improved neuropathic symptoms 382. In this study, the 600 mg/day dose was as effective as the higher doses. Finally, a four-year, multicenter, clinical trial in 421 diabetic patients with distal symmetric sensorimotor polyneuropathy found no difference between oral administration of 600 mg/day of alpha lipoic acid and placebo on the primary endpoint, a composite score that assessed neuropathic impairment of the lower limbs and nerve conduction 383. Yet, measures of specific neuropathic impairments (secondary outcomes) improved with alpha lipoic acid supplementation 383. A post-hoc analysis suggested that oral alpha lipoic acid supplementation may reduce neuropathic symptoms particularly in subjects with a high burden of cardiovascular disease, diabetes, and neuropathy yet with normal body mass index (BMI) and blood pressure 384. Overall, the available research suggests that treatment with intravenous or oral alpha lipoic acid may help reduce symptoms of diabetic peripheral neuropathy.
Alpha-lipoic acid is a type of antioxidant and chemoprotective agent. Alpha-lipoic acid is an antioxidant — a substance the body can use to prevent or manage a tissue-damaging process called oxidative stress. Oxidative stress is a part of the diabetic neuropathy disease process. Alpha-lipoic acid also has been shown to reduce blood sugar levels. Alpha lipoic acid is being studied for its ability to protect normal cells from the side effects of chemotherapy and prevent peripheral neuropathy (numbness, tingling, burning, and weakness in the hands or feet) 385. A few small clinical trials have tested the treatment effect of alpha-lipoic acid given either as a supplement or intravenously. People with diabetic neuropathy had reduced pain, improvements in nerve function tests and improvements in other clinical measures of diabetic neuropathy (Han T, Bai J, Liu W, et al. A systematic review and meta-analysis of α-lipoic acid in the treatment of diabetic peripheral neuropathy. European Journal of Endocrinology. 2012;167(4):465-471.)). But long-term studies are needed.
Alpha-lipoic acid is being studied for its effect on complications of diabetes, including diabetic macular edema (an eye condition that can cause vision loss) and diabetic neuropathy (nerve damage caused by diabetes).
- In a 2011 study of 235 people with type 2 diabetes, 2 years of supplementation with alpha-lipoic acid did not help to prevent macular edema 386.
- A 2016 assessment of treatments for symptoms of diabetic neuropathy that included 2 studies of oral alpha-lipoic acid, with a total of 205 participants, indicated that alpha-lipoic acid may be helpful 387.
Currently, alpha lipoic acid is available in tablets and capsules of 50 to 600 mg and the recommended dosage has ranged from 100 to 600 mg once or twice daily. Alpha lipoic acid is usually well tolerated when taken as recommended but side effects at higher doses can include abdominal discomfort, heartburn, constipation or diarrhea, nausea, dizziness, and headache. Rare, potentially severe adverse effects reported after single large overdoses include confusion, stupor, seizures, lactic acidosis, rhabdomyolysis, coma, and multiorgan failure that can be fatal. Don’t use alpha-lipoic acid if you’re a heavy user of alcohol.
Alpha lipoic acid deficiency has been described in rare cases of inherited mutations in the lipoic acid biosynthetic pathway. Mutations identified in patients with defective alpha lipoic acid metabolism affect genes involved in the synthesis of iron-sulfur clusters and genes coding for lipoic acid synthetase (LIAS), lipoyl transferase 1 (LIPT1), and dihydrolipoamide dehydrogenase (E3 component of alpha-ketoacid dehydrogenase complexes; DLD) 388, 389, 390.
Figure 9. Alpha lipoic acid R and S enantiomers
Figure 10. Alpha lipoic acid functions
[Source 391 ]N-acetyl cysteine
N-acetylcysteine (NAC) also known as N-acetyl-cysteine or acetylcysteine, is a natural amino acid with powerful antioxidant with anti-inflammatory activity and a scavenger of reactive oxygen species (ROS) 392, 393. N-acetylcysteine (NAC) is a modified amino acid that is used as an antidote to treat acetaminophen (paracetamol) overdose to prevent acute liver injury and is almost 100% effective if given within 8 hours post-ingestion acetaminophen (paracetamol) 394, 395, 396, 397. People commonly use N-acetylcysteine (NAC) for cough in idiopathic pulmonary fibrosis and as a mucolytic agent in cystic fibrosis, chronic obstructive pulmonary disease (COPD), pneumonia, bronchitis, tracheobronchitis, tracheostomy patients, postoperative pulmonary complications, posttraumatic chest conditions and before diagnostic bronchoscopy to help with mucous plugging 394, 398, 399, 400, 401. N-acetylcysteine (NAC) is also used for flu, dry eye (keratoconjunctivitis sicca), contrast-induced nephropathy, acute liver failure, Alzheimer disease and many other conditions, but there is no good scientific evidence to support many of these uses 402, 403, 404, 405. There is also no good evidence to support using N-acetylcysteine (NAC) for COVID-19 398. Although many dietary supplement products contain N-acetylcysteine (NAC), the United States Food and Drug Administration (FDA) states that it’s illegal for dietary supplements to contain N-acetyl cysteine since it’s technically an approved drug. Prescription N-acetyl cysteine products are available under the guidance of a healthcare provider. N-acetylcysteine is found mostly in plants of the Allium species, especially in the onion (Allium cepa, 45 mg NAC/kg) 406, 407
N-acetylcysteine (NAC) has also been used as an antioxidant precursor to glutathione (GSH), a tripeptide made up of cysteine, glycine, and glutamic acid that is found in relatively high concentrations inside many cells 172. Therefore, the human body requires all three amino acids (i.e, cysteine, glycine, and glutamic acid) and adequate enzymatic function to make sufficient quantities of glutathione 176. The availability of the amino acid cysteine is known to be rate-limiting for the synthesis of glutathione, and it is well documented, both clinically and in animal studies, that cysteine supplementation most practically achieved by administration of N-acetylcysteine (NAC) can boost glutathione synthesis and levels 408, 401, yet a review of research data indicates the use of N-acetylcysteine (NAC) to boost glutathione synthesis and levels may be inconclusive or equivocal 170. Cysteine is a sulfur amino acid, which might imply that consuming cysteine-rich foods such as meat, fish, grains, dairy, soybean, and egg products, may also support glutathione synthesis 177, 409. A systematic review 179 that included twelve clinical trials utilizing N-acetylcysteine (NAC) supplementation with a specific focus on cognitive markers indicated that there may be some benefit to using N-acetylcysteine in certain populations; however, the studies were too variable in design and outcome to make any definitive conclusion 170.
Although N-acetylcysteine (NAC) is promising as a supplement to both boost glutathione levels and has the potential to reduce some of the issues related to oxidative stress 213, 214, the research up till now is inconclusive 215, 216, 217, 218. Furthermore, it is unclear if the effects of N-acetylcysteine (NAC) on oxidative stress are due to its antioxidant properties or due to increased glutathione synthesis 170. Moreover, it has been suggested that N-acetylcysteine (NAC) may work synergistically with other supplemental nutrients. For example, it has been postulated that glycine may be as important as cysteine when it comes to glutathione production, especially when concurrently supplemented with N-acetylcysteine 175. While further studies are required, it may be the better approach to supplement with both cysteine and glycine to see a boost in glutathione, especially among those who may not have adequate quantities of the amino acids or require higher levels of glutathione.
For example, in a small study 293 with eight healthy elderly adults and a control group of eight younger subjects, after measuring baseline glutathione synthesis in both groups, the older subjects were orally administered 0.81 mmol N-acetylcysteine per kg per day (around 132 mg/kg/day) and 1.33 mmol glycine/kg/day (roughly 100 mg/kg/day) for 14 days. Initially, the older subjects had 55.2% less glycine and 24.4% less cysteine in their red blood cells 293. They also had a 46.2% lower glutathione level than the controls 293. However, after N-acetylcysteine (NAC) supplementation, the glycine levels increased by 117.6% and the cysteine by 55.1%. Furthermore, they had a 94.6% higher glutathione concentration in their red blood cells, which also led to no statistical difference between the young controls and the elderly subjects in their glutathione levels 293. In addition, they experienced lower plasma oxidative stress and F2-isoprostanes 293. The researchers speculated that the typical reduction of glutathione in the elderly was due to a lower supply of glycine and cysteine, the precursors to glutathione synthesis, and that upon N-acetylcysteine (NAC) supplementation, they had the ability to stimulate synthesis and restore levels. Although impressive, it is important to recognize that this was a small study. It is worthwhile to note that there was also no translation to clinical benefit, as these subjects were healthy 170.
In another study 219 on five people with mild to moderate Parkinson’s disease and three controls, a high dose of N-acetylcysteine (3000 mg taken orally twice daily) for a period of four weeks led to an increase of cysteine levels and antioxidant measures, with no commensurate improvement in oxidative stress measurements (4-hydroxynonenal and malondialdehyde) nor did it increase the level of glutathione in the brain 219. Additionally, some of the participants experienced a worsening of Parkinson’s symptoms that were alleviated upon stopping the N-acetylcysteine supplementation 170.
Another study 220 looking at those with neurodegenerative disorders found that a single intravenous (IV) dose of N-acetylcysteine led to an increase of the blood reduced glutathione (GSH)/oxidized glutathione (GSSG) ratio and levels of glutathione in the brain 220. Those who had the greatest percent change in reduced glutathione (GSH)/oxidized glutathione (GSSG) ratio also had a greater percent change in their levels of glutathione in their brain. While the study was too small and of too short duration to make any conclusions about the role of N-acetylcysteine in these conditions, what is notable is that through intravenous (IV) administration of N-acetylcysteine, brain levels could be altered 170.
In a 12-week clinical trial with children (n = 31) with autism spectrum disorder administered 60 mg/kg/day in three doses (maximum dose of 4200 mg/day) found that although there was no significant impact on the social impairment associated with autism, there was a significant impact on boosting the glutathione levels in the children 221. Similar to other studies, more needs to be explored as to how N-acetylcysteine influences glutathione levels, factors that modify individual response to supplementation and how symptomatology interrelates. Glutathione-S-transferase (GST) polymorphisms may play a role in the efficacy of N-acetylcysteine 170. In one study 222 investigating the impact of N-acetylcysteine on noise-induced hearing loss in men (n = 53) taking 1200 mg per day for 14 days led to a significant reduction of noise-induced temporary threshold shift, or the amount of hearing loss after a period of heightened noise exposure in a work setting relative to baseline, or pre-shift levels. When the participants were grouped according to their glutathione-S-transferase (GST) genotypes, the researchers found that only those with the null genotypes experienced a significant effect from taking N-acetylcysteine 170.
N-acetylcysteine can cause side effects such as dry mouth, nausea, vomiting, and diarrhea. One of the properties of N-acetylcysteine is that it has an unpleasant smell and taste that some people find hard to tolerate. Vomiting after intravenous use has been reported in about 11% at doses of 150 mg/kg and one anaphylactic reaction has been reported 410. N-acetylcysteine has anticoagulant and platelet inhibiting properties and the use in patients with bleeding disorders or blood thinners may be relatively counterindicated 411. The use of N-acetylcysteine with patients on nitroglycerine should be cautioned, since it may cause hypotension 412. Other more rare side effects may include inflammation of the mucous membrane of the mouth (stomatitis), drowsiness, runny nose (rhinorrhea), and coughing up blood (hemoptysis) 413.
Table 12. N-acetylcysteine (NAC) potential mechanisms of action
1 | Action on glutathione | N-acetylcysteine restores glutathione (cysteine is rate limiting) as seen in cell and animal studies and clinically in acetaminophen overdose 414. |
2 | Stabilizes proteins/DNA | Protects proteins by crosslinking cysteine disulfide molecules 415. Various mechanisms of DNA repair/protection as seen in animal studies and human cell studies 416. |
3 | Scavenges free radicals | Scavenging property via the redox potential of thiols as demonstrated in cell culture 417. |
4 | Anti-inflammatory property | Reduces proinflammatory cytokines as seen in animal studies 418. |
5 | Antioxidant property | Reduces oxidative damage as seen in cell cultures 419. |
6 | Mucolytic property | Splits disulfide bonds in mucoproteins lowering viscosity demonstrated in purified mucus gels and tracheal explant systems and in vitro (in a pig tracheal pouch) models 420. |
7 | Mitochondrial resilience | Neurogenesis-inducing ability 421 reduces apoptosis of mitochondria as demonstrated in human dental pulp cells 422. |
8 | Metal chelation | Thiol groups provide binding sites for metals in animal studies 423. |
9 | Glutamate/dopamine homeostasis | Modulates glutamate and dopamine extensive studies in humans 403. |
10 | Antiviral properties | Immune modulation, anti-NF-KB properties, and other unexplored mechanisms observed in vitro and in vivo 424. |
11 | Vascular endothelial growth factor | Inhibition of vascular permeability as seen in human keratinocytes 425. |
12 | Adenosine triphosphate (ATP) and nitric oxide (NO) production | Increased ATP production in some cells like fibroblasts in vitro 426. Increased nitric oxide production as demonstrated in human studies 427. |
Figure 11. N-acetylcysteine structure
Astaxanthin
Astaxanthin (3,3′-dihydroxy-β, β′-carotene-4,4′-dione) is a lipid-soluble red-orange pigment belonging to the xanthophyll carotenoid family created by micro-algae (Haematococcus pluvialis, Chlorella zofingiensis, Chlorococcum, and Phaffia rhodozyma) in times of stress 429, 430, 431, 432, 433, 434. Haematococcus pluvialis is a green microalga, which accumulates high astaxanthin content under stress conditions such as high salinity, nitrogen deficiency, high temperature and light 435. When the Haematococcus pluvialis algae are exposed to change in their environment such as direct sunlight, starved of food or being eaten, it produces astaxanthin to protect itself. As a potent antioxidant, astaxanthin protects the algae from free radicals and oxidative stress, keeping it alive and well when stressed. Astaxanthin accumulates up to 3.8% on the dry weight basis in Haematococcus pluvialis. Astaxanthin produced from haematococcus pluvialis is a main source for human consumption 436. Astaxanthin carotenoid is what gives many sea creatures such as salmon, trout, krill, crayfish, shrimp and crab their red color 437, 438, 439. Carotenoids contribute to the yellow, orange, and red colors of the skin, shell, or exoskeleton of aquatic animals. Indeed, carotenoids are the most widespread pigments found in nature, as they occur in bacteria, yeasts, mold, all green plants, and many animals, and therefore various functions have been attributed to them. From anthropocentric consideration, the most significant aspect of carotenoids is the color they impart to our food and environment.
Astaxanthin is closely related to beta-carotene, lutein, and zeaxanthin, sharing with them many of the general metabolic and physiological functions attributed to carotenoids. Mammals, including humans, lack the ability to synthesize astaxanthin or to convert dietary astaxanthin into vitamin A.
Astaxanthin is a carotenoid widely used in salmon, trout, red seabream, shrimp, lobster, fish eggs and crustacean aquaculture to provide the pink color characteristic of that species 437, 438, 440, 441. Astaxanthin is also found in some birds, such as flamingoes, quails, and other species. Astaxanthin, similar to other carotenoids, cannot be synthesized by animals and must be provided in the diet. Salmon farming increased substantially in the 1980s, which created a large market for astaxanthin, the principal pigment of salmon. Since animals lack the ability to synthesize carotenoids, the pigments must be supplemented to feeds, usually at considerable expense to the farmer (10 to 15% of total feed costs). This application has been well documented for over two decades and is currently the major market driver for the astaxanthin pigment. Currently, chemically synthesized astaxanthin and canthaxanthin (β,β-carotene4,4′-dione) are added to salmon feeds as pigmenters, but there is considerable interest within the aquaculture industry in using natural sources of astaxanthin. Additionally, astaxanthin also plays a key role in animals as an intermediary in reproductive processes, the carotenoids are associated with reproductive organs and hence the hatching success and survival of alevins (newly spawned salmons or trouts still carrying the yolk).
Synthetic astaxanthin dominates the world market but recent interest in natural sources of the pigment has increased substantially. Common sources of natural astaxanthin are the green algae Haematococcus pluvialis, the red yeast Phaffia rhodozyma, as well as crustacean byproducts. Each natural pigment source has its limitations and they currently cannot compete economically with the synthetic additive 442. The algae Haematococcus pluvialis has a high concentration of astaxanthin (0.2 to 2%), but industrial application is limited by the lengthy autotrophic cultivation in open freshwater ponds and the requirement for disruption of the cell wall to liberate the carotenoid 442. The red yeast Phaffia rhodozyma has desirable properties as a biological source of pigment, including rapid heterotrophic metabolism and production of high cell densities in fermentors, but its content of astaxanthin in wild strains is only 200 to 300 μg/g yeast (0.02 to 0.03%) 442. Mutants have been isolated that produce >3000 μg total carotenoid per gram of yeast (>0.30%) in shake flasks after 5 d growth, and measurement of carotenoid fluorescence in individual cells indicates that levels of 10,000 to 15,000 μg/g can be obtained 442. High producers, however, are often unstable and further strain development is required.
Astaxanthin possesses an unusual antioxidant activity which has caused a surge in the nutraceutical market for the encapsulated product. Also, health benefits such as cardiovascular disease prevention, immune system boosting, bioactivity against Helycobacter pylori, and cataract prevention, have been associated with astaxanthin consumption 443. Research on the health benefits of astaxanthin is very recent and has mostly been performed in test tube study or at the pre-clinical level with humans.
Figure 12. Astaxanthin chemical structure
Figure 13. Astaxanthin isomers
Footnotes: Astaxanthin optical isomers and major geometric isomers. Most natural astaxanthin exists as a mixture of geometrical isomers, including small amounts of 9-cis, 13-cis, and 15-cis forms, along with the all-trans form.
Abbreviation: AST = astaxanthin
[Source 434 ]Coenzyme Q10
Coenzyme Q10 (CoQ10) also called ubiquinone, ubidecarenone or vitamin Q10 is a benzoquinone compound (a fat-soluble vitamin-like molecule) that is naturally present in the human body in most body tissues, with the highest levels in the heart, liver, kidneys, and pancreas 444. The lowest amounts are found in the lungs 445. CoQ10 decreases in the body as people get older due to increased requirements, decreased production or insufficient intake of the chemical precursors needed for coenzyme Q10 synthesis 445, 446. Your body uses Coenzyme Q10 to make energy needed for the cells to grow and stay healthy. Your body also uses Coenzyme Q10 as an antioxidant 447, 448, 449, 450, 451. An antioxidant is a substance that protects cells from free radicals, which are highly reactive chemicals, often containing oxygen atoms, capable of damaging important cellular components such as DNA and lipids. In addition, the plasma level of coenzyme Q10 has been used in studies as a measure of oxidative stress 452, 453. Conditions such as fibromyalgia, diabetes, cancer, heart failure, and neurodegenerative, mitochondrial, and muscular diseases are associated with decreased circulating levels of Coenzyme Q10 (CoQ10) 454, 455, 456, 457, 458, 459. Several studies have been conducted to determine whether increasing systemic CoQ10 levels would enhance bodily function 460, 461, 462.
Coenzyme Q10 is a lipid-soluble benzoquinone that has 10 isoprenyl units in its side chain and is a key component of the mitochondrial respiratory chain for adenosine triphosphate (ATP) synthesis by acting as an electron carrier in mitochondria and as a co-enzyme for mitochondrial enzymes 463, 464. Studies has indicated that coenzyme Q10 is an intracellular antioxidant can protects membrane phospholipids, mitochondrial membrane protein, and low density lipoprotein-cholesterol (LDL-C) from free radical-induced oxidative damage 465. In vitro or in vivo studies have demonstrated that coenzyme Q10 not only plays an antioxidant, but also has anti-inflammation effects 466 by modulating the expression of cyclooxygenase-2 and nuclear factor-κB (NF-κB) in the liver tissue of rats with hepatocellular carcinoma 467.
The “Q” and the “10” in Coenzyme Q10 refer to the quinone chemical group and the 10 isoprenyl subunits that are part of this compound’s structure 468. The term “coenzyme” denotes it as an organic (meaning it contains carbon atoms) nonprotein molecule necessary for the proper functioning of its protein partner (an enzyme or an enzyme complex) 468.
Coenzyme Q10 is used by cells of your body in a process known variously as 468:
- Aerobic respiration: A chemical process in which oxygen is used to make energy from carbohydrates (sugars). Also called aerobic metabolism, cell respiration, and oxidative metabolism.
- Aerobic metabolism: A chemical process in which oxygen is used to make energy from carbohydrates (sugars). Also called aerobic respiration, cell respiration, and oxidative metabolism.
- Oxidative metabolism: A chemical process in which oxygen is used to make energy from carbohydrates (sugars). Also called aerobic metabolism, aerobic respiration, and cell respiration
- Cell respiration: A chemical process in which oxygen is used to make energy from carbohydrates (sugars). Also called aerobic metabolism, aerobic respiration, and oxidative metabolism..
Coenzyme Q10 is crucial for efficiently transferring electrons within the mitochondrial oxidative respiratory chain and producing adenosine triphosphate (ATP), which is a packet of energy for cell growth and maintenance 469, 447, 448, 470, 471. CoQ10 can potentially increase the production of vital antioxidants, such as superoxide dismutase, an enzyme that effectively reduces vascular oxidative stress in individuals with high blood pressure 472. In addition, CoQ10 lowers lipid peroxidation levels by diminishing pro-oxidative compounds 473. Furthermore, CoQ10 can improve blood flow and safeguard blood vessels by preserving nitric oxide.
Figure 14. Coenzyme Q10
A coenzyme Q10 deficiency occurs with age; however, certain drugs can cause depletion of coenzyme Q10 levels, particularly hydroxy-methylglutaryl-coenzyme A (HMG-CoA) reductase inhibitors, or statins 474. Statins are prescribed to reduce cholesterol levels and work by inhibiting HMG-CoA reductase and the mevalonate metabolic pathway 475. Mevalonate is used to synthesize cholesterol as well as coenzyme Q10 476, therefore, when statin drugs lower cholesterol levels they simultaneously lower coenzyme Q10 levels. Statins are known to block coenzyme Q10 biosynthesis and reduce serum concentrations of coenzyme Q10 by up to 40% 477. Furthermore, statin use is often associated with a variety of muscle-related symptoms or myopathies. Research has suggested that coenzyme Q10 supplementation may decrease muscle pain associated with statin treatment 478.
Coenzyme Q10 is sold in the United States as a dietary supplement. Supplementary oral administration of coenzyme Q10 has been shown to increase coenzyme Q10 levels in plasma, platelets, and white blood cells 479. Because CoQ10 has important functions in the body and because people with some diseases have reduced levels of this substance, researchers have been interested in finding out whether CoQ10 supplements might have health benefits. Studies suggest that CoQ10 deficiency may be associated with a multitude of diseases as diverse as coronary artery disease and congestive heart failure, Parkinson’s disease, diabetes, and breast cancer, as well as the risk factor, hypertension 479. It has been suggested that Coenzyme Q10 has the potential to lower blood pressure without significant adverse events in hypertensive patients 480.
There are also a number of ways that Coenzyme Q10 could act favorably to reduce blood pressure. Coenzyme Q10 could act directly on vascular endothelium and decrease total peripheral resistance by acting as an antagonist of vascular superoxide, by either scavenging it, or suppressing its synthesis 481. Further to this, a recent meta-analysis has associated CoQ10 supplementation with a significant improvement in arterial endothelial function in patients with and without cardiovascular disease 482. Coenzyme Q10’s antioxidant properties may also result in the quenching of free radicals that cause inactivation of endothelium-derived relaxing factor or fibrosis of arteriolar smooth muscle, or both 483. In addition, CoQ10 has been found to decrease blood viscosity and improve blood flow to cardiac muscle in patients with ischemic heart disease; therefore it may reduce blood pressure 484.
Dietary supplementation with coenzyme Q10 results in increased levels of ubiquinol-10 (the reduced form of coenzyme Q10) within circulating lipoproteins. In its reduced form the coenzyme Q10 molecule acts as a powerful intracellular antioxidant due to its ability to hold electrons rather loosely, and will quite easily give up one or both electrons. The antioxidant and free radical scavenger effects of coenzyme Q10 can therefore help to prevent lipid peroxidation and thus the progression of atherosclerosis 477. Furthermore, coenzyme Q10 has also been found to modulate the amount of ß-integrin levels on the surface of blood monocytes, strongly suggesting that the anti-atherogenic effects of coenzyme Q10 are mediated by other mechanisms beside its antioxidant properties 485.
According to 2022 American College of Cardiology/American Heart Association/The Heart Failure Society of America guidelines for the Management of Heart Failure recommended supplementation with coenzyme Q10 effectively reduced vascular mortality, all-cause mortality, and hospital stays for heart failure at 2 years 486. However, long-term supplementation is needed 486.
A recently published systematic review showed that supplementation with coenzyme Q10, in addition to standard therapy in patients with moderate-to-severe heart failure, is associated with symptom reduction and reduction of major adverse cardiovascular events 461, 487. CoQ10 may improve functional capacity, endothelial function, and left ventricle contractility in congestive heart failure patients 461, 488.
Coenzyme Q10 supplementation shows promising results in improving endothelial function in several subsets of patients. CoQ10 can improve endothelial function in patients with ischemic left ventricular systolic dysfunction and heart failure 489, 490. Likewise, compared with placebo, CoQ10 improves endothelial function in the peripheral circulation of patients with type 2 diabetes and hyperlipidemia 491. However, routine use of CoQ10 in patients with coronary artery disease apart from congestive heart failure is still inconclusive 489, 492.
There is also evidence that combined with selenium, CoQ10 supplementation in healthy older patients and older patients with diabetes, hypertension, and ischemic heart disease may decrease cardiovascular mortality risk 493. Data are conflicting on whether CoQ10 may play a role in treating high blood pressure 494. Coenzyme Q10 shows the potential to decrease pain, fatigue, and morning tiredness compared to a placebo in patients with fibromyalgia 495, 496. Some data suggest that supplementation with moderate-to-high dose coenzyme Q10 may influence bicycle exercise aerobic capacity in patients with mitochondrial disorders 497.
Supplementation with Coenzyme Q10 300 mg daily for 24 weeks in men with Peyronie disease may decrease penile plaque size, reduce penile curvature, and improve erectile function 498. Statin drugs inhibit the production of an intermediate in the mevalonate pathway—a biochemical route leading to CoQ10 synthesis 499. Therefore, many researchers theorize that statin drugs may contribute to coenzyme Q10 depletion in the body. Given that muscle pain and cramping are frequent side effects of statins, they attribute these symptoms to the diminished levels of coenzyme Q10 500.
Although most studies have used patients with preexisting medical conditions, one study of healthy participants did show that oral coenzyme Q10 supplementation improved fatigue and physical performance during bicycle exercise routines 501.
Coenzyme Q10 has also shown promise in migraine prevention 456. A cohort study of 1550 children and adolescents with headaches found that this population has low coenzyme Q10 levels 502. Coenzyme Q10 supplementation appeared to decrease headache frequency 456 A recent study indicated that CoQ10 is beneficial for the prophylactic treatment of migraine headaches in children without significant adverse effects 503. A double-blind, randomized controlled trial showed Coenzyme Q10 300 mg daily to be safe and superior to a placebo for migraine prevention 504. Another randomized, double-blind, placebo-controlled trial in adult women showed that Coenzyme Q10 400 mg of supplementation decreased migraine frequency, severity, and duration 456. One study showed that only Coenzyme Q10 100 mg daily reduced the severity of headaches and the number of headaches per month in migraine sufferers 505.
Interestingly, CoQ10 levels may be decreased in those with acute influenza infection 506. However, studies on coenzyme Q10 supplementation in patients with acute influenza have yet to be done.
Coenzyme Q10 supplemented alongside standard psychiatric medical therapy appears to lessen symptoms of depression in patients with bipolar disorder 507. In patients with polycystic ovary syndrome (PCOS), Coenzyme Q10 supplementation may improve fasting blood glucose, insulin levels, and total testosterone levels 508.
Primary Coenzyme Q10 deficiency (COQ10D6) is a rare autosomal recessive disorder caused by COQ6 gene mutations involved in coenzymeQ biosynthesis with clinical features of steroid-resistant nephrotic syndrome (SRNS), optic atrophy, retinopathy, and encephalopathy 509, 510. Coenzyme Q10 replacement therapy is indicated for this rare genetic disorder 509, 510. In patients with primary Coenzyme Q10 deficiency (COQ10D6), early treatment with high-dose Coenzyme Q10 supplementation (ranging from 5 to 50 mg/kg/day) can limit disease progression 509. A recent study of CoQ10 supplementation (20 mg/kg) demonstrated promising results in patients with primary CoQ10 deficiency with nephrotic syndrome and steroid-resistant nephrotic syndrome (SRNS) 509. Children with severe multisystem CoQ10 deficiency respond poorly to treatment and generally die within the neonatal period or in the first year of life 509.
Turmeric curcumin
Curcumin also called Curcuma longa, Indian saffron, diarylheptanoid or diferuloylmethane, is a natural bright yellow pigment obtained from the tubers of turmeric (Curcuma longa plant) in the ginger family, is native to Southeast Asia and is grown commercially in that region, primarily in India 511, 512, 513. Curcumin is a well-known antioxidant due to its reactive oxygen species (ROS) scavenging property. Curcumin comprises between 1% and 6% by dry weight of whole turmeric extracts, other components being starch, fats, proteins, fiber, vitamins, minerals, and water 514. Turmeric is used as a culinary spice and traditional medicine. The turmeric root can be dried and made into capsules, tablets, extracts, powders or teas. Or it can be made into a paste to apply to the skin. Turmeric’s main active component — curcumin — is what gives a yellowish color to curry powder and is a major ingredient in curry powders common in many Indian and Asian dishes, and it is also used as a coloring for foods, fabrics and cosmetics. The extract of turmeric contains three major curcuminoids: Curcumin (60–70%), demethoxycurcumin (20–27%), and bisdemethoxycurcumin (10–15%) 515. The activities of turmeric are commonly attributed to curcuminoids (curcumin, demethoxycurcumin and others). Commercial preparations of turmeric and curcumin vary widely in curcuminoid content. The antiinflammatory effects of turmeric and curcumin are thought to be mediated by inhibition of leukotriene synthesis. Curcumin has also been reported to have anti-cancer effects mediated by inhibition of intracellular kinases. Turmeric and curcumin are under active investigation as antiinflammatory and anti-cancer agents, for treatment of diabetes and hyperlipidemia and as therapy of liver diseases including nonalcoholic steatohepatitis (NASH) 516. The scientific bases for the purported effects of turmeric and curcumin are not well established and rigorous proof of their effictiveness in any medical condition is lacking.
Turmeric may be unsafe for use during pregnancy in amounts greater than those commonly found in food. Little is known about whether it’s safe to use turmeric in amounts greater than those commonly found in food while breastfeeding. In one study, high doses of curcumin (8000 mg/day) do not cause side effects 517. In another study, curcumin was administered at doses up to 12 g/day for three months with no apparent toxicity 518. However, some studies have found that high concentrations of curcumin have some side effects. Tanwar et al 519 found that curcumin had antioxidant effects at lower concentrations (100 or 200 mg/kg/day), but curcumin had side effects of promoting lipid peroxidation at higher concentrations (400 mg/kg/day) in a rat model of myocardial necrosis induced by isoprenaline. In another study, Sun et al 520 found that curcumin (75 mg/kg/day) can induce histone hypoacetylation and inhibit the expression of transcription factors such as GATA4 early in heart development, ultimately leading to defects in heart development, such as thinning of the ventricular wall and septum. In general, curcumin may be detrimental to embryonic heart development during early pregnancy 520. Clinically, the common side effects of curcumin are flatulence, changes in liver enzymes and blood biochemical parameters 521, 522. The metabolism and lipid-lowering effects of curcumin are mainly carried out in the liver, which may be the main reason for the increase of liver enzymes 523.
Curcumin has been consumed for hundreds of years and is considered pharmacologically safe when taken at doses up to 100 mg/day 524, 525, 526. According to the Joint Food and Agriculture Organization/The World Health Organization (JECFA) report on food additives, the Acceptable Daily Intake Level (ADI) of curcumin is 0 to 0.1 mg/kg body weight 527. In clinical trials, curcumin was found to be safe and effective. In a randomized and controlled clinical trial involving patients with arthritis, curcumin was administered in doses ranging from 120 to 1500 mg for 4 to 36 weeks, reduced inflammation and pain levels, and was shown to be a safe treatment method 528. Also, in a clinical study comparing the administration of curcumin 500 mg (BCM-95®) 3 times/day and diclofenac 50 mg, 2 times/day for 28 days in patients with knee osteoarthritis, patients that received curcumin presented similar improvement in the severity of pain, reduction in flatulence episodes, no requirement of H2 blockers, weight reduction, and anti-ulcer effects, proving to be a very safe treatment method presenting a 13% adverse effect rate versus 38% in the diclofenac group 529. Furthermore, in chronic kidney disease subjects, supplementation with 500 mg of curcumin tablets, two times/day for six months, reduced plasma pro-inflammatory mediators and lipid peroxidation. During the long-term administration treatment, no serious adverse events were observed, confirming the safety profile of curcumin 530. In addition, in patients with non-alcoholic fatty liver disease (NAFLD), curcumin supplementation of 1000 mg/day in two doses for eight weeks was considered safe and well tolerated with no report of severe adverse events during the treatment 531. The US Food and Drug Administration (FDA) approved the curcumin as a “generally safe” (GRAS) compound 532.
Historically, turmeric was used in Ayurveda and other traditional Indian medical systems, as well as Eastern Asian medical systems such as traditional Chinese medicine. In India, it was traditionally used for disorders of the skin, upper respiratory tract infections, joints, indigestion and liver diseases. Today, curcumin and its derivatives (curcuminoids) have received immense attention due to their biofunctional properties such as antioxidant, anti-inflammatory, immune regulatory, antiviral, antifungal, antibacterial, anticancer, antidiabetic, neuro-protective, cardiovascular protective, and hepatoprotective effects 533, 534, 535, 536. These properties are attributed to the key elements in the curcumin structure 537. Turmeric is currently being promoted as a dietary supplement for a variety of conditions, including arthritis, digestive disorders, respiratory infections, allergies, liver disease, depression, and many others.
The metabolism of curcumin is critical for its potent biological activities as well as the beneficial effects on health 538. In the mammalian body, curcumin can be presented in three major forms and they are free, conjugated, and reduced curcumin states 539. Oral administration mainly metabolizes curcumin into the conjugated curcumin via glucuronidation and sulfation. Especially, the finding demonstrates that the gastrointestinal tract plays an important role in the glucuronidation of curcuminoids in human 540. In addition, intravenous or intraperitoneal administration leads to the reduction of curcumin into dihydrocurcumin, tetrahydrocurcumin, and hexahydrocurcumin 541. However, minor free and intact curcumin can be detected in plasma after the administration 542. On the other hand, the metabolism of curcuminoids mainly leads to reductive metabolites, for example, hexahydrocurcuminoids are the major reductive metabolites observed in both male and female rat liver 543.
So far, both pre-clinical and clinical studies of curcumin administration via the oral route have demonstrated the poor biodistribution of curcumin 544, 545. A typical example is a pharmacokinetic investigation performed on healthy human subjects, which revealed that even after high oral-dosage administration (Cmax) of 10 g and 12 g of curcumin, only 2.30 and 1.73 μg/mL of curcumin were found in the blood. This means curcumin undergoes several physiological changes as it passes through the liver and gut 546. Due to the low chemical stability and poor bioavailability of curcumin, more attention has been paid to its metabolites 547. Although the major structure of its metabolites is consistent with curcumin, the minor differences in the structure of metabolites improve their chemical stability. For example, the hexahydrocurcumin has the same phenolic groups or diketo moieties as curcumin, but has no olefinic double bonds, leading to hexahydrocurcumin being more stable than curcumin at a physiological pH of 7.4 548. In the intestine, the absorption of curcumin is poor, while the reductive and conjugated curcumin metabolites show moderate absorption 549. Hence, it is hypothesized that the biological effects of curcumin in tissues, such as liver and kidney, may be attributed to the curcumin metabolites 550. However, the metabolism or degradation of curcumin can affect the bioactivity of curcumin. A finding shows that curcumin induces arrest in the G2/M phase and mitotic catastrophe in three human cancer cell lines, while the reductive metabolism and chemical degradation inactivate the ability of curcumin to cause cancer cell death 551.
The relationship between curcumin and microbiota (community of micro-organisms) plays an important role in the gastrointestinal metabolism of curcumin 552. The main metabolic process in human intestinal microbiota includes demethoxylation, reduction, hydroxylation, methylation, and acetylation 553. A study used the bacteria strain, Bacillus megaterium DCMB-002, isolated from mice feces to treat with curcumin, and the bacteria have been found to transform curcumin into various metabolites 554. In addition, the human intestinal bacteria, Blautia sp. MRG-PMF1, have been reported to biotransform curcumin via the methyl aryl ether cleavage reaction 555.
Figure 15. Three major curcuminoids in turmeric and their chemical structures
[Source 556 ]Figure 16. Metabolic pathways of curcumin
Footnotes: Oral administration mainly metabolizes curcumin into conjugated curcumin, while intravenous or intraperitoneal administration mainly leads to reduced curcumin. In addition, minor free and intact curcumin can be detected in plasma after any administration.
[Source 556 ]Lycopene
Lycopene (chemical formula of C40H56) is a linear unsaturated hydrocarbon carotenoid, the major bright red pigment, a phytochemical found in fruits such as tomatoes and other red fruits such as papaya, pink grapefruit, apricots, red oranges, watermelon, rosehips and guavas 557, 558, 559, 560, 561. Human body cannot make lycopene on its own, so it needs to be replenished from food 562, 563. It has been estimated that 80% of the lycopene in the US diet comes from tomatoes and tomato products like tomato sauce, tomato paste, and ketchup 564, 565, 566, 567, 568. Tomatoes are also the cheapest source for lycopene production 569. The tomato-based products are a better source of Lycopene than raw tomatoes 566. In the United States, Lycopene consumption among men ranges from 6.6 to 10.5 mg/day and among women from 5.7 to 10.4 mg/day 570. In the European population, the average consumption of lycopene ranges from 5 to 7 mg/day 571. Eating food containing lycopene can improve body’s immune system, regulate blood fats, and prevent cardiovascular diseases 572. High intakes of lycopene-rich food or supplements may result in a deep orange discoloration of the skin known as lycopenodermia. Because lycopene is more intensely colored than the carotenes, deep orange discoloration of the skin (lycopenodermia) may occur at lower doses than carotenodermia (orange discoloration of the outermost skin layer due to excess of dietary carotenoids) 573.
Lycopene is not a provitamin A carotenoid because it cannot be converted to retinol. Lycopene is a natural pigment made by plants, lycopene helps to protect plants from light-induced stress and it also transfers light energy during photosynthesis 574. Lycopene is the most common carotenoid in the human body and is one of the most potent carotenoid antioxidants and scavenger of free radicals with antioxidant activity ranking of lycopene > alpha-carotene > beta-cryptoxanthin > zeaxanthin = beta carotene > lutein 575, 576. Lycopene oxidation potential is 100 times that of vitamin E and is more than twice that of carotene 577, 578. Lycopene as antioxidant can neutralize reactive oxygen species (ROS) such as hydrogen peroxide, hydroxyradicals, and nitrogen dioxide. As an antioxidant, lycopene is increasingly used in food, pharmaceuticals, and cosmetics 579. Lycopene has also been shown to be a hypolipidemic agent to reduce lipid levels in the body which has potential for reducing cardiovascular disease, inhibitor of pro-inflammatory and pro-thrombotic factors, decreasing genetic damage, and inhibiting the occurrence and development of tumors 580, 581, 582, 583, 584, 585. Its name is derived from the tomato’s species classification, Solanum lycopersicum 586.
In North America, 85% of dietary lycopene comes from tomato products such as tomato juice or paste. One cup (240 mL) of tomato juice provides about 23 mg of lycopene 587. Processing raw tomatoes using heat (in the making of tomato juice, tomato paste or ketchup, for example) actually changes the lycopene in the raw product into a form that is easier for the body to use. The lycopene in supplements is about as easy for the body to use as lycopene found in food.
- Lycopene’s absorption is improved with concurrent dietary fat intake, this is because lycopene is fat soluble and oil in tomato sauce, for example, makes it ideal for absorbing lycopene. There is evidence that dietary fat may help increase the absorption of carotenoids, including lycopene. In one experiment, healthy volunteers consumed mixed-vegetable salads with nonfat, low-fat, or full-fat salad dressing. Analysis of blood samples indicated that eating full-fat salad dressing led to more carotenoid absorption than eating low-fat or nonfat dressing. Results of a randomized study published in 2005 demonstrated that cooking diced tomatoes with olive oil significantly increased lycopene absorption compared with cooking tomatoes without olive oil. In another study, there was no difference in plasma lycopene levels following consumption of tomatoes mixed with olive oil or tomatoes mixed with sunflower oil, suggesting that absorption of lycopene may not be dependent on the type of oil used. However, this study found that combining olive oil, but not sunflower oil, with tomatoes resulted in greater plasma antioxidant activity.
Lycopene is the most abundant carotenoid in the human body, accounting for about 50% of the total carotenoid content 588. Lycopene is widely disseminated in various organs and tissues of the human body, mainly including blood, adrenal glands, testicles, and liver 589. Generally, the content of lycopene in the serum is used to represent nutritional status of human lycopene 590. Under normal conditions, the concentration of lycopene in human serum is in the range of 0.2 ∼ 1.0 µmol/L, accounting for 6.35% of total lycopene content 591. It was noted that the lycopene in plants is mainly in the trans configuration, while the proportion of cis-isomer of lycopene in human body is higher 590. Cis-isomer lycopene has stronger bioavailability and can be digested and absorbed more easily 592. After the human body obtains lycopene from plants, lycopene enters the intestinal tract in packages of oil droplets, when are then transformed into mixed micelles 593. Through digestion and absorption, the lycopene-containing chylomicrons produced by enzyme cleavage are circulated in the blood or are transported to various body organs via lipoproteins 594.
Lycopene has been investigated for its role in chronic diseases, including cardiovascular disease and cancer 595. Numerous epidemiological studies suggest that lycopene may help prevent cardiovascular disease by decreasing cholesterol synthesis and increasing the degradation of low-density lipoproteins (LDL or “bad” cholesterol) 596, although some interventional studies have shown mixed results 585.
A number of test tube and animal studies suggest that lycopene may also be protective against cancers of the skin, breast, lung, and liver 597, 595. However, epidemiological studies have yielded inconsistent findings regarding lycopene’s potential in reducing cancer risk.
The few human intervention trials have been small and generally focused on intermediate endpoints and not response of clinically evident disease or overall survival and thus have limited translation to practice 574, 598. A Cochrane Review in 2011 concluded there is insufficient evidence to either support, or refute, the use of lycopene for the prevention of prostate cancer. Similarly, there is no robust evidence from randomized controlled trials to identify the impact of lycopene consumption upon the incidence of prostate cancer, prostate symptoms, PSA levels or adverse events 598.
On the basis of overall evidence, the association between tomato consumption and reduced risk of prostate cancer is limited 599.
Figure 17. Lycopene chemical structure
[Source 600 ]Figure 18. Lycopene isomers
Footnotes: Natural occurrence of lycopene shows that it exists mostly as a trans-isomer 601, while in human tissues and plasma, it appears as a cis-isomer 602. There are four common cis-isomers of lycopene, 15-cis, 13-cis, 9-cis, and 5-cis lycopene. All trans-lycopene predominates in tomato products, suggesting that all trans-lycopene is isomerised in the body or is less bioavailable. Cis-lycopene has stronger bioavailability and can be digested and absorbed more easily 592. After the human body obtains lycopene from plants, lycopene enters the intestinal tract in packages of oil droplets, when are then transformed into mixed micelles 593. Through digestion and absorption, the lycopene-containing chylomicrons produced by enzyme cleavage are circulated in the blood or are transported to various body organs via lipoproteins 594.
[Source 603 ]Table 13. Lycopene content in some fruits
Fruits name | Species | Organ | Lycopene content (mcg/g) |
---|---|---|---|
Autumn olive | Elaeagnus umbellata | Fruit | 150 ∼ 540 |
Carrot | Daucus carota | Root | 70 |
Guavas | Psidium guajava | Fruit | 35.5 |
Grapefruits | Citrus paradisi | Fruit | 6.1 (pulp) 0.32 (flavedo) |
Mango | Mangifera indica | Fruit | 0.53 |
Papaya | Carica papaya | Fruit | 14.4 ∼ 33.9 |
Persimmon | Diospyros kaki | Fruit | 270 |
Pummelo | Citrus grandis | Fruit | 5.83 |
Rose hip | Rosa canina | Fruit | 129 ∼ 352 |
Tomato | Lycopersicon esculentum | Fruit | 26.2 ∼ 6290 |
Watermelon | Citrullus lanatus | Fruit | 36.5 ∼ 69.2 |
Footnote: Lycopene content expressed as fresh weight except dry weight in persimmon
[Source 589 ]Table 14. Lycopene content of selected foods
Food | Serving | Lycopene (mg) |
---|---|---|
Tomato paste, canned | 1 cup | 75.5 |
Tomato purée, canned | 1 cup | 54.5 |
Tomato juice, canned | 1 cup | 22 |
Vegetable juice cocktail, canned | 1 cup | 17.3 |
Tomato soup, canned, condensed | 1 cup | 16.1 |
Watermelon, raw | 1 cup | 6.9 |
Tomato, raw | 1 medium | 3.2 |
Ketchup (catsup) | 1 tablespoon | 2.1 |
Pink or red grapefruit, raw | ½ grapefruit | 1.8 |
Baked beans, canned | 1 cup | 1.3 |
Beta-carotene
Beta-carotene is a red-orange pigment found in plants and fruits, especially carrots and colorful vegetables. Beta-carotene is the yellow/orange pigment that gives vegetables and fruits their rich colors. In Latin, the word “carotene” stands for carrot. This is the origin of the present name. Beta-carotene is one of the most widely consumed provitamin A carotenoids. Fruit and vegetables provide most of the 40 to 50 carotenoids found in the human diet. There are more than 50 provitamin A carotenoids, but only beta-carotene, alpha-carotene, lutein, lycopene, and beta-cryptoxanthin are present in significant amounts in the human diet 605. Currently, the only essential function of carotenoids recognized in humans is that of the provitamin A carotenoids, alpha-carotene, beta-carotene, and beta-cryptoxanthin, to serve as a source of vitamin A 573. The human body is not able to produce vitamin A, therefore, it is necessary to obtain it from the diet either as preformed vitamin A or in the form of provitamin A carotenoids. The human diet contains two sources for vitamin A: preformed vitamin A (retinol and retinyl esters) and provitamin A carotenoids 29, 573. Preformed vitamin A is found in foods from animal sources, including dairy products, eggs, fish, and organ meats 29, 35. Provitamin A carotenoids are plant pigments that include beta-carotene, alpha-carotene, and beta-cryptoxanthin 29. The body converts provitamin A carotenoids into vitamin A in the intestine via the beta-carotene monooxygenase type 1 BCMO1 enzyme 29, 573, 31. Although beta-carotene can be converted to vitamin A, the conversion of beta-carotene to vitamin A decreases when body stores of vitamin A are high and the conversion rates may have genetic variability 24, 32, 33, 34. This may explain why high doses of beta-carotene have never been found to cause vitamin A toxicity. High doses of beta-carotene (up to 180 mg/day) have been used to treat erythropoietic protoporphyria (EPP), a photosensitivity disorder, without toxic side effects 573.
Beta-carotene is a precursor for vitamin A biosynthesis with antioxidant properties including free radical scavenging, singlet oxygen quencher, and an ability to inhibit lipid peroxidation with high-fat solubility 606, 607. Other carotenoids in food, such as lycopene, lutein, and zeaxanthin, are not converted into vitamin A and are referred to as non-provitamin A carotenoids; they might have other important activities not involving vitamin A formation 29.
Studies have found out that majority of the vitamin A in our diet comes from provitamin A carotenoids like beta-carotene. There are also other sources for beta-carotene. Beta-carotene can be manufactured as a synthetic compound in laboratories or also can be isolated from different fungi or algal sources. Palm oil is another rich product containing beta-carotene 608.
Humans obtain vitamin A primarily from beta-carotene and other provitamin A carotenoids present in most fruits and vegetables. Vitamin A is involved in immune function, cellular communication, growth and development, and male and female reproduction 29, 35, 609. Vitamin A supports cell growth and differentiation, playing a critical role in the normal formation and maintenance of the heart, lungs, eyes, and other organs 29, 35. Vitamin A is also critical for vision as an essential component of rhodopsin, the light-sensitive protein in the retina that responds to light entering the eye, and because it supports the normal differentiation and functioning of the conjunctival membranes and cornea 26. Epidemiological studies recommended the daily intake of beta-carotene protect against diabetes, cardiovascular diseases, rheumatoid arthritis, atherosclerosis, inflammatory bowel disease, and cancer 610, 611.
Provitamin A carotenoids (alpha-carotene, beta-carotene, and beta-cryptoxanthin) are less easily absorbed than preformed vitamin A (retinol and retinyl esters) and must be converted to retinol and other retinoids by the body 612. The efficiency of conversion of provitamin A carotenoids (alpha-carotene, beta-carotene, and beta-cryptoxanthin) into preformed vitamin A retinol is highly variable, depending on factors like food matrix, food preparation, and one’s digestive and absorptive capacities 613.
The most recent international standard of measure for vitamin A is retinol activity equivalent (RAE), which represents vitamin A activity as retinol. It has been determined that 2 micrograms (µg) of beta-carotene in oil provided as a supplement could be converted by the body to 1 µg of retinol, giving it an RAE ratio of 2:1. However, 12 µg of beta-carotene from food are required to provide the body with 1 µg of retinol, giving dietary beta-carotene an RAE (retinol activity equivalent) ratio of 12:1. Other provitamin A carotenoids in food are less easily absorbed than beta-carotene, resulting in RAE ratios of 24:1. RAE ratios are shown in Table 1.
There has been an extensive number of studies carried out and research papers published on the health benefits and harms of beta carotene such as increase the risk of lung cancer, cardiovascular disease morbidity and mortality, and all-cause mortality. Examples are provided below.
In Finland, the Α-Tocopherol Βeta-Carotene (ATBC) cancer prevention trial 56 evaluated the effects of 20 mg/day of beta-carotene and/or 50 mg/day of vitamin E (alpha-tocopherol) on more than 29,000 male smokers and in the United States, the Beta-Carotene And Retinol Efficacy Trial (CARET) 614 evaluated the effects of a combination of 30 mg/day of beta-carotene and 25,000 IU/day of retinol (preformed vitamin A) in 18,314 men and women who were smokers, former smokers, or had a history of occupational asbestos exposure. Unexpectedly, the risk of lung cancer in the groups taking beta-carotene supplements was increased by 16% after six years in the ATBC participants and by 28% after four years in the CARET participants. The risk of lung cancer is increased with high doses of beta-carotene. In contrast, the Physicians’ Health Study (PHS) examined the effect of beta-carotene supplementation (50 mg every other day) on cancer risk in 22,071 male physicians in the United States, of whom only 11% were current smokers 64. In that lower risk population, beta-carotene supplementation for more than 12 years was not associated with an increased risk of lung cancer. In addition, in the Linxian General Population trial conducted in about 29,000 Chinese participants, randomization to 15 mg/day of beta-carotene, 30 mg/day of vitamin E (alpha-tocopherol) and 15 µg/day of selenium, was not found to be associated with lung cancer mortality 10 years after the intervention ended 615. Moreover, five-year follow-up of the Age-Related Eye Disease Study 2 (AREDS2) trial found that beta-carotene supplementation nearly doubled the risk of developing lung cancer in former smokers compared to nonsmokers (current smokers did not receive beta-carotene supplements) 12. Age-Related Eye Disease Study 2 (AREDS2) was a multicenter, randomized double-blind, placebo-controlled trial that evaluated the effects of supplementation with antioxidant vitamins and minerals for five years to treat age-related macular degeneration (AMD). Finally, a meta-analysis of four randomized controlled trials, including but not limited to trials in high-risk populations like smokers, found beta-carotene supplementation alone or with retinol; 3.7 to 12 years increased the risk of lung cancer by 20% compared to control 51.
Although the reasons for the increase in lung cancer risk in people taking carotenoids supplement are not yet clear, several mechanisms have been proposed 616. Baseline beta-carotene status might be one factor that influences whether with beta-carotene supplementation promotes carcinogenesis in the lungs of smokers 617. The US Preventive Services Task Force (USPSTF) estimated that the risks of high-dose beta-carotene supplementation outweigh any potential benefits for cancer prevention and recommended against supplementation, especially in smokers or other high-risk populations 51, 618.
When taken by mouth, beta-carotene is likely safe when taken in appropriate amounts for certain medical conditions 29. But beta-carotene supplements are not recommended for general use. Beta-carotene supplements are possibly unsafe when taken by mouth in high doses, especially when taken long-term. The Food and Nutrition Board at the National Academies of Sciences, Engineering, and Medicine advises against the use of beta-carotene supplements for the general population, except as a provitamin A source to prevent vitamin A deficiency 97.
Figure 19. Provitamin A carotenoids
[Source 604 ]Figure 20. Beta carotene chemical structure
[Source 608 ]Table 15. Beta-carotene content in selected foods
Food Source | Beta-Carotene Content |
---|---|
Orange-red vegetables | |
Sweet potato | 20–22,600 a |
Bitter gourd | 17,040 b |
Winter squash (butternut) | 12,340–15,770 a |
Chili pepper | 100–15,400 a |
Carrot | 4350–8840 a |
Pumpkins | 70–6070 a |
Cantaloupe | 2448–3861 a |
Red pepper | 1441–2390 a |
Tomato | 59–1500 a |
Green vegetables | |
Drumstick leaves | 19,700 a |
Amaranth | 8600 a |
Kale | 1020–10,000 a |
Garden rocket | 7960 a |
Chicory | 3940–7310 a |
Wild rocket | 7010 a |
Dandelion | 6340 a |
Onion leaf | 4900 a |
Coriander | 4800 a |
Parsley | 4440–4680 a |
Spinach | 3100–4810 a |
Endive | 1340–4350 a |
Cress | 2720–3690 a |
Leek | 3190 a |
Lettuce | 870–2960 a |
Broccoli | 291–1750 a |
Fruits | |
Apricot | 585–3800 a |
Mango | 109–3210 a |
Persimmon | 3000 b |
Dates | 2950 a |
Guava | 1–2669 a |
Red grapefruit | 2580 a |
Papaya | 190–1050 a |
Cereals | |
Golden rice | 160–3700 a |
Maize | 171–1500 a |
Medicinal plants and herbs | |
Rose hips | 3600 a |
Marigold flowers | 940–20,600 a |
Dill | 5450 a |
Basil | 4820 a |
Others | |
Spirulina | 184,100–272,500 a |
Sea buckthorn oil | 16,740 c |
Red palm oil | 5000–5602 c |
Footnotes: a µg/100 g of fresh weight, b CE-β-carotene equivalent (µg/100 g of fresh weight)—also includes other carotenoids (content of β-carotene + 1/2 content of other vitamin A active carotenoids), c µg/100 g of oil.
[Source 26 ]Table 16. Beta carotene in selected foods
Food | Serving | Beta-carotene (mg) |
---|---|---|
Carrot juice, canned | 1 cup (8 fl. oz.) | 21.9 |
Pumpkin, canned | 1 cup | 17 |
Spinach, frozen, cooked | 1 cup | 13.8 |
Sweet potato, baked | 1 medium | 13.1 |
Carrots, cooked | 1 cup | 13 |
Collards, frozen, cooked | 1 cup | 11.6 |
Carrots, raw | 1 medium | 10.1 |
Pumpkin pie | 1 piece | 7.4 |
Turnip greens, cooked | 1 cup | 6.6 |
Winter squash, cooked | 1 cup | 5.7 |
Cantaloupe, raw | 1 cup | 4.5 |
Kale, cooked | 1 cup | 2 |
Pycnogenol
Pycnogenol is a patented, proprietary powder extract made exclusively from French maritime pine (Pinus pinaster Aiton) bark by Horphag Research (Geneva, Switzerland) 619, 620, 621. Pycnogenol is a standardized extract from the bark of the French maritime pine consists of a concentrate of polyphenols 622, 623. A pharmacokinetic study with volunteers ingesting Pycnogenol revealed that catechin, caffeic acid, ferulic acid, taxifolin and the metabolite M1 (δ-(3,4-dihydroxy-phenyl)-γ-valerolactone) were detectable in a nanomolar range in plasma 624. Moreover, about 65–75 % of the Pycnogenol extract are procyanidins that consist of catechin and epicatechin subunits of varying chain lengths 625, besides taxifolin, catechin, and phenol acids 626. The active ingredients in maritime pine can also be extracted from other sources, including peanut skin, grape seed, and witch hazel bark. Procyanidin is a powerful antioxidant also found in food such as grapes, berries, pomegranates, red wine and various nuts. Maritime pine bark extract, pycnogenol, is commonly used orally to treat and prevent diabetes, diabetes-related health issues, and problems of the heart and blood vessels among many other uses. Some people use skin creams that contain maritime pine bark extract as “anti-aging” products. Pycnogenol is also applied to the skin to treat foot ulcers in people with diabetes, hemorrhoids, and mouth ulcers caused by chemotherapy. There is limited scientific research to support most of these uses.
French maritime pine bark extract is a complex mixture of polyphenolic compounds 627. A catechin metabolite (M1) produced by human intestinal bacteria was found in the plasma samples. Subsequent investigations showed that M1 exerted various anti-inflammatory effects in vitro such as the inhibition of the activity of the matrix metalloproteinases MMP-1, −2 and −9, decrease of the release of MMP-9 from human monocytes or inhibition of the expression of the inducible NO synthase (iNOS) in RAW 264.7 macrophages 628. In other in vitro experiments using the whole extract a decrease of IL1B mRNA synthesis in RAW 264.7 cells was reported 629 as well as inhibitory effects on the expression of COX-2, IL-8 and iNOS in human chondrocytes and fibroblasts 630. However, since not all components of the French maritime pine extract are bioavailable and other bioactive molecules such as M1 are generated in vivo, it is not clear whether experiments using the whole extract would be indicative for cellular effects that actually occur in vivo.
Clinical studies indicate that pycnogenol is effective in the treatment of chronic venous insufficiency and retinal micro-hemorrhages 631. Pycnogenol protects against oxidative stress in several cell systems by doubling the intracellular synthesis of anti-oxidative enzymes and by acting as a potent scavenger of free radicals. Other anti-oxidant effects involve a role in the regeneration and protection of vitamin C and E. Anti-inflammatory activity has been demonstrated in vitro and in vivo in animals. Protection against UV-radiation-induced erythema was found in a clinical study following oral intake of pycnogenol 631. In asthma patients symptom scores and circulating leukotrienes are reduced and lung function is improved 631. Immunomodulation has been observed in both animal models as well as in patients with Lupus erythematosus. Pycnogenol antagonizes the vasoconstriction caused by epinephrine and norepinephrine by increasing the activity of endothelial nitric oxide synthase. Dilation of the small blood vessels has been observed in patients with cardiovascular disease, whereas in smokers, pycnogenol prevents smoking-induced platelet aggregation and reduces the concentration of thromboxane 631. The ability to inhibit angiotensin-converting enzyme is associated with a mild antihypertensive effect 631. Pycnogenol relieves premenstrual symptoms, including abdominal pain and this action may be associated with the spasmolytic action of some phenolic acids 631. An improvement in cognitive function has been observed in controlled animal experiments and these findings support anecdotal reports of improvement in ADHD patients taking pycnogenol supplements 631.
Chemical identification studies showed that pycnogenol is primarily composed of procyanidins and phenolic acids. Procyanidins are biopolymers of catechin and epicatechin subunits which are recognized as important constituents in human nutrition. Pycnogenol contains a wide variety of procyanidins that range from the monomeric catechin and taxifolin to oligomers with 7 or more flavonoid subunits 631. The phenolic acids are derivatives of benzoic and cinnamic acids. The ferulic acid and taxifolin components are rapidly absorbed and excreted as glucuronides or sulphates in men, whereas procyanidins are absorbed slowly and metabolized to valerolactones which are excreted as glucuronides. As all of these constituents of Pycnogenol and its metabolites exhibit anti-inflammatory actions, the progressing appearance of the diverse active substances provides a long-lasting pain relief, so that patients feel less pain, also during the night 632. Pycnogenol has low acute and chronic toxicity with mild unwanted effects occurring in a small percentage of patients following oral administration.
Lutein
Lutein is a oxygenated carotenoid found naturally in vegetables and fruits 633. Lutein is synthesized only by plants and like other xanthophylls (xanthophylls is part of the carotenoid group; the other group is formed by the carotenes), is found in high quantities in all dark green leafy vegetables such as kale and spinach which contain 40 mg and 12 mg lutein in 100 g serving, respectively 634. Other foods rich in lutein include egg yolks, yellow carrots, corn, orange pepper, kiwi fruit, grapes, zucchini and squash 635, 636. In green plants, xanthophylls act to modulate light energy and serve as non-photochemical quenching agents to deal with triplet chlorophyll (an excited form of chlorophyll), which is overproduced at very high light levels, during photosynthesis 637.
Lutein is among the most abundant carotenoids in the human diet 638 often found combined with zeaxanthin. Lutein and zeaxanthin combined represent approximately 20% of the total carotenoids 639, 640. Zeaxanthin is generally found in much lower amounts in vegetables and is the dominant xanthophyll in only a few food products, such as the goji berry and orange pepper. Lutein consumed from dietary sources exceeds the amounts of zeaxanthin being consumed typically at a dietary ratio of 5:1.
The human eye accumulates the dietary lutein and zeaxanthin, which are both of dietary origin, and meso-zeaxanthin, which is derived from the conversion from lutein in the body. The maximum concentration of lutein and zeaxanthin (about 70% of their total content in the eye and more than a thousand times their concentration in serum) is observed in the macula lutea of the human retina, a small area of the retina responsible for central vision, where together with zeaxanthin it forms the macular pigment 641. These three carotenoids are efficient absorbers of blue light. They may prevent a substantial amount of the blue light entering the eye from reaching the underlying structures involved in vision and protect against light-induced oxidative damage, which is thought to play a role in the pathology of age-related macular degeneration (AMD) 642. It is also possible, though not proven, that lutein, zeaxanthin, and meso-zeaxanthin, act directly to neutralize oxidants formed in the retina.
Among the dietary carotenoids, only lutein and zeaxanthin are selectively deposited in this pigmented area of the retina responsible for high acuity color vision 643. The fact that lutein and zeaxanthin are highly concentrated in the macula strongly suggests that they might play a vital physiologic role 644. Zeaxanthin, which is fully conjugated (lutein is not), may offer somewhat better protection than lutein against phototoxic damage caused by blue and near ultraviolet light radiation 645, 637.
Lutein in the retina is believed to act as a yellow filter, filtering out potentially phototoxic blue light and near-ultraviolet radiation from the macula. The protective effect is due in part, to the reactive oxygen species (ROS) quenching ability of this carotenoid. The hypothesis is that lutein helps protect from oxidative stress and high-energy light. The specific ability of lutein and zeaxanthin to absorb high-energy short wavelengths of visible light (blue light) have an impact on vision beyond the protection of photoreceptor from blue-light induced production of reactive oxygen species (ROS). Blue light absorption can positively influence visual function by attenuating chromatic aberration, light scatter in the eye associated with disability glare, blue haze and improve contrast sensitivity 646, 647, 648, 649, 650. Supplemental lutein, alone or with zeaxanthin, was found to improve contrast sensitivity and protect against visual fatigue in young and/or healthy individuals 651, 652, 653, 654. Lutein has also been suggested to improve visual function through stimulating neuronal signaling efficiency in the eye 655.
Several studies show that an increase in macula pigmentation decreases the risk for eye diseases such as age-related macular degeneration (AMD) 656. Studies conducted in patients suffering from age-related macular degeneration (AMD) have shown the benefits of supplementation with 10 mg lutein in combination with 2 mg zeaxanthin or with other amounts in improving visual function and delaying the progression of AMD to more advanced disease stages 657, 658, 659, 660. Additional studies conducted in a healthy young population or more senior adults have shown that 10 mg lutein + 2 mg zeaxanthin supplementation increases macular pigment optical density (MPOD) and provide benefits on visual and cognitive health and function 661, 648, 662, 663, 664, 665, 666. Although possible (10 mg of lutein are found in 80 g of spinach), getting these nutrients in the adequate amount through the diet alone can be difficult on a regular daily basis especially in childhood.
The term macular pigment optical density (MPOD) refers to a measurement of the attenuation of blue light by macular pigment (expressed in density units [du]) and provides an indication of the amount of lutein and zeaxanthin isomers in the macula. Typical macular pigment optical density (MPOD) levels vary between 0 and 1 density units [du] 647. The concentration of macular carotenoids can be measured in the macula of donor eyes with high performance liquid chromatography (HPLC) while in living eyes MPOD can be assessed non-invasively using subjective psychophysical techniques, such as heterochromatic flicker photometry, and objective optical methods, such as fundus autofluorescence and reflectometry 647. In children up to the age of 7 years, due to the difficulties in performing psychophysical task reliably, objective measurements are preferred, and blue light reflectometry offers the opportunity to image the MP of infants and children 667. Preterm infants, who are highly susceptible to oxidative stress and related newborn diseases, were reported to have significantly lower serum and brain lutein concentrations than full-term infants 668, 669. Additionally, formula-fed infants were found to have lower serum lutein concentrations than breastfed infants 670, 671. A previous study reported that lutein/zeaxanthin concentration increased from 48 µg/L at birth to 96 µg/L at one month in breastfed newborns, while it decreased from 49 µg/L to 33 µg/L in infants fed with a formula that was not fortified with lutein 671. Children with higher lutein and zeaxanthin status reflected by higher MPOD were found to have better cognitive performance then their low MPOD peers 672. Therefore, increasing lutein intake through supplementation or fortification could benefit infants, especially those at risk 673.
Lutein and zeaxanthin research has focused on the beneficial effects for eye and brain aging 657, 658, 663, 665, 674, 675, 676 and more recently researchers have started to explore their effect for vision and cognition in young adults and early life 648, 662, 666, 677, 678, 679, 680, 681, 682.
Lutein acts as an antioxidant, protecting cells against the damaging effects of free radicals. Lutein like other Xanthophylls also can inhibit peroxidation of membrane phospholipids and reduce lipofuscin formation, both of which contribute to their antioxidant properties.
Lutein is more stable to decomposition by pro-oxidants than are other carotenoids such as beta-carotene and lycopene.
Lutein is one of only two carotenoids that have been identified in the human lens, may be protective against age-related increases in lens density and cataract formation 637. This study showed dietary lutein and zeaxanthin intake is associated with a reduced risk of age-related cataract, especially nuclear cataract in a dose-response manner, indicating a beneficial effect of lutein and zeaxanthin in age-related cataract prevention 683. Again, the possible protection afforded by lutein may be accounted for, in part, by its reactive oxygen species scavenging abilities. Carotenoids also provide protection from cancer 684, 685. One of the mechanisms of this is by increasing the expression of the protein connexin-43, thereby stimulating gap junctional communication and preventing unrestrained cell proliferation 637.
Lutein in most fruit and vegetables is in the free form 686, whereas both free and esterified lutein are available in commercial dietary supplements. Earlier studies found esterified and free lutein to be similarly bioavailable 687, 688. More recently, it was found that serum lutein and macular pigment optical density (MPOD) responses were similar between either free or esterified lutein supplementation for 3 months 689. However, a larger 4-week supplementation study found greater serum lutein responses from free lutein than from lutein ester supplements 690.
Lutein supplementation increases blood, tissue, and breast-milk carotenoids in a dose-response manner. In a current 140-day lutein supplementation study, serum lutein and macular pigment optical density (MPOD) changed positively, reaching a plateau that was linearly dependent on dose, across doses of 0, 5, 10, or 20 mg/day 691. Lutein supplementation also increased breast milk as well as infant and maternal plasma lutein concentrations in a dose-dependent manner 692. A recent meta-analysis concluded that lutein and zeaxanthin supplementation increases macular pigment optical density (MPOD) in patients with age-related macular degeneration (AMD) and healthy individuals in a dose-response manner 693.
Figure 21. Lutein chemical structure
[Source 672 ]Figure 22. Zeaxanthin
Table 17. Commonly consumed foods as high sources of lutein and zeaxanthin
Food | Lutein | Zeaxanthin |
---|---|---|
Vegetables | ||
Basil a | 70.5 | in |
Parsley a | 64–106.5 | in |
Spinach a | 59.3–79.0 | in |
Kale a | 48–114.7 | – |
Leek a | 36.8 | in |
Pea a | 19.1 | in |
Lettuce a | 10–47.8 | – |
Green pepper a | 8.8 | – |
Broccoli a | 7.1–33 | in |
Carrot a | 2.5–5.1 | in |
Red pepper a | 2.5–85.1 | 5.9–13.5 |
Eggs | ||
Egg yolk a | 3.84–13.2 | – |
Nuts | ||
Pistachio a | 7.7–49 | – |
Baked foods | ||
High lutein bread b | 36.7 | 3.3 |
High lutein cookie b | 21.3 | 2.9 |
High lutein muffin b | 26.1 | 3.7 |
Corn tortilla c | 72.5 | 105.3 |
Corn chips c | 61.1 | 92.5 |
Grains | ||
Corn d | 21.9 | 10.3 |
Einkorn wheat d | 7.4 | 0.9 |
Khorasan wheat d | 5.5 | 0.7 |
Durum wheat d | 5.4 | 0.5 |
Footnote: µg/g fresh weight except for corn tortilla and chips µg/g dry matter; in = included with lutein.
Data obtained from: a 694; b 695; c 696; d 697
[Source 698 ]Apigenin
Apigenin also called trihydroxyflavone (4′,5,7-trihydroxyflavone) is a plant-derived flavonoid or polyphenol found in vegetables, fruits and beverages such as parsley, celery, onions, grapes, apples, strawberries, oranges, chamomile tea and red wine 699, 700. It is generally accepted that people cannot synthesize apigenin but can only obtain it from food 701. Fresh parsley had the highest flavone concentrations of the fresh foods, with ≤1484 mg apigenin/100 g 702. Apigenin has many potential physiological functions including antioxidant anti-inflammatory, anticancer effects, lowering blood pressure, anti-genotoxic, antiallergic, neuroprotective, cardioprotective, antibacterial and antiviral properties and low toxicity 703, 704, 705, 706, 707, 708, 709, 710. In test tube studies, apigenin has been demonstrated to show broad anti-cancer effects in various types of cancer cells, including colorectal cancer, breast cancer, liver cancer, lung cancer, melanoma, prostate cancer and osteosarcoma 711, 712, 713, 714, 715, 716. In test tube studies, apigenin inhibits cancer cell proliferation by triggering cell apoptosis, inducing autophagy and modulating the cell cycle 717. Apigenin also decreases cancer cell motility and inhibits cancer cell migration and invasion 717. Recently, apigenin was reported to show anti-cancer activities by stimulating an immune response 718. Future human clinical trials are needed to evaluate and confirm the therapeutic as well as the preventive potential of apigenin.
Apigenin because of its low lipid (0.001–1.63 mg/mL) and water (2.16 μg/mL) solubility, it can be deactivated in the acidic environment of the gastrointestinal tract, leading to lower bioavailability, which limits its potential use in healthcare products and functional foods 719, 720, 721. The bioavailability of apigenin also depends on its bioaccessibility, which refers to the extraction of apigenin from the food matrix during gastrointestinal digestion and the transformation into compounds available for absorption 722. Glycosides of apigenin survive acid hydrolysis in the stomach, and it goes to the duodenum unbroken. Apigenin can be found in various plants as an aglycone or as a number of apigenin glycosides. Apigenin-7-O-glucoside, apigenin-6-C-glucoside (isovitexin), apigenin-8-C-glucoside (vitexin), api-genin-7-O-neohesperidoside (rhoifolin), and apigenin-6-C-glucoside-8-C-arabinoside are the common apigenin glycosides 723. The glycosylated forms of apigenin (e.g., 7-O-glucoside, 6-C-glucoside, or 8-C-glucoside) are broken down by beta-glucosidases in the stomach and small intestine to generate free apigenin 724. Eubacterium ramulus and Bacteroides distasonis have been identified as the main bacterial species essential for the biotransformation of 7-glycosides into free apigenin (aglycone apigenin) 725, 726. Apigenin taken orally is absorbed into the body with an availability of approximately 30% higher in the colon (40%) and lower in the terminal ileum (21%) via passive transport and independently of concentration 724.
Figure 23. Apigenin chemical structure
[Source 727 ]Figure 24. Apigenin and its glycosides
Footnotes: In nature, apigenin is typically found in a glycosylated form, with the tricyclic core structure linked to a sugar moiety through hydroxyl groups (O-glycosides) or directly to carbon (C-glycosides). The common apigenin glycosides are apiin, apigenin-7-O-glucoside, apigenin-8-C-glucoside (vitexin), apigenin-6-C-glucoside (isovitexin), apigenin-7-O-neohesperidoside (rhoifolin), and apigenin-6-C-glucoside 8-C-arabinoside (schaftoside) 728, 729, 730.
[Source 699 ]Figure 25. Apigenin health benefits
[Source 717 ]Table 18. Apigenin in fruits and vegetables
Food (serving size, g) | Flavone | Flavone, mg/100 g fresh weight | Flavone, mg/serving |
---|---|---|---|
Spinach (200 g) | Apigenin | <0.12 | <1 |
Luteolin | <0.13–6.6 | <1–13 | |
Parsley (5 g) | Apigenin | <2.44–1484 | <1–74 |
Luteolin | <0.44–22 | <1–1 | |
Celery stalks (200 g) | Apigenin | 1.3–10.8 | 3–22 |
Luteolin | <1.2–2.2 | <2–4 | |
Celery hearts (200 g) | Apigenin | 19.1 | 38 |
Luteolin | 3.5 | 7 | |
Chinese celery (200 g) | Apigenin | 24 | 48 |
Luteolin | 34.9 | 70 | |
Lettuce (100 g) | Apigenin | <0.75–2.7 | <1–3 |
Luteolin | <1.26–8.8 | <1–9 | |
Artichoke heads (200 g) | Apigenin/apigenin glycosides | 3.9–18.9 | 8–38 |
Luteolin/luteolin glycosides | 2.3–7.5 | 5–15 | |
Chicory leaves (100 g) | Apigenin/apigenin O-glycosides | <0.1–68 | <1–68 |
Luteolin/luteolin O-glycosides | <0.1–333 | <1–333 | |
Rutabaga (200 g) | Apigenin | <0.1–15.4 | <1–31 |
Luteolin | <0.1 | <1 | |
Chinese cabbage (200 g) | Apigenin | <0.1–4.5 | <1–9 |
Luteolin | <0.1–1.2 | <1–2 | |
Peas (100 g) | Apigenin | <0.12–17.6 | <1–18 |
Luteolin | <0.1–0.4 | <1 | |
Black olives (50 g) | Apigenin | 6.5 | 3 |
Luteolin | 3.2–17.5 | 2–9 | |
Olive oil (15 g) | Apigenin | <0.1–0.2 | <1 |
Luteolin | <0.1–0.7 | <1 | |
Grapefruit (200 g) | Apigenin/apigenin glycosides | <0.35–4.9 | <1–10 |
Luteolin | <1.2–1.4 | <1–3 | |
Kumquat (100 g) | Apigenin glycosides | 21.9 | 22 |
Honey (20 g) | Apigenin | <0.1–29.3 | <1–6 |
Luteolin | <0.49–3.2 | <1–1 |
Are antioxidant supplements safe?
Antioxidants can have harmful effects when taken at high doses 732. A few studies have raised the possibility that taking antioxidant supplements, either single agents or combinations, could interfere with your health.
- As discussed earlier, high-dose beta-carotene supplementation can increase people’s risks of lung cancer and cardiovascular disease. These effects have been seen primarily in people at high risk, such as smokers. The connection came in a large trial of beta-carotene conducted among men in Finland who were heavy smokers, and therefore at high risk for developing lung cancer 733. The trial was stopped early when researchers saw a significant increase in lung cancer among those taking beta-carotene supplement compared to those taking the placebo 733. In another trial among heavy smokers and people were exposed to asbestos, beta-carotene was combined with vitamin A 61. Again, an increase in lung cancer was seen in the supplement group 61.
- Research has not found any adverse effects from consuming vitamin E in food 734. However, high doses of vitamin E alpha-tocopherol supplements can cause bleeding and interrupt blood’s ability to form clots in animals, and in vitro data suggest that high doses inhibit platelet aggregation. Two clinical trials have found an increased risk of hemorrhagic stroke in participants taking alpha-tocopherol; one trial included Finnish male smokers who consumed 50 mg/day for an average of 6 years 56 and the other trial involved a large group of male physicians in the United States who consumed 400 IU (180 mg) of synthetic vitamin E every other day for 8 years 735. Because the majority of physicians in the latter study were also taking aspirin, this finding could indicate that vitamin E has a tendency to cause bleeding. Vitamin E supplements may also interact with certain medicines, including anticoagulant or antiplatelet medicines.
- High doses of vitamin C can cause diarrhea, nausea, and stomach cramps. Vitamin C supplements may also interact with cancer treatments, such as chemotherapy and radiation therapy, and they can worsen iron overload in people with hemochromatosis, a condition that causes the body to store too much iron.
- Combinations of antioxidants may have undesirable effects. For example, in one study, a combination of vitamin E, vitamin C, selenium, and beta-carotene reduced the cholesterol-lowering effects of two drugs taken together for this purpose.
In conclusion, although excessive free radicals contribute to chronic diseases including cancer, heart disease, cognitive decline, and vision loss. This doesn’t automatically mean that substances with antioxidant properties will fix the problem, especially if they are taken out of their natural context. The studies so far are inconclusive but generally don’t provide strong evidence that antioxidant supplements have a substantial impact on disease. At the same time, abundant evidence suggests that a healthy diet rich in fruits, vegetables, and whole grains—all rich in networks of naturally occurring antioxidants and their helper molecules—provides protection against many diseases and aging.
And if you are considering a dietary supplement, first get information on it from reliable sources. Keep in mind that dietary supplements may interact with medications or other supplements and may contain ingredients not listed on the label. Your doctor and pharmacist can advise you. If you are pregnant or breastfeeding a child or if you are considering giving a child a dietary supplement, it is especially important to consult your or your child’s doctor.
- Diplock AT, Charleux JL, Crozier-Willi G, Kok FJ, Rice-Evans C, Roberfroid M, Stahl W, Viña-Ribes J. Functional food science and defence against reactive oxidative species. Br J Nutr. 1998 Aug;80 Suppl 1:S77-112. doi: 10.1079/bjn19980106[↩][↩][↩]
- Valko M, Leibfritz D, Moncol J, Cronin MT, Mazur M, Telser J. Free radicals and antioxidants in normal physiological functions and human disease. Int J Biochem Cell Biol. 2007;39(1):44-84. doi: 10.1016/j.biocel.2006.07.001[↩][↩][↩]
- Reuter S, Gupta SC, Chaturvedi MM, Aggarwal BB. Oxidative stress, inflammation, and cancer: how are they linked? Free Radic Biol Med. 2010 Dec 1;49(11):1603-16. doi: 10.1016/j.freeradbiomed.2010.09.006[↩][↩]
- Biswas SK. Does the Interdependence between Oxidative Stress and Inflammation Explain the Antioxidant Paradox? Oxid Med Cell Longev. 2016;2016:5698931. doi: 10.1155/2016/5698931[↩]
- Stone WL, Basit H, Zubair M, et al. Pathology, Inflammation. [Updated 2024 Aug 11]. In: StatPearls [Internet]. Treasure Island (FL): StatPearls Publishing; 2024 Jan-. Available from: https://www.ncbi.nlm.nih.gov/books/NBK534820[↩]
- Carlsen MH, Halvorsen BL, Holte K, Bøhn SK, Dragland S, Sampson L, Willey C, Senoo H, Umezono Y, Sanada C, Barikmo I, Berhe N, Willett WC, Phillips KM, Jacobs DR Jr, Blomhoff R. The total antioxidant content of more than 3100 foods, beverages, spices, herbs and supplements used worldwide. Nutr J. 2010 Jan 22;9:3. doi: 10.1186/1475-2891-9-3[↩][↩][↩][↩][↩][↩][↩]
- Finkel T. Signal transduction by reactive oxygen species. J Cell Biol. 2011 Jul 11;194(1):7-15. doi: 10.1083/jcb.201102095[↩]
- Slauch JM. How does the oxidative burst of macrophages kill bacteria? Still an open question. Mol Microbiol. 2011 May;80(3):580-3. doi: 10.1111/j.1365-2958.2011.07612.x[↩]
- Stone WL, Pham T, Mohiuddin SS. Biochemistry, Antioxidants. [Updated 2023 May 1]. In: StatPearls [Internet]. Treasure Island (FL): StatPearls Publishing; 2024 Jan-. Available from: https://www.ncbi.nlm.nih.gov/books/NBK541064[↩]
- Stone WL, Bailey B, Khraisha N. The pathophysiology of smoking during pregnancy: a systems biology approach. Front Biosci (Elite Ed). 2014 Jun 1;6(2):318-28. doi: 10.2741/e708[↩]
- Stone WL, Shah D, Hollinger SM. Retinopathy of prematurity: an oxidative stress neonatal disease. Front Biosci (Landmark Ed). 2016 Jan 1;21(1):165-77. doi: 10.2741/4382[↩][↩]
- Chew EY, Clemons TE, Agrón E, Domalpally A, Keenan TDL, Vitale S, Weber C, Smith DC, Christen W; AREDS2 Research Group. Long-term Outcomes of Adding Lutein/Zeaxanthin and ω-3 Fatty Acids to the AREDS Supplements on Age-Related Macular Degeneration Progression: AREDS2 Report 28. JAMA Ophthalmol. 2022 Jul 1;140(7):692-698. doi: 10.1001/jamaophthalmol.2022.1640[↩][↩]
- Evans JR, Lawrenson JG. Antioxidant vitamin and mineral supplements for preventing age-related macular degeneration. Cochrane Database Syst Rev. 2017 Jul 30;7(7):CD000253. doi: 10.1002/14651858.CD000253.pub4[↩]
- Agrón E, Mares J, Clemons TE, Swaroop A, Chew EY, Keenan TDL; AREDS and AREDS2 Research Groups. Dietary Nutrient Intake and Progression to Late Age-Related Macular Degeneration in the Age-Related Eye Disease Studies 1 and 2. Ophthalmology. 2021 Mar;128(3):425-442. doi: 10.1016/j.ophtha.2020.08.018[↩]
- Agrón E, Domalpally A, Cukras CA, Clemons TE, Chen Q, Lu Z, Chew EY, Keenan TDL; AREDS and AREDS2 Research Groups. Reticular Pseudodrusen: The Third Macular Risk Feature for Progression to Late Age-Related Macular Degeneration: Age-Related Eye Disease Study 2 Report 30. Ophthalmology. 2022 Oct;129(10):1107-1119. doi: 10.1016/j.ophtha.2022.05.021[↩]
- Wong P, Markey M, Rapp CM, Darrow RM, Ziesel A, Organisciak DT. Enhancing the efficacy of AREDS antioxidants in light-induced retinal degeneration. Mol Vis. 2017 Oct 10;23:718-739[↩]
- Al-Zamil WM, Yassin SA. Recent developments in age-related macular degeneration: a review. Clin Interv Aging. 2017 Aug 22;12:1313-1330. doi: 10.2147/CIA.S143508[↩]
- Pérez S, Taléns-Visconti R, Rius-Pérez S, Finamor I, Sastre J. Redox signaling in the gastrointestinal tract. Free Radic Biol Med. 2017 Mar;104:75-103. doi: 10.1016/j.freeradbiomed.2016.12.048[↩]
- Stone WL, Krishnan K, Campbell SE, Palau VE. The role of antioxidants and pro-oxidants in colon cancer. World J Gastrointest Oncol. 2014 Mar 15;6(3):55-66. doi: 10.4251/wjgo.v6.i3.55[↩]
- Brieger K, Schiavone S, Miller FJ Jr, Krause KH. Reactive oxygen species: from health to disease. Swiss Med Wkly. 2012 Aug 17;142:w13659. doi: 10.4414/smw.2012.13659[↩]
- Aune D, Giovannucci E, Boffetta P, Fadnes LT, Keum N, Norat T, Greenwood DC, Riboli E, Vatten LJ, Tonstad S. Fruit and vegetable intake and the risk of cardiovascular disease, total cancer and all-cause mortality-a systematic review and dose-response meta-analysis of prospective studies. Int J Epidemiol. 2017 Jun 1;46(3):1029-1056. doi: 10.1093/ije/dyw319[↩]
- Fan H, Han X, Shang X, Zhu Z, He M, Xu G, Chen Z, Deng R. Fruit and vegetable intake and the risk of cataract: insights from the UK Biobank study. Eye (Lond). 2023 Oct;37(15):3234-3242. doi: 10.1038/s41433-023-02498-9[↩][↩]
- Mathew MC, Ervin AM, Tao J, Davis RM. Antioxidant vitamin supplementation for preventing and slowing the progression of age-related cataract. Cochrane Database Syst Rev. 2012 Jun 13;2012(6):CD004567. doi: 10.1002/14651858.CD004567.pub2[↩]
- Wang XD. Carotenoids. In: Ross CA, Caballero B, Cousins RJ, Tucker KL, Ziegler TR, eds. Modern Nutrition in Health and Disease. 11th ed: Lippincott Williams & Wilkins; 2014:427-439.[↩][↩][↩]
- Davis CD, Tsuji PA, Milner JA. Selenoproteins and cancer prevention. Annu Rev Nutr. 2012 Aug 21;32:73-95. doi: 10.1146/annurev-nutr-071811-150740[↩]
- Carazo A, Macáková K, Matoušová K, Krčmová LK, Protti M, Mladěnka P. Vitamin A Update: Forms, Sources, Kinetics, Detection, Function, Deficiency, Therapeutic Use and Toxicity. Nutrients. 2021 May 18;13(5):1703. doi: 10.3390/nu13051703[↩][↩][↩][↩]
- Vitamin A and Carotenoids. https://ods.od.nih.gov/factsheets/VitaminA-HealthProfessional/[↩][↩]
- Vitamin A. https://lpi.oregonstate.edu/mic/vitamins/vitamin-A[↩][↩]
- Blaner WS. Vitamin A and Provitamin A Carotenoids. In: Marriott BP, Birt DF, Stallings VA, Yates AA, eds. Present Knowledge in Nutrition. 11th ed. Cambridge, Massachusetts: Wiley-Blackwell; 2020:73-91.[↩][↩][↩][↩][↩][↩][↩][↩][↩][↩][↩][↩][↩][↩][↩][↩]
- Groff JL. Advanced Nutrition and Human Metabolism. 2nd ed. St. Paul: West Publishing; 1995.[↩]
- Kedishvili NY. Enzymology of retinoic acid biosynthesis and degradation. J Lipid Res. 2013 Jul;54(7):1744-60. doi: 10.1194/jlr.R037028[↩][↩]
- Zumaraga MPP, Arquiza JMRA, Concepcion MA, Perlas L, Alcudia-Catalma MN, Rodriguez M. Genotype Effects on β-Carotene Conversion to Vitamin A: Implications on Reducing Vitamin A Deficiency in the Philippines. Food Nutr Bull. 2022 Mar;43(1):25-34. doi: 10.1177/03795721211060229[↩][↩]
- Lietz G, Oxley A, Leung W, Hesketh J. Single nucleotide polymorphisms upstream from the β-carotene 15,15′-monoxygenase gene influence provitamin A conversion efficiency in female volunteers. J Nutr. 2012 Jan;142(1):161S-5S. doi: 10.3945/jn.111.140756[↩][↩]
- Leung WC, Hessel S, Méplan C, Flint J, Oberhauser V, Tourniaire F, Hesketh JE, von Lintig J, Lietz G. Two common single nucleotide polymorphisms in the gene encoding beta-carotene 15,15′-monoxygenase alter beta-carotene metabolism in female volunteers. FASEB J. 2009 Apr;23(4):1041-53. doi: 10.1096/fj.08-121962[↩][↩]
- Ross A. Vitamin A. In: Ross AC, Caballero B, Cousins RJ, Tucker KL, Ziegler TR, eds. Modern Nutrition in Health and Disease. 11th ed. Baltimore, MD: Lippincott Williams & Wilkins; 2014:260-77.[↩][↩][↩][↩][↩][↩][↩]
- Institute of Medicine. Food and Nutrition Board. Dietary Reference Intakes for Vitamin C, Vitamin E, Selenium, and Carotenoids. Washington, DC: National Academy Press; 2000.https://nap.nationalacademies.org/read/9810/chapter/1[↩]
- Vitamin C. https://ods.od.nih.gov/factsheets/VitaminC-HealthProfessional[↩][↩]
- Vitamin C. https://lpi.oregonstate.edu/mic/vitamins/vitamin-C[↩][↩]
- Li Y, Schellhorn HE. New developments and novel therapeutic perspectives for vitamin C. J Nutr. 2007 Oct;137(10):2171-84. doi: 10.1093/jn/137.10.2171[↩]
- Carr AC, Frei B. Toward a new recommended dietary allowance for vitamin C based on antioxidant and health effects in humans. Am J Clin Nutr. 1999 Jun;69(6):1086-107. doi: 10.1093/ajcn/69.6.1086[↩]
- Frei B, England L, Ames BN. Ascorbate is an outstanding antioxidant in human blood plasma. Proc Natl Acad Sci U S A. 1989 Aug;86(16):6377-81. doi: 10.1073/pnas.86.16.6377[↩]
- Jacob RA, Sotoudeh G. Vitamin C function and status in chronic disease. Nutr Clin Care. 2002 Mar-Apr;5(2):66-74. doi: 10.1046/j.1523-5408.2002.00005.x[↩]
- Traber MG. Vitamin E. In: Shils ME, Shike M, Ross AC, Caballero B, Cousins R, eds. Modern Nutrition in Health and Disease. 10th ed. Baltimore, MD: Lippincott Williams & Wilkins, 2006;396-411.[↩]
- Vitamin E. https://ods.od.nih.gov/factsheets/VitaminE-HealthProfessional[↩]
- He FJ, Nowson CA, Lucas M, MacGregor GA. Increased consumption of fruit and vegetables is related to a reduced risk of coronary heart disease: meta-analysis of cohort studies. J Hum Hypertens. 2007 Sep;21(9):717-28. doi: 10.1038/sj.jhh.1002212[↩]
- Lippman SM, Klein EA, Goodman PJ, et al. Effect of selenium and vitamin E on risk of prostate cancer and other cancers: the Selenium and Vitamin E Cancer Prevention Trial (SELECT). JAMA. 2009 Jan 7;301(1):39-51. doi: 10.1001/jama.2008.864[↩][↩][↩][↩]
- Klein EA, Thompson IM Jr, Tangen CM, Crowley JJ, Lucia MS, Goodman PJ, Minasian LM, Ford LG, Parnes HL, Gaziano JM, Karp DD, Lieber MM, Walther PJ, Klotz L, Parsons JK, Chin JL, Darke AK, Lippman SM, Goodman GE, Meyskens FL Jr, Baker LH. Vitamin E and the risk of prostate cancer: the Selenium and Vitamin E Cancer Prevention Trial (SELECT). JAMA. 2011 Oct 12;306(14):1549-56. doi: 10.1001/jama.2011.1437[↩][↩][↩][↩]
- Mursu J, Robien K, Harnack LJ, Park K, Jacobs DR Jr. Dietary supplements and mortality rate in older women: the Iowa Women’s Health Study. Arch Intern Med. 2011 Oct 10;171(18):1625-33. doi: 10.1001/archinternmed.2011.445[↩]
- Patterson RE, White E, Kristal AR, Neuhouser ML, Potter JD. Vitamin supplements and cancer risk: the epidemiologic evidence. Cancer Causes Control. 1997 Sep;8(5):786-802. doi: 10.1023/a:1018443724293[↩]
- Antioxidants and Cancer Prevention. https://www.cancer.gov/about-cancer/causes-prevention/risk/diet/antioxidants-fact-sheet[↩][↩][↩][↩][↩]
- O’Connor EA, Evans CV, Ivlev I, Rushkin MC, Thomas RG, Martin A, Lin JS. Vitamin and Mineral Supplements for the Primary Prevention of Cardiovascular Disease and Cancer: Updated Evidence Report and Systematic Review for the US Preventive Services Task Force. JAMA. 2022 Jun 21;327(23):2334-2347. doi: 10.1001/jama.2021.15650[↩][↩][↩]
- Fortmann SP, Burda BU, Senger CA, Lin JS, Whitlock EP. Vitamin and mineral supplements in the primary prevention of cardiovascular disease and cancer: An updated systematic evidence review for the U.S. Preventive Services Task Force. Ann Intern Med. 2013 Dec 17;159(12):824-34. doi: 10.7326/0003-4819-159-12-201312170-00729[↩]
- Bouayed J, Bohn T. Exogenous antioxidants–Double-edged swords in cellular redox state: Health beneficial effects at physiologic doses versus deleterious effects at high doses. Oxid Med Cell Longev. 2010 Jul-Aug;3(4):228-37. doi: 10.4161/oxim.3.4.12858[↩]
- Blot WJ, Li JY, Taylor PR, Guo W, Dawsey S, Wang GQ, Yang CS, Zheng SF, Gail M, Li GY, et al. Nutrition intervention trials in Linxian, China: supplementation with specific vitamin/mineral combinations, cancer incidence, and disease-specific mortality in the general population. J Natl Cancer Inst. 1993 Sep 15;85(18):1483-92. doi: 10.1093/jnci/85.18.1483[↩]
- Qiao YL, Dawsey SM, Kamangar F, Fan JH, Abnet CC, Sun XD, Johnson LL, Gail MH, Dong ZW, Yu B, Mark SD, Taylor PR. Total and cancer mortality after supplementation with vitamins and minerals: follow-up of the Linxian General Population Nutrition Intervention Trial. J Natl Cancer Inst. 2009 Apr 1;101(7):507-18. doi: 10.1093/jnci/djp037. Epub 2009 Mar 24. Erratum in: J Natl Cancer Inst. 2010 Jan 20;102(2):140.[↩]
- Alpha-Tocopherol, Beta Carotene Cancer Prevention Study Group. The effect of vitamin E and beta carotene on the incidence of lung cancer and other cancers in male smokers. N Engl J Med. 1994 Apr 14;330(15):1029-35. doi: 10.1056/NEJM199404143301501[↩][↩][↩]
- Rautalahti MT, Virtamo JR, Taylor PR, Heinonen OP, Albanes D, Haukka JK, Edwards BK, Kärkkäinen PA, Stolzenberg-Solomon RZ, Huttunen J. The effects of supplementation with alpha-tocopherol and beta-carotene on the incidence and mortality of carcinoma of the pancreas in a randomized, controlled trial. Cancer. 1999 Jul 1;86(1):37-42.[↩]
- Virtamo J, Edwards BK, Virtanen M, Taylor PR, Malila N, Albanes D, Huttunen JK, Hartman AM, Hietanen P, Mäenpää H, Koss L, Nordling S, Heinonen OP. Effects of supplemental alpha-tocopherol and beta-carotene on urinary tract cancer: incidence and mortality in a controlled trial (Finland). Cancer Causes Control. 2000 Dec;11(10):933-9. doi: 10.1023/a:1026546803917[↩]
- Albanes D, Malila N, Taylor PR, Huttunen JK, Virtamo J, Edwards BK, Rautalahti M, Hartman AM, Barrett MJ, Pietinen P, Hartman TJ, Sipponen P, Lewin K, Teerenhovi L, Hietanen P, Tangrea JA, Virtanen M, Heinonen OP. Effects of supplemental alpha-tocopherol and beta-carotene on colorectal cancer: results from a controlled trial (Finland). Cancer Causes Control. 2000 Mar;11(3):197-205. doi: 10.1023/a:1008936214087[↩]
- Wright ME, Virtamo J, Hartman AM, Pietinen P, Edwards BK, Taylor PR, Huttunen JK, Albanes D. Effects of alpha-tocopherol and beta-carotene supplementation on upper aerodigestive tract cancers in a large, randomized controlled trial. Cancer. 2007 Mar 1;109(5):891-8. doi: 10.1002/cncr.22482[↩]
- Omenn GS, Goodman GE, Thornquist MD, Balmes J, Cullen MR, Glass A, Keogh JP, Meyskens FL, Valanis B, Williams JH, Barnhart S, Hammar S. Effects of a combination of beta carotene and vitamin A on lung cancer and cardiovascular disease. N Engl J Med. 1996 May 2;334(18):1150-5. doi: 10.1056/NEJM199605023341802[↩][↩][↩]
- Goodman GE, Thornquist MD, Balmes J, Cullen MR, Meyskens FL Jr, Omenn GS, Valanis B, Williams JH Jr. The Beta-Carotene and Retinol Efficacy Trial: incidence of lung cancer and cardiovascular disease mortality during 6-year follow-up after stopping beta-carotene and retinol supplements. J Natl Cancer Inst. 2004 Dec 1;96(23):1743-50. doi: 10.1093/jnci/djh320[↩]
- Neuhouser ML, Barnett MJ, Kristal AR, Ambrosone CB, King IB, Thornquist M, Goodman GG. Dietary supplement use and prostate cancer risk in the Carotene and Retinol Efficacy Trial. Cancer Epidemiol Biomarkers Prev. 2009 Aug;18(8):2202-6. doi: 10.1158/1055-9965.EPI-09-0013[↩]
- Hennekens CH, Buring JE, Manson JE, Stampfer M, Rosner B, Cook NR, Belanger C, LaMotte F, Gaziano JM, Ridker PM, Willett W, Peto R. Lack of effect of long-term supplementation with beta carotene on the incidence of malignant neoplasms and cardiovascular disease. N Engl J Med. 1996 May 2;334(18):1145-9. doi: 10.1056/NEJM199605023341801[↩][↩][↩]
- Lee IM, Cook NR, Manson JE, Buring JE, Hennekens CH. Beta-carotene supplementation and incidence of cancer and cardiovascular disease: the Women’s Health Study. J Natl Cancer Inst. 1999 Dec 15;91(24):2102-6. doi: 10.1093/jnci/91.24.2102[↩]
- Lee IM, Cook NR, Gaziano JM, Gordon D, Ridker PM, Manson JE, Hennekens CH, Buring JE. Vitamin E in the primary prevention of cardiovascular disease and cancer: the Women’s Health Study: a randomized controlled trial. JAMA. 2005 Jul 6;294(1):56-65. doi: 10.1001/jama.294.1.56[↩]
- Hercberg S, Galan P, Preziosi P, Bertrais S, Mennen L, Malvy D, Roussel AM, Favier A, Briançon S. The SU.VI.MAX Study: a randomized, placebo-controlled trial of the health effects of antioxidant vitamins and minerals. Arch Intern Med. 2004 Nov 22;164(21):2335-42. doi: 10.1001/archinte.164.21.2335. Erratum in: Arch Intern Med. 2005 Feb 14;165(3):286.[↩][↩][↩]
- Hercberg S, Kesse-Guyot E, Druesne-Pecollo N, Touvier M, Favier A, Latino-Martel P, Briançon S, Galan P. Incidence of cancers, ischemic cardiovascular diseases and mortality during 5-year follow-up after stopping antioxidant vitamins and minerals supplements: a postintervention follow-up in the SU.VI.MAX Study. Int J Cancer. 2010 Oct 15;127(8):1875-81. doi: 10.1002/ijc.25201[↩]
- Hercberg S, Ezzedine K, Guinot C, Preziosi P, Galan P, Bertrais S, Estaquio C, Briançon S, Favier A, Latreille J, Malvy D. Antioxidant supplementation increases the risk of skin cancers in women but not in men. J Nutr. 2007 Sep;137(9):2098-105. doi: 10.1093/jn/137.9.2098[↩]
- Ezzedine K, Latreille J, Kesse-Guyot E, Galan P, Hercberg S, Guinot C, Malvy D. Incidence of skin cancers during 5-year follow-up after stopping antioxidant vitamins and mineral supplementation. Eur J Cancer. 2010 Dec;46(18):3316-22. doi: 10.1016/j.ejca.2010.06.008[↩]
- Lonn E, Bosch J, Yusuf S, Sheridan P, Pogue J, Arnold JM, Ross C, Arnold A, Sleight P, Probstfield J, Dagenais GR; HOPE and HOPE-TOO Trial Investigators. Effects of long-term vitamin E supplementation on cardiovascular events and cancer: a randomized controlled trial. JAMA. 2005 Mar 16;293(11):1338-47. doi: 10.1001/jama.293.11.1338[↩][↩]
- Gaziano JM, Glynn RJ, Christen WG, Kurth T, Belanger C, MacFadyen J, Bubes V, Manson JE, Sesso HD, Buring JE. Vitamins E and C in the prevention of prostate and total cancer in men: the Physicians’ Health Study II randomized controlled trial. JAMA. 2009 Jan 7;301(1):52-62. doi: 10.1001/jama.2008.862[↩]
- Lawenda BD, Kelly KM, Ladas EJ, Sagar SM, Vickers A, Blumberg JB. Should supplemental antioxidant administration be avoided during chemotherapy and radiation therapy? J Natl Cancer Inst. 2008 Jun 4;100(11):773-83. doi: 10.1093/jnci/djn148[↩][↩]
- Sayin VI, Ibrahim MX, Larsson E, Nilsson JA, Lindahl P, Bergo MO. Antioxidants accelerate lung cancer progression in mice. Sci Transl Med. 2014 Jan 29;6(221):221ra15. doi: 10.1126/scitranslmed.3007653[↩]
- Le Gal K, Ibrahim MX, Wiel C, Sayin VI, Akula MK, Karlsson C, Dalin MG, Akyürek LM, Lindahl P, Nilsson J, Bergo MO. Antioxidants can increase melanoma metastasis in mice. Sci Transl Med. 2015 Oct 7;7(308):308re8. doi: 10.1126/scitranslmed.aad3740[↩]
- Piskounova E, Agathocleous M, Murphy MM, Hu Z, Huddlestun SE, Zhao Z, Leitch AM, Johnson TM, DeBerardinis RJ, Morrison SJ. Oxidative stress inhibits distant metastasis by human melanoma cells. Nature. 2015 Nov 12;527(7577):186-91. doi: 10.1038/nature15726[↩]
- Evans JR, Lawrenson JG. Antioxidant vitamin and mineral supplements for slowing the progression of age-related macular degeneration. Cochrane Database Syst Rev. 2017 Jul 31;7(7):CD000254. doi: 10.1002/14651858.CD000254.pub4. Update in: Cochrane Database Syst Rev. 2023 Sep 13;9:CD000254. doi: 10.1002/14651858.CD000254.pub5[↩][↩][↩]
- Age-Related Eye Disease Study Research Group. A randomized, placebo-controlled, clinical trial of high-dose supplementation with vitamins C and E, beta carotene, and zinc for age-related macular degeneration and vision loss: AREDS report no. 8. Arch Ophthalmol. 2001 Oct;119(10):1417-36. doi: 10.1001/archopht.119.10.1417. Erratum in: Arch Ophthalmol. 2008 Sep;126(9):1251.[↩]
- Age-Related Eye Disease Study Research Group. A randomized, placebo-controlled, clinical trial of high-dose supplementation with vitamins C and E and beta carotene for age-related cataract and vision loss: AREDS report no. 9. Arch Ophthalmol. 2001 Oct;119(10):1439-52. doi: 10.1001/archopht.119.10.1439. Erratum in: Arch Ophthalmol. 2008 Sep;126(9):1251.[↩]
- The Age-Related Eye Disease Study 2 (AREDS2) Research Group. Secondary Analyses of the Effects of Lutein/Zeaxanthin on Age-Related Macular Degeneration Progression: AREDS2 Report No. 3. JAMA Ophthalmol. 2014;132(2):142–149. doi:10.1001/jamaophthalmol.2013.7376[↩]
- Christen WG, Glynn RJ, Gaziano JM, Darke AK, Crowley JJ, Goodman PJ, Lippman SM, Lad TE, Bearden JD, Goodman GE, Minasian LM, Thompson IM Jr, Blanke CD, Klein EA. Age-related cataract in men in the selenium and vitamin e cancer prevention trial eye endpoints study: a randomized clinical trial. JAMA Ophthalmol. 2015 Jan;133(1):17-24. doi: 10.1001/jamaophthalmol.2014.3478[↩]
- Antioxidants. https://nutritionsource.hsph.harvard.edu/antioxidants[↩]
- Lee I, Cook NR, Gaziano JM, et al. Vitamin E in the Primary Prevention of Cardiovascular Disease and Cancer: The Women’s Health Study: A Randomized Controlled Trial. JAMA. 2005;294(1):56–65. doi:10.1001/jama.294.1.56[↩][↩][↩]
- Dietary supplementation with n-3 polyunsaturated fatty acids and vitamin E after myocardial infarction: results of the GISSI-Prevenzione trial. Gruppo Italiano per lo Studio della Sopravvivenza nell’Infarto miocardico. Lancet. 1999 Aug 7;354(9177):447-55. Erratum in: Lancet 2001 Feb 24;357(9256):642. Erratum in: Lancet. 2007 Jan 13;369(9556):106.[↩]
- Milman U, Blum S, Shapira C, Aronson D, Miller-Lotan R, Anbinder Y, Alshiek J, Bennett L, Kostenko M, Landau M, Keidar S, Levy Y, Khemlin A, Radan A, Levy AP. Vitamin E supplementation reduces cardiovascular events in a subgroup of middle-aged individuals with both type 2 diabetes mellitus and the haptoglobin 2-2 genotype: a prospective double-blinded clinical trial. Arterioscler Thromb Vasc Biol. 2008 Feb;28(2):341-7. doi: 10.1161/ATVBAHA.107.153965[↩]
- Cook NR, Albert CM, Gaziano JM, Zaharris E, MacFadyen J, Danielson E, Buring JE, Manson JE. A randomized factorial trial of vitamins C and E and beta carotene in the secondary prevention of cardiovascular events in women: results from the Women’s Antioxidant Cardiovascular Study. Arch Intern Med. 2007 Aug 13-27;167(15):1610-8. doi: 10.1001/archinte.167.15.1610[↩]
- Marchese ME, Kumar R, Colangelo LA, Avila PC, Jacobs DR Jr, Gross M, Sood A, Liu K, Cook-Mills JM. The vitamin E isoforms α-tocopherol and γ-tocopherol have opposite associations with spirometric parameters: the CARDIA study. Respir Res. 2014 Mar 15;15(1):31. doi: 10.1186/1465-9921-15-31[↩][↩][↩]
- Berdnikovs S, Abdala-Valencia H, McCary C, Somand M, Cole R, Garcia A, Bryce P, Cook-Mills JM. Isoforms of vitamin E have opposing immunoregulatory functions during inflammation by regulating leukocyte recruitment. J Immunol. 2009 Apr 1;182(7):4395-405. doi: 10.4049/jimmunol.0803659. Erratum in: J Immunol. 2010 Jul 15;185(2):1341.[↩]
- Grodstein F, Kang JH, Glynn RJ, Cook NR, Gaziano JM. A randomized trial of beta carotene supplementation and cognitive function in men: the Physicians’ Health Study II. Arch Intern Med. 2007 Nov 12;167(20):2184-90. doi: 10.1001/archinte.167.20.2184[↩]
- Kryscio RJ, Abner EL, Caban-Holt A, et al. Association of Antioxidant Supplement Use and Dementia in the Prevention of Alzheimer’s Disease by Vitamin E and Selenium Trial (PREADViSE). JAMA Neurol. 2017;74(5):567–573. doi:10.1001/jamaneurol.2016.5778[↩][↩]
- Bjelakovic G, Nikolova D, Gluud LL, Simonetti RG, Gluud C. Mortality in Randomized Trials of Antioxidant Supplements for Primary and Secondary Prevention: Systematic Review and Meta-analysis. JAMA. 2007;297(8):842–857. doi:10.1001/jama.297.8.842[↩][↩]
- Bjelakovic G, Nikolova D, Gluud LL, Simonetti RG, Gluud C. Antioxidant supplements for prevention of mortality in healthy participants and patients with various diseases. Cochrane Database Syst Rev. 2012 Mar 14;2012(3):CD007176. doi: 10.1002/14651858.CD007176.pub2[↩][↩][↩]
- Joshipura KJ, Hu FB, Manson JE, Stampfer MJ, Rimm EB, Speizer FE, Colditz G, Ascherio A, Rosner B, Spiegelman D, Willett WC. The effect of fruit and vegetable intake on risk for coronary heart disease. Ann Intern Med. 2001 Jun 19;134(12):1106-14. doi: 10.7326/0003-4819-134-12-200106190-00010[↩][↩]
- Dagfinn Aune, Edward Giovannucci, Paolo Boffetta, Lars T Fadnes, NaNa Keum, Teresa Norat, Darren C Greenwood, Elio Riboli, Lars J Vatten, Serena Tonstad, Fruit and vegetable intake and the risk of cardiovascular disease, total cancer and all-cause mortality—a systematic review and dose-response meta-analysis of prospective studies, International Journal of Epidemiology, Volume 46, Issue 3, June 2017, Pages 1029–1056, https://doi.org/10.1093/ije/dyw319[↩][↩]
- Miller V, Mente A, Dehghan M, et al. Prospective Urban Rural Epidemiology (PURE) study investigators. Fruit, vegetable, and legume intake, and cardiovascular disease and deaths in 18 countries (PURE): a prospective cohort study. Lancet. 2017 Nov 4;390(10107):2037-2049. doi: 10.1016/S0140-6736(17)32253-5[↩][↩]
- Solomons NW. Vitamin A. In: Bowman B, Russell R, eds. Present Knowledge in Nutrition. 9th ed. Washington, DC: International Life Sciences Institute; 2006:157-83.[↩]
- Institute of Medicine. Food and Nutrition Board. Dietary Reference Intakes for Vitamin A, Vitamin K, Arsenic, Boron, Chromium, Copper, Iodine, Iron, Manganese, Molybdenum, Nickel, Silicon, Vanadium, and Zinc. Washington, DC: National Academy Press; 2001. https://nap.nationalacademies.org/read/10026/chapter/1[↩][↩]
- Bolat E, Sarıtaş S, Duman H, Eker F, Akdaşçi E, Karav S, Witkowska AM. Polyphenols: Secondary Metabolites with a Biological Impression. Nutrients. 2024 Aug 3;16(15):2550. doi: 10.3390/nu16152550[↩]
- Farhan M. The Promising Role of Polyphenols in Skin Disorders. Molecules. 2024 Feb 15;29(4):865. doi: 10.3390/molecules29040865[↩][↩]
- Vicente-Zurdo D, Gómez-Mejía E, Rosales-Conrado N, León-González ME. A Comprehensive Analytical Review of Polyphenols: Evaluating Neuroprotection in Alzheimer’s Disease. Int J Mol Sci. 2024 May 28;25(11):5906. doi: 10.3390/ijms25115906[↩][↩]
- Duda-Chodak A, Tarko T. Possible Side Effects of Polyphenols and Their Interactions with Medicines. Molecules. 2023 Mar 10;28(6):2536. doi: 10.3390/molecules28062536[↩]
- Grabska-Kobyłecka I, Szpakowski P, Król A, Książek-Winiarek D, Kobyłecki A, Głąbiński A, Nowak D. Polyphenols and Their Impact on the Prevention of Neurodegenerative Diseases and Development. Nutrients. 2023 Aug 4;15(15):3454. doi: 10.3390/nu15153454[↩]
- Benkhouili F.Z., Moutawalli A., Ouchari L., El Fahime E., Benzeid H., Doukkali A., Zahidi A. Evaluation of the Content of Polyphenols, Flavonoids and Tannins, the Antioxidant Capacity, and the Antimicrobial Activity of Different Organic and Aqueous Fractions of Stems of Retama Monosperma. Plant Sci. Today. 2024;11 doi: 10.14719/pst.2944[↩]
- Pandey KB, Rizvi SI. Plant polyphenols as dietary antioxidants in human health and disease. Oxidative Medicine and Cellular Longevity. 2009;2(5):270-278. https://www.ncbi.nlm.nih.gov/pmc/articles/PMC2835915/[↩][↩][↩]
- Dietary polyphenols and the prevention of diseases. Scalbert A, Manach C, Morand C, Rémésy C, Jiménez L. Crit Rev Food Sci Nutr. 2005; 45(4):287-306. https://www.ncbi.nlm.nih.gov/pubmed/16047496/[↩]
- Biomarkers of the intake of dietary polyphenols: strengths, limitations and application in nutrition research. Spencer JP, Abd El Mohsen MM, Minihane AM, Mathers JC. Br J Nutr. 2008 Jan; 99(1):12-22. https://www.ncbi.nlm.nih.gov/pubmed/17666146/[↩]
- Flavonols, flavones, flavanones, and human health: epidemiological evidence. Graf BA, Milbury PE, Blumberg JB. J Med Food. 2005 Fall; 8(3):281-90. https://www.ncbi.nlm.nih.gov/pubmed/16176136/[↩]
- Polyphenols and disease risk in epidemiologic studies. Arts IC, Hollman PC. Am J Clin Nutr. 2005 Jan; 81(1 Suppl):317S-325S. https://www.ncbi.nlm.nih.gov/pubmed/15640497/[↩]
- Dufresne C. J., Farnworth E. R. A review of latest research findings on the health promotion properties of tea. Journal of Nutritional Biochemistry. 2001;12(7):404–421. doi: 10.1016/S0955-2863(01)00155-3. https://www.ncbi.nlm.nih.gov/pubmed/11448616[↩]
- Khan N., Mukhtar H. Cancer and metastasis: prevention and treatment by green tea. Cancer and Metastasis Reviews. 2010;29(3):435–445. doi: 10.1007/s10555-010-9236-1. https://www.ncbi.nlm.nih.gov/pmc/articles/PMC3142888/[↩]
- Aggarwal B. B., Shishodia S. Molecular targets of dietary agents for prevention and therapy of cancer. Biochemical Pharmacology. 2006;71(10):1397–1421. doi: 10.1016/j.bcp.2006.02.009. https://www.ncbi.nlm.nih.gov/pubmed/16563357[↩]
- Garrido G., González D., Lemus Y., et al. In vivo and in vitro anti-inflammatory activity of Mangifera indica L. extract (VIMANG) Pharmacological Research. 2004;50(2):143–149. doi: 10.1016/j.phrs.2003.12.003. https://www.ncbi.nlm.nih.gov/pubmed/15177302[↩]
- Nichenametla S. N., Taruscio T. G., Barney D. L., Exon J. H. A review of the effects and mechanisms of polyphenolics in cancer. Critical Reviews in Food Science and Nutrition. 2006;46(2):161–183. doi: 10.1080/10408390591000541. https://www.ncbi.nlm.nih.gov/pubmed/16431408[↩]
- Zaveri N. T. Green tea and its polyphenolic catechins: medicinal uses in cancer and noncancer applications. Life Sciences. 2006;78(18):2073–2080. doi: 10.1016/j.lfs.2005.12.006. https://www.ncbi.nlm.nih.gov/pubmed/16445946[↩]
- Polyphenols: food sources and bioavailability. Manach C, Scalbert A, Morand C, Rémésy C, Jiménez L. Am J Clin Nutr. 2004 May; 79(5):727-47. https://ajcn.nutrition.org/article/S0002-9165(22)03914-4/fulltext[↩]
- Soleas G.J., Diamandis E.P., Goldberg D.M. Resveratrol: A molecule whose time has come? And gone? Clin. Biochem. 1997;30:91–113. doi: 10.1016/S0009-9120(96)00155-5[↩]
- Akinwumi B.C., Bordun K.M., Anderson H.D. Biological activities of stilbenoids. Int. J. Mol. Sci. 2018;19:792. doi: 10.3390/ijms19030792[↩]
- Salehi B., Mishra A.P., Nigam M., Sener B., Kilic M., Sharifi-Rad M., Fokou P.V.T., Martins N., Sharifi-Rad J. Resveratrol: A Double-Edged Sword in Health Benefits. Biomedicines. 2018;6:91. doi: 10.3390/biomedicines6030091[↩][↩]
- Raj P., Louis X.L., Thandapilly S.J., Movahed A., Zieroth S., Netticadan T. Potential of resveratrol in the treatment of heart failure. Life Sci. 2014;95:63–71. doi: 10.1016/j.lfs.2013.12.011[↩][↩]
- Neves A.R., Lucio M., Lima J.L., Reis S. Resveratrol in medicinal chemistry: A critical review of its pharmacokinetics, drug-delivery, and membrane interactions. Curr. Med. Chem. 2012;19:1663–1681. doi: 10.2174/092986712799945085[↩]
- Burns J., Yokota T., Ashihara H., Lean M.E., Crozier A. Plant foods and herbal sources of resveratrol. J. Agric. Food Chem. 2002;50:3337–3340. doi: 10.1021/jf0112973[↩]
- Farhan M., Rizvi A. The Pharmacological Properties of Red Grape Polyphenol Resveratrol: Clinical Trials and Obstacles in Drug Development. Nutrients. 2023;15:4486. doi: 10.3390/nu15204486[↩]
- Chen H.G., Tuck T., Ji X.H., Zhou X., Kelly G., Cuerrier A., Zhang J.Z. Quality Assessment of Japanese Knotweed (Fallopia japonica) Grown on Prince Edward Island as a Source of Resveratrol. J. Agric. Food Chem. 2013;61:6383–6392. doi: 10.1021/jf4019239[↩]
- Sun L., Hu Y., Mishra A., Sreeharsha N., Moktan J.B., Kumar P. et al.. (2020) Protective role of poly(lactic-co-glycolic) acid nanoparticle loaded with resveratrol against isoproterenol-induced myocardial infarction. Biofactors 10.1002/biof.1611[↩]
- Zhang Y., Zheng L., Zheng Y., Zhou C., Huang P., Xiao X., Zhao Y., Hao X., Hu Z., Chen Q., et al. Assembly and Annotation of a Draft Genome of the Medicinal Plant Polygonum Cuspidatum. Front. Plant Sci. 2019;10:1274. doi: 10.3389/fpls.2019.01274[↩]
- Tang K., Zhan J.C., Yang H.R., Huang W.D. Changes of resveratrol and antioxidant enzymes during UV-induced plant defense response in peanut seedlings. J. Plant Physiol. 2010;167:95–102. doi: 10.1016/j.jplph.2009.07.011[↩]
- Kang J.E., Yoo N., Jeon B.J., Kim B.S., Chung E.H. Resveratrol Oligomers, Plant-Produced Natural Products With Anti-virulence and Plant Immune-Priming Roles. Front. Plant Sci. 2022;13:885625. doi: 10.3389/fpls.2022.885625[↩]
- Takaoka M. Resveratrol, a new phenolic compound from Veratrum grandiflorum. J. Chem. Soc. Jpn. 1939;60:1090–1100.[↩]
- Guerrero R.F., Garcia-Parrilla M.C., Puertas B., Cantos-Villar E. Wine, resveratrol and health: A review. Nat. Prod. Commun. 2009;4:635–658. doi: 10.1177/1934578X0900400503[↩]
- Renaud S., de Lorgeril M. Wine, alcohol, platelets, and the French paradox for coronary heart disease. Lancet. 1992;339:1523–1526. doi: 10.1016/0140-6736(92)91277-F[↩]
- Gianchecchi E., Fierabracci A. Insights on the Effects of Resveratrol and Some of Its Derivatives in Cancer and Autoimmunity: A Molecule with a Dual Activity. Antioxidants. 2020;9:91. doi: 10.3390/antiox9020091[↩]
- Raj P, Thandapilly SJ, Wigle J, Zieroth S, Netticadan T. A Comprehensive Analysis of the Efficacy of Resveratrol in Atherosclerotic Cardiovascular Disease, Myocardial Infarction and Heart Failure. Molecules. 2021 Oct 31;26(21):6600. doi: 10.3390/molecules26216600[↩][↩]
- Glasgow M.D., Chougule M.B. Recent developments in active tumor targeted multifunctional nanoparticles for combination chemotherapy in cancer treatment and imaging. J. Biomed. Nanotechnol. 2015;11:1859–1898. doi: 10.1166/jbn.2015.2145[↩]
- Salehi B., Mishra A.P., Nigam M., Sener B., Kilic M., Sharifi-Rad M., Fokou P.V.T., Martins N., Sharifi-Rad J. Resveratrol: A Double-Edged Sword in Health Benefits. Biomedicines. 2018;6:91. doi: 10.3390/biomedicines6030091[↩]
- Wallerath T., Deckert G., Ternes T., Anderson H., Li H., Witte K., Forstermann U. Resveratrol, a polyphenolic phytoalexin present in red wine, enhances expression and activity of endothelial nitric oxide synthase. Circulation. 2002;106:1652–1658. doi: 10.1161/01.CIR.0000029925.18593.5C[↩]
- Gligorijević N, Stanić-Vučinić D, Radomirović M, Stojadinović M, Khulal U, Nedić O, Ćirković Veličković T. Role of Resveratrol in Prevention and Control of Cardiovascular Disorders and Cardiovascular Complications Related to COVID-19 Disease: Mode of Action and Approaches Explored to Increase Its Bioavailability. Molecules. 2021 May 11;26(10):2834. doi: 10.3390/molecules26102834[↩][↩][↩]
- Figueiras T.S., Neves-Petersen M.T., Petersen S.B. Activation Energy of Light Induced Isomerization of Resveratrol. J. Fluoresc. 2011;21:1897–1906. doi: 10.1007/s10895-011-0886-3[↩]
- Jarosova V., Vesely O., Doskocil I., Tomisova K., Marsik P., Jaimes J.D., Smejkal K., Kloucek P., Havlik J. Metabolism of cis- and trans-Resveratrol and Dihydroresveratrol in an Intestinal Epithelial Model. Nutrients. 2020;12:595. doi: 10.3390/nu12030595[↩]
- Jhanji M., Rao C.N., Sajish M. Towards resolving the enigma of the dichotomy of resveratrol: Cis- and trans-resveratrol have opposite effects on TyrRS-regulated PARP1 activation. Geroscience. 2020 doi: 10.1007/s11357-020-00295-w[↩]
- Zupancic S., Lavric Z., Kristl J. Stability and solubility of trans-resveratrol are strongly influenced by pH and temperature. Eur. J. Pharm. Biopharm. 2015;93:196–204. doi: 10.1016/j.ejpb.2015.04.002[↩]
- Meng X., Zhou J., Zhao C.N., Gan R.Y., Li H.B. Health Benefits and Molecular Mechanisms of Resveratrol: A Narrative Review. Foods. 2020;9:340. doi: 10.3390/foods9030340[↩]
- Almatroodi SA, A Alsahli M, S M Aljohani A, Alhumaydhi FA, Babiker AY, Khan AA, Rahmani AH. Potential Therapeutic Targets of Resveratrol, a Plant Polyphenol, and Its Role in the Therapy of Various Types of Cancer. Molecules. 2022 Apr 21;27(9):2665. doi: 10.3390/molecules27092665[↩]
- Abu-Amero K.K., Kondkar A.A., Chalam K.V. Resveratrol and ophthalmic diseases. Nutrients. 2016;8:200. doi: 10.3390/nu8040200[↩]
- Limmongkon A., Janhom P., Amthong A., Kawpanuk M., Nopprang P., Poohadsuan J., Somboon T., Saijeen S., Surangkul D., Srikummool M., et al. Antioxidant activity, total phenolic, and resveratrol content in five cultivars of peanut sprouts. Asian Pac. J. Trop. Biomed. 2017;7:332–338. doi: 10.1016/j.apjtb.2017.01.002[↩]
- Jang I.A., Kim E.N., Lim J.H., Kim M.Y., Ban T.H., Yoon H.E., Park C.W., Chang Y.S., Choi B.S. Effects of resveratrol on the renin-angiotensin system in the aging kidney. Nutrients. 2018;10:1741. doi: 10.3390/nu10111741[↩]
- Li Y.R., Li S., Lin C.C. Effect of resveratrol and pterostilbene on aging and longevity. BioFactors. 2018;44:69–82. doi: 10.1002/biof.1400[↩]
- Chen W.-M., Shaw L.-H., Chang P.-J., Tung S.-Y., Chang T.-S., Shen C.-H., Hsieh Y.-Y., Wei K.-L. Hepatoprotective effect of resveratrol against ethanol-induced oxidative stress through induction of superoxide dismutase in vivo and in vitro. Exp. Ther. Med. 2016;11:1231–1238. doi: 10.3892/etm.2016.3077[↩]
- Mokni M., Elkahoui S., Limam F., Amri M., Aouani E. Effect of resveratrol on antioxidant enzyme activities in the brain of healthy rat. Neurochem. Res. 2007;32:981–987. doi: 10.1007/s11064-006-9255-z[↩]
- Robb E.L., Winkelmolen L., Visanji N., Brotchie J., Stuart J.A. Dietary resveratrol administration increases MnSOD expression and activity in mouse brain. Biochem. Biophys. Res. Commun. 2008;372:254–259. doi: 10.1016/j.bbrc.2008.05.028[↩]
- Kitada M., Kume S., Imaizumi N., Koya D. Resveratrol improves oxidative stress and protects against diabetic nephropathy through normalization of Mn-SOD dysfunction in AMPK/SIRT1-independent pathway. Diabetes. 2011;60:634–643. doi: 10.2337/db10-0386[↩]
- Chedea V.S., Vicas S.I., Sticozzi C., Pessina F., Frosini M., Maioli E., Valacchi G. Resveratrol: From diet to topical usage. Food Funct. 2017;8:3879–3892. doi: 10.1039/C7FO01086A[↩]
- Poulsen M.M., Fjeldborg K., Ornstrup M.J., Kjaer T.N., Nohr M.K., Pedersen S.B. Resveratrol and inflammation: Challenges in translating pre-clinical findings to improved patient outcomes. Biochim. Biophys. Acta. 2015;1852:1124–1136. doi: 10.1016/j.bbadis.2014.12.024[↩]
- Yin S.Y., Wei W.C., Jian F.Y., Yang N.S. Therapeutic applications of herbal medicines for cancer patients. Evid. Based Complement. Altern. Med. 2013;2013:302426. doi: 10.1155/2013/302426[↩]
- Harvard University. Harvard Health Publications. Diet rich in resveratrol offers no health boost. http://www.health.harvard.edu/blog/diet-rich-resveratrol-offers-health-boost-201405157153[↩]
- Sharma S., Anjaneyulu M., Kulkarni S., Chopra K. Resveratrol, a polyphenolic phytoalexin, attenuates diabetic nephropathy in rats. Pharmacology. 2006;76:69–75. doi: 10.1159/000089720[↩]
- Gupta S.C., Kannappan R., Reuter S., Kim J.H., Aggarwal B.B. Chemosensitization of tumors by resveratrol. Ann. N. Y. Acad. Sci. 2011;1215:150–160. doi: 10.1111/j.1749-6632.2010.05852.x[↩]
- Opipari A.W., Jr., Tan L., Boitano A.E., Sorenson D.R., Aurora A., Liu J.R. Resveratrol-induced autophagocytosis in ovarian cancer cells. Cancer Res. 2004;64:696–703. doi: 10.1158/0008-5472.CAN-03-2404[↩]
- Tome-Carneiro J., Larrosa M., Gonzalez-Sarrias A., Tomas-Barberan F.A., Garcia-Conesa M.T., Espin J.C. Resveratrol and clinical trials: The crossroad from in vitro studies to human evidence. Curr. Pharm. Des. 2013;19:6064–6093. doi: 10.2174/13816128113199990407[↩]
- Aggarwal B.B., Bhardwaj A., Aggarwal R.S., Seeram N.P., Shishodia S., Takada Y. Role of resveratrol in prevention and therapy of cancer: Preclinical and clinical studies. Anticancer Res. 2004;24:2783–2840. https://ar.iiarjournals.org/content/24/5A/2783.long[↩]
- Siemann EH, Creasy L.L. Concentration of the phytoalexin resveratrol in wine. Am. J. Eno. Vitic. 1992;43:49–52.[↩]
- Cancer chemopreventive activity of resveratrol, a natural product derived from grapes. Science. 1997 Jan 10; 275(5297):218-20. https://www.ncbi.nlm.nih.gov/pubmed/8985016/[↩]
- Therapeutic potential of resveratrol: the in vivo evidence. Baur JA, Sinclair DA. Nat Rev Drug Discov. 2006 Jun; 5(6):493-506. https://www.ncbi.nlm.nih.gov/pubmed/16732220/[↩]
- What is new for an old molecule? Systematic review and recommendations on the use of resveratrol. PLoS One. 2011; 6(6):e19881. https://www.ncbi.nlm.nih.gov/pubmed/21698226/[↩]
- Shaito A., Posadino A.M., Younes N., Hasan H., Halabi S., Alhababi D., Al-Mohannadi A., Abdel-Rahman W.M., Eid A.H., Nasrallah G.K., et al. Potential Adverse Effects of Resveratrol: A Literature Review. Int. J. Mol. Sci. 2020;21:2084. doi: 10.3390/ijms21062084[↩][↩]
- Hyrsova L., Vanduchova A., Dusek J., Smutny T., Carazo A., Maresova V., Trejtnar F., Barta P., Anzenbacher P., Dvorak Z., et al. Trans-resveratrol, but not other natural stilbenes occurring in food, carries the risk of drug-food interaction via inhibition of cytochrome P450 enzymes or interaction with xenosensor receptors. Toxicol. Lett. 2019;300:81–91. doi: 10.1016/j.toxlet.2018.10.028[↩]
- Salehi B., Mishra A.P., Nigam M., Sener B., Kilic M., Sharifi-Rad M., Fokou P.V.T., Martins N., Sharifi-Rad J. Resveratrol: A double-edged sword in health benefits. Biomedicines. 2018;6:91. doi: 10.3390/biomedicines6030091[↩]
- Salehi B, Mishra AP, Nigam M, Sener B, Kilic M, Sharifi-Rad M, Fokou PVT, Martins N, Sharifi-Rad J. Resveratrol: A Double-Edged Sword in Health Benefits. Biomedicines. 2018 Sep 9;6(3):91. doi: 10.3390/biomedicines6030091[↩]
- Hopkins F.G. On glutathione, a reinvestigation. J. Biol. Chem. 1929;84:269–320. doi: 10.1016/S0021-9258(18)77062-2[↩]
- Hunter G., Eagles B.A. Glutathione. A critical study. J. Biol. Chem. 1927;72:147–166. doi: 10.1016/S0021-9258(18)84368-X[↩]
- Minich DM, Brown BI. A Review of Dietary (Phyto)Nutrients for Glutathione Support. Nutrients. 2019 Sep 3;11(9):2073. doi: 10.3390/nu11092073[↩][↩][↩][↩][↩][↩][↩][↩][↩][↩][↩][↩][↩]
- Simoni R.D., Hill R.L., Vaughan M. The discovery of glutathione by F. Gowland Hopkins and the beginning of biochemistry at Cambridge University. J. Biol. Chem. 2002;277:27–28. doi: 10.1016/S0021-9258(20)70350-9[↩]
- Pizzorno J. Glutathione! Integr Med (Encinitas). 2014 Feb;13(1):8-12. https://www.ncbi.nlm.nih.gov/pmc/articles/PMC4684116[↩][↩][↩][↩][↩][↩][↩][↩][↩][↩][↩][↩]
- Ballatori N, Krance SM, Notenboom S, Shi S, Tieu K, Hammond CL. Glutathione dysregulation and the etiology and progression of human diseases. Biol Chem. 2009 Mar;390(3):191-214. doi: 10.1515/BC.2009.033[↩][↩][↩]
- Lillig C.H., Berndt C. Preface. Cellular functions of glutathione. Biochim. Biophys. Acta. 2013;1830:3137–3138. doi: 10.1016/j.bbagen.2013.02.019[↩]
- McCarty MF, O’Keefe JH, DiNicolantonio JJ. Dietary Glycine Is Rate-Limiting for Glutathione Synthesis and May Have Broad Potential for Health Protection. Ochsner J. 2018 Spring;18(1):81-87. https://www.ncbi.nlm.nih.gov/pmc/articles/PMC5855430[↩][↩][↩][↩]
- Lu S.C. Glutathione synthesis. Biochim. Biophys. Acta. 2013;1830:3143–3153. doi: 10.1016/j.bbagen.2012.09.008[↩][↩][↩][↩][↩]
- Jones D.P., Park Y., Gletsu-Miller N., Liang Y., Yu T., Accardi C.J., Ziegler T.R. Dietary sulfur amino acid effects on fasting plasma cysteine/cystine redox potential in humans. Nutrition. 2011;27:199–205. doi: 10.1016/j.nut.2010.01.014[↩][↩][↩]
- Parcell S. Sulfur in human nutrition and applications in medicine. Altern Med Rev. 2002 Feb;7(1):22-44.[↩][↩]
- Skvarc D.R., Dean O.M., Byrne L.K., Gray L., Lane S., Lewis M., Fernanders B.S., Berk M., Marriott A. The effect of N-acetylcysteine (NAC) on human cognition—A systematic review. Neurosci. Biobehav. Rev. 2017;78:44–56. doi: 10.1016/j.neubiorev.2017.04.013[↩][↩][↩]
- Vašková J, Kočan L, Vaško L, Perjési P. Glutathione-Related Enzymes and Proteins: A Review. Molecules. 2023 Feb 2;28(3):1447. doi: 10.3390/molecules28031447[↩]
- Ballatori N., Krance S.M., Notenboom S., Shi S., Tieu K., Hammond C.L. Glutathione dysregulation and the etiology and progression of human diseases. Biol. Chem. 2009;390:191–214. doi: 10.1515/BC.2009.033[↩]
- Forman H.J., Zhang H., Rinna A. Glutathione: Overview of its protective roles, measurement, and biosynthesis. Mol. Asp. Med. 2009;30:1–12. doi: 10.1016/j.mam.2008.08.006[↩][↩]
- Forman HJ, Zhang H, Rinna A. Glutathione: overview of its protective roles, measurement, and biosynthesis. Mol Aspects Med. 2009 Feb-Apr;30(1-2):1-12. doi: 10.1016/j.mam.2008.08.006[↩]
- Jozefczak M, Remans T, Vangronsveld J, Cuypers A. Glutathione is a key player in metal-induced oxidative stress defenses. Int J Mol Sci. 2012;13(3):3145-3175. doi: 10.3390/ijms13033145[↩]
- Schafer FQ, Buettner GR. Redox environment of the cell as viewed through the redox state of the glutathione disulfide/glutathione couple. Free Radic Biol Med. 2001 Jun 1;30(11):1191-212. doi: 10.1016/s0891-5849(01)00480-4[↩]
- Ruparell A, Alexander JE, Eyre R, Carvell-Miller L, Leung YB, Evans SJM, Holcombe LJ, Heer M, Watson P. Glycine supplementation can partially restore oxidative stress-associated glutathione deficiency in ageing cats. Br J Nutr. 2024 Jun 28;131(12):1947-1961. doi: 10.1017/S0007114524000370[↩]
- Meister A, Anderson ME. Glutathione. Annu Rev Biochem. 1983;52:711-60. doi: 10.1146/annurev.bi.52.070183.003431[↩][↩]
- Marí M, Morales A, Colell A, García-Ruiz C, Fernández-Checa JC. Mitochondrial glutathione, a key survival antioxidant. Antioxid Redox Signal. 2009;11(11):2685–2700. doi: 10.1089/ARS.2009.2695[↩]
- Anderson M.E. Glutathione: An overview of biosynthesis and modulation. Chem. Biol. Interact. 1998;111–112:1–14. doi: 10.1016/S0009-2797(97)00146-4[↩][↩]
- Chasseaud LF. The role of glutathione and glutathione S-transferases in the metabolism of chemical carcinogens and other electrophilic agents. Adv Cancer Res. 1979;29:175-274. doi: 10.1016/s0065-230x(08)60848-9[↩]
- Lushchak V.I. Glutathione homeostasis and functions: Potential targets for medical interventions. J. Amino Acids. 2012;2012:736837. doi: 10.1155/2012/736837[↩]
- Glutathione synthetase deficiency. https://medlineplus.gov/genetics/condition/glutathione-synthetase-deficiency[↩][↩][↩][↩][↩]
- Maher P. The effects of stress and aging on glutathione metabolism. Ageing Res Rev. 2005 May;4(2):288-314. doi: 10.1016/j.arr.2005.02.005[↩][↩][↩]
- Kumar D, Rizvi SI. Markers of oxidative stress in senescent erythrocytes obtained from young and old age rats. Rejuvenation Res. 2014 Oct;17(5):446-52. doi: 10.1089/rej.2014.1573[↩]
- Zhu Y, Carvey PM, Ling Z. Age-related changes in glutathione and glutathione-related enzymes in rat brain. Brain Res. 2006 May 23;1090(1):35-44. doi: 10.1016/j.brainres.2006.03.063[↩]
- Park JS, Mathison BD, Hayek MG, Zhang J, Reinhart GA, Chew BP. Astaxanthin modulates age-associated mitochondrial dysfunction in healthy dogs. J Anim Sci. 2013 Jan;91(1):268-75. doi: 10.2527/jas.2012-5341[↩]
- Vajdovich P, Gaál T, Szilágyi A, Harnos A. Changes in some red blood cell and clinical laboratory parameters in young and old Beagle dogs. Vet Res Commun. 1997 Oct;21(7):463-70. doi: 10.1023/a:1005929801735[↩]
- Ferguson G, Bridge W. Glutamate cysteine ligase and the age-related decline in cellular glutathione: The therapeutic potential of γ-glutamylcysteine. Arch Biochem Biophys. 2016 Mar 1;593:12-23. doi: 10.1016/j.abb.2016.01.017[↩]
- Gil L, Siems W, Mazurek B, Gross J, Schroeder P, Voss P, Grune T. Age-associated analysis of oxidative stress parameters in human plasma and erythrocytes. Free Radic Res. 2006 May;40(5):495-505. doi: 10.1080/10715760600592962[↩]
- Lang CA, Naryshkin S, Schneider DL, Mills BJ, Lindeman RD. Low blood glutathione levels in healthy aging adults. J Lab Clin Med. 1992 Nov;120(5):720-5.[↩]
- Rizvi SI, Maurya PK. Markers of oxidative stress in erythrocytes during aging in humans. Ann N Y Acad Sci. 2007 Apr;1100:373-82. doi: 10.1196/annals.1395.041[↩]
- Sekhar RV, Patel SG, Guthikonda AP, Reid M, Balasubramanyam A, Taffet GE, Jahoor F. Deficient synthesis of glutathione underlies oxidative stress in aging and can be corrected by dietary cysteine and glycine supplementation. Am J Clin Nutr. 2011 Sep;94(3):847-53. doi: 10.3945/ajcn.110.003483[↩][↩][↩]
- Cannizzo ES, Clement CC, Sahu R, Follo C, Santambrogio L. Oxidative stress, inflamm-aging and immunosenescence. J Proteomics. 2011 Oct 19;74(11):2313-23. doi: 10.1016/j.jprot.2011.06.005[↩]
- Lepetsos P, Papavassiliou AG. ROS/oxidative stress signaling in osteoarthritis. Biochim Biophys Acta. 2016 Apr;1862(4):576-591. doi: 10.1016/j.bbadis.2016.01.003[↩]
- Rains JL, Jain SK. Oxidative stress, insulin signaling, and diabetes. Free Radic Biol Med. 2011 Mar 1;50(5):567-75. doi: 10.1016/j.freeradbiomed.2010.12.006[↩]
- Kumar P, Liu C, Suliburk J, Hsu JW, Muthupillai R, Jahoor F, Minard CG, Taffet GE, Sekhar RV. Supplementing Glycine and N-Acetylcysteine (GlyNAC) in Older Adults Improves Glutathione Deficiency, Oxidative Stress, Mitochondrial Dysfunction, Inflammation, Physical Function, and Aging Hallmarks: A Randomized Clinical Trial. J Gerontol A Biol Sci Med Sci. 2023 Jan 26;78(1):75-89. doi: 10.1093/gerona/glac135[↩]
- Nguyen D, Hsu JW, Jahoor F, Sekhar RV. Effect of increasing glutathione with cysteine and glycine supplementation on mitochondrial fuel oxidation, insulin sensitivity, and body composition in older HIV-infected patients. J Clin Endocrinol Metab. 2014 Jan;99(1):169-77. doi: 10.1210/jc.2013-2376[↩][↩]
- Pizzorno J. The path ahead: what should we tell our patients about alcohol? Integrative Med Clin J. 2012;11(6):8–11.[↩]
- Buhl R, Vogelmeier C, Critenden M, Hubbard RC, Hoyt RF Jr, Wilson EM, Cantin AM, Crystal RG. Augmentation of glutathione in the fluid lining the epithelium of the lower respiratory tract by directly administering glutathione aerosol. Proc Natl Acad Sci U S A. 1990 Jun;87(11):4063-7. https://www.ncbi.nlm.nih.gov/pmc/articles/PMC54047/pdf/pnas01036-0049.pdf[↩]
- Allen J, Bradley RD. Effects of oral glutathione supplementation on systemic oxidative stress biomarkers in human volunteers. J Altern Complement Med. 2011 Sep;17(9):827-33. doi: 10.1089/acm.2010.0716[↩]
- Kern JK, Geier DA, Adams JB, Garver CR, Audhya T, Geier MR. A clinical trial of glutathione supplementation in autism spectrum disorders. Med Sci Monit. 2011 Dec;17(12):CR677-82. doi: 10.12659/msm.882125[↩][↩]
- Pendyala L, Creaven PJ. Pharmacokinetic and pharmacodynamic studies of N-acetylcysteine, a potential chemopreventive agent during a phase I trial. Cancer Epidemiol Biomarkers Prev. 1995 Apr-May;4(3):245-51.[↩]
- Jannatifar R., Parivar K., Roodbari N.H., Nasr-Esfahani M.H. Effects of N-acetyl-cysteine supplementation on sperm quality, chromatin integrity and level of oxidative stress in infertile men. Reprod Biol. Endocrinol. 2019;17:24. doi: 10.1186/s12958-019-0468-9[↩][↩]
- Zhang Q., Ju Y., Ma Y., Wang T. N-acetylcysteine improves oxidative stress and inflammatory response in patients with community acquired pneumonia: A randomized controlled trial. Medicine. 2018;97:e13087. doi: 10.1097/MD.0000000000013087[↩][↩]
- Rushworth G.F., Megson I.L. Existing and potential therapeutic uses for N-acetylcysteine: the need for conversion to intracellular glutathione for antioxidant benefits. Pharmacol. Ther. 2014;141:150–159. doi: 10.1016/j.pharmthera.2013.09.006[↩][↩]
- Ellegaard P.K., Licht R.W., Nielsen R.E., Dean O.M., Berk M., Poulsen H.E., Mohebbi M., Nielsen C.T. The efficacy of adjunctive N-acetylcysteine in acute bipolar depression: A randomized placebo-controlled study. J. Affect. Disord. 2019;245:1043–1051. doi: 10.1016/j.jad.2018.10.083[↩][↩]
- Berk M., Turner A., Malhi G.S., Ng C.H., Cotton S.M., Dodd S., Samuni Y., Tanious M., McAulay C., Dowling N., et al. A randomised controlled trial of a mitochondrial therapeutic target for bipolar depression: mitochondrial agents, N-acetylcysteine, and placebo. BMC Med. 2019;17:18. doi: 10.1186/s12916-019-1257-1[↩][↩]
- Panizzutti B., Bortolasci C., Hasebe K., Kidnapillai S., Gray L., Walder K., Berk M., Mohebbi M., Dodd S., Gama C., et al. Mediator effects of parameters of inflammation and neurogenesis from a N-acetyl cysteine clinical-trial for bipolar depression. Acta Neuropsychiatr. 2018;30:334–341. doi: 10.1017/neu.2018.13[↩][↩]
- Coles L.D., Tuite P.J., Öz G., Mishra U.R., Kartha R.V., Sullivan K.M., Cloyd J.C., Terpstra M. Repeated-dose oral N-Acetylcysteine in Parkinson’s disease: Pharmacokinetics and effect on brain glutathione and oxidative stress. J. Clin. Pharmacol. 2018;58:158–167. doi: 10.1002/jcph.1008[↩][↩][↩][↩][↩][↩]
- Holmay M.J., Terpstra M., Coles L.D., Mishra U., Ahlskog M., Oz G., Cloyd J.C., Tuite P.J. N-Acetylcysteine boosts brain and blood glutathione in Gaucher and Parkinson diseases. Clin. Neuropharmacol. 2013;36:103–106. doi: 10.1097/WNF.0b013e31829ae713[↩][↩][↩]
- Wink L.K., Adams R., Wang Z., Klaunig J.E., Plawecki M.H., Posey D.J., McDpugle C.J., Erickson C.A. A randomized placebo-controlled pilot study of N-acetylcysteine in youth with autism spectrum disorder. Mol. Autism. 2016;7:26. doi: 10.1186/s13229-016-0088-6[↩][↩]
- Lin C.Y., Wu J.L., Shih T.S., Tsai P.J., Sun Y.M., Ma M.C., Guo Y.L. N-Acetyl-cysteine against noise-induced temporary threshold shift in male workers. Hear Res. 2010;269:42–47. doi: 10.1016/j.heares.2010.07.005[↩][↩][↩]
- Lieber CS, Packer L. S-Adenosylmethionine: molecular, biological, and clinical aspects–an introduction. Am J Clin Nutr. 2002 Nov;76(5):1148S-50S. doi: 10.1093/ajcn/76/5.1148S[↩]
- Pamuk GE, Sonsuz A. N-acetylcysteine in the treatment of non-alcoholic steatohepatitis. J Gastroenterol Hepatol. 2003 Oct;18(10):1220-1. doi: 10.1046/j.1440-1746.2003.03156.x[↩]
- Martínez Alvarez JR, Bellés VV, López-Jaén AB, Marín AV, Codoñer-Franch P. Effects of alcohol-free beer on lipid profile and parameters of oxidative stress and inflammation in elderly women. Nutrition. 2009 Feb;25(2):182-7. doi: 10.1016/j.nut.2008.08.005[↩]
- Li N, Jia X, Chen CY, Blumberg JB, Song Y, Zhang W, Zhang X, Ma G, Chen J. Almond consumption reduces oxidative DNA damage and lipid peroxidation in male smokers. J Nutr. 2007 Dec;137(12):2717-22. doi: 10.1093/jn/137.12.2717[↩]
- Sharma H, Datta P, Singh A, Sen S, Bhardwaj NK, Kochupillai V, Singh N. Gene expression profiling in practitioners of Sudarshan Kriya. J Psychosom Res. 2008 Feb;64(2):213-8. doi: 10.1016/j.jpsychores.2007.07.003[↩]
- Franco R., Schoneveld O.J., Pappa A., Panayiotidis M.I. The central role of glutathione in the pathophysiology of human diseases. Arch. Physiol. Biochem. 2007;113:234–258. doi: 10.1080/13813450701661198[↩]
- Aoyama K., Nakaki T. Impaired glutathione synthesis in neurodegeneration. Int. J. Mol. Sci. 2013;14:21021–21044. doi: 10.3390/ijms141021021[↩]
- Saharan S., Mandal P.K. The emerging role of glutathione in Alzheimer’s disease. J. Alzheimers Dis. 2014;40:519–529. doi: 10.3233/JAD-132483[↩]
- Rae C.D., Williams S.R. Glutathione in the human brain: Review of its roles and measurement by magnetic resonance spectroscopy. Anal. Biochem. 2017;529:127–143. doi: 10.1016/j.ab.2016.12.022[↩]
- Carvalho A.N., Lim J.L., Nijland P.G., Witte M.E., Van Horssen J. Glutathione in multiple sclerosis: More than just an antioxidant? Mult. Scler. 2014;20:1425–1431. doi: 10.1177/1352458514533400[↩]
- Nucifora L.G., Tanaka T., Hayes L.N., Kim M., Lee B.J., Matsuda T., Nucifora F.C., Jr., Sedlak T., Mojtabai R., Eaton W., et al. Reduction of plasma glutathione in psychosis associated with schizophrenia and bipolar disorder in translational psychiatry. Transl. Psychiatry. 2017;7:e1215. doi: 10.1038/tp.2017.178[↩]
- Nguyen D., Hsu J.W., Jahoor F., Sekhar R.V. Effect of increasing glutathione with cysteine and glycine supplementation on mitochondrial fuel oxidation, insulin sensitivity, and body composition in older HIV-infected patients. J. Clin. Endocrinol. Metab. 2014;99:169–177. doi: 10.1210/jc.2013-2376[↩]
- Robaczewska J, Kedziora-Kornatowska K, Kozakiewicz M, Zary-Sikorska E, Pawluk H, Pawliszak W, Kedziora J. Role of glutathione metabolism and glutathione-related antioxidant defense systems in hypertension. J Physiol Pharmacol. 2016 Jun;67(3):331-7.[↩]
- Czuczejko J, Zachara BA, Staubach-Topczewska E, Halota W, Kedziora J. Selenium, glutathione and glutathione peroxidases in blood of patients with chronic liver diseases. Acta Biochim Pol. 2003;50(4):1147-54.[↩]
- Achari A.E., Jain S.K. l-Cysteine supplementation increases insulin sensitivity mediated by upregulation of GSH and adiponectin in high glucose treated 3T3-L1 adipocytes. Arch. Biochem. Biophys. 2017;630:54–65. doi: 10.1016/j.abb.2017.07.016[↩]
- Sekhar R.V., McKay S.V., Patel S.G., Guthikonda A.P., Reddy V.T., Balasubramanyam A., Jahoor F. Glutathione synthesis is diminished in patients with uncontrolled diabetes and restored by dietary supplementation with cysteine and glycine. Diabetes Care. 2011;34:162–167. doi: 10.2337/dc10-1006[↩]
- Kettle A.J., Turner R., Gangell C.L., Harwood D.T., Khalilova I.S., Chapman A.L., Winterbourn C.C., Sly P.D., Arest C.F. Oxidation contributes to low glutathione in the airways of children with cystic fibrosis. Eur. Respir. J. 2014;44:122–129. doi: 10.1183/09031936.00170213[↩]
- Lang C.A., Mills B.J., Lang H.L., Liu M.C., Usui W.M., Richie J., Jr., Mastropaolo W., Murrell S.A. High blood glutathione levels accompany excellent physical and mental health in women ages 60 to 103 years. J Lab. Clin. Med. 2002;140:413–417. doi: 10.1067/mlc.2002.129504[↩]
- Julius M, Lang CA, Gleiberman L, Harburg E, DiFranceisco W, Schork A. Glutathione and morbidity in a community-based sample of elderly. J Clin Epidemiol. 1994 Sep;47(9):1021-6. doi: 10.1016/0895-4356(94)90117-1[↩]
- Borrás C, Esteve JM, Viña JR, Sastre J, Viña J, Pallardó FV. Glutathione regulates telomerase activity in 3T3 fibroblasts. J Biol Chem. 2004 Aug 13;279(33):34332-5. doi: 10.1074/jbc.M402425200[↩]
- Wei YH, Ma YS, Lee HC, Lee CF, Lu CY. Mitochondrial theory of aging matures–roles of mtDNA mutation and oxidative stress in human aging. Zhonghua Yi Xue Za Zhi (Taipei). 2001 May;64(5):259-70.[↩]
- Barja G, Herrero A. Oxidative damage to mitochondrial DNA is inversely related to maximum life span in the heart and brain of mammals. FASEB J. 2000 Feb;14(2):312-8. doi: 10.1096/fasebj.14.2.312[↩]
- Traverso N., Ricciarelli R., Nitti M., Marengo B., Furfaro A.L., Pronzato M.A., Marinari U.M., Domenicotti C. Role of glutathione in cancer progression and chemoresistance. Oxid. Med. Cell Longev. 2013;2013:972913. doi: 10.1155/2013/972913[↩]
- Adeoye O., Olawumi J., Opeyemi A., Christiania O. Review on the role of glutathione on oxidative stress and infertility. JBRA Assist. Reprod. 2018;22:61–66. doi: 10.5935/1518-0557.20180003[↩]
- Shah D., Sah S., Nath S.K. Interaction between glutathione and apoptosis in systemic lupus erythematosus. Autoimmun. Rev. 2013;12:741–751. doi: 10.1016/j.autrev.2012.12.007[↩]
- Hauser RA, Lyons KE, McClain T, Carter S, Perlmutter D. Randomized, double-blind, pilot evaluation of intravenous glutathione in Parkinson’s disease. Mov Disord. 2009 May 15;24(7):979-83. doi: 10.1002/mds.22401[↩]
- Arosio E, De Marchi S, Zannoni M, Prior M, Lechi A. Effect of glutathione infusion on leg arterial circulation, cutaneous microcirculation, and pain-free walking distance in patients with peripheral obstructive arterial disease: a randomized, double-blind, placebo-controlled trial. Mayo Clin Proc. 2002 Aug;77(8):754-9. doi: 10.4065/77.8.754[↩]
- Bishop C, Hudson VM, Hilton SC, Wilde C. A pilot study of the effect of inhaled buffered reduced glutathione on the clinical status of patients with cystic fibrosis. Chest. 2005 Jan;127(1):308-17. doi: 10.1378/chest.127.1.308[↩]
- Stav D, Raz M. Effect of N-acetylcysteine on air trapping in COPD: a randomized placebo-controlled study. Chest. 2009 Aug;136(2):381-386. doi: 10.1378/chest.09-0421[↩]
- Cooke RW, Drury JA. Reduction of oxidative stress marker in lung fluid of preterm infants after administration of intra-tracheal liposomal glutathione. Biol Neonate. 2005;87(3):178-80. doi: 10.1159/000082623[↩]
- Saitoh T, Satoh H, Nobuhara M, Machii M, Tanaka T, Ohtani H, Saotome M, Urushida T, Katoh H, Hayashi H. Intravenous glutathione prevents renal oxidative stress after coronary angiography more effectively than oral N-acetylcysteine. Heart Vessels. 2011 Sep;26(5):465-72. doi: 10.1007/s00380-010-0078-0[↩]
- Testa B, Testa D, Mesolella M, D’Errico G, Tricarico D, Motta G. Management of chronic otitis media with effusion: the role of glutathione. Laryngoscope. 2001 Aug;111(8):1486-9. doi: 10.1097/00005537-200108000-00028[↩]
- Kasperczyk S, Dobrakowski M, Kasperczyk A, Ostałowska A, Birkner E. The administration of N-acetylcysteine reduces oxidative stress and regulates glutathione metabolism in the blood cells of workers exposed to lead. Clin Toxicol (Phila). 2013 Jul;51(6):480-6. doi: 10.3109/15563650.2013.802797[↩]
- Ghanizadeh A, Derakhshan N, Berk M. N-acetylcysteine versus placebo for treating nail biting, a double blind randomized placebo controlled clinical trial. Antiinflamm Antiallergy Agents Med Chem. 2013;12(3):223-8. doi: 10.2174/1871523011312030003[↩]
- Medved I, Brown MJ, Bjorksten AR, Murphy KT, Petersen AC, Sostaric S, Gong X, McKenna MJ. N-acetylcysteine enhances muscle cysteine and glutathione availability and attenuates fatigue during prolonged exercise in endurance-trained individuals. J Appl Physiol (1985). 2004 Oct;97(4):1477-85. doi: 10.1152/japplphysiol.00371.2004[↩]
- Ulrich K., Jakob U. The role of thiols in antioxidant systems. Free Radic. Biol. Med. 2019;140:14–27. doi: 10.1016/j.freeradbiomed.2019.05.035[↩]
- Biswas SK, Rahman I. Environmental toxicity, redox signaling and lung inflammation: the role of glutathione. Mol Aspects Med. 2009 Feb-Apr;30(1-2):60-76. doi: 10.1016/j.mam.2008.07.001[↩]
- Lenton K.J., Therriault H., Wagner J.R. Analysis of glutathione and glutathione disulfide in whole cells and mitochondria by postcolumn derivatization high-performance liquid chromatography with ortho-phthalaldehyde. Anal. Biochem. 1999;274:125–130. doi: 10.1006/abio.1999.4258[↩]
- Meister A. Glutathione metabolism and its selective modification. J. Biol. Chem. 1988;263:17205–17208. doi: 10.1016/S0021-9258(19)77815-6[↩]
- Josephy P.D., Mannervik B. Molecular Toxicology. 2nd ed. Oxford University Press, Inc.; New York, NY, USA: 2006. Biochemistry of Glutathione; pp. 333–364.[↩]
- Townsend D.M., Tew K.D., Tapiero H. The importance of glutathione in human disease. Biomed. Pharmacother. 2003;57:145–155. doi: 10.1016/S0753-3322(03)00043-X[↩]
- Grey V, Mohammed SR, Smountas AA, Bahlool R, Lands LC. Improved glutathione status in young adult patients with cystic fibrosis supplemented with whey protein. J Cyst Fibros. 2003 Dec;2(4):195-8. doi: 10.1016/S1569-1993(03)00097-3. Erratum in: J Cyst Fibros. 2004 Mar;3(1):62.[↩]
- Atwal PS, Medina CR, Burrage LC, Sutton VR. Nineteen-year follow-up of a patient with severe glutathione synthetase deficiency. J Hum Genet. 2016 Jul;61(7):669-72. doi: 10.1038/jhg.2016.20[↩][↩][↩]
- National Center for Biotechnology Information (2023). PubChem Compound Summary for CID 124886, Glutathione. https://pubchem.ncbi.nlm.nih.gov/compound/Glutathione[↩][↩][↩]
- Al-Jishi E, Meyer BF, Rashed MS, Al-Essa M, Al-Hamed MH, Sakati N, et al. Clinical, biochemical, and molecular characterization of patients with glutathione synthetase deficiency. Clin Genet. 1999;55:444–449. doi: 10.1034/j.1399-0004.1999.550608.x[↩]
- Glutathione synthetase deficiency. https://rarediseases.info.nih.gov/diseases/10047/glutathione-synthetase-deficiency[↩][↩][↩]
- Xia H, Ye J, Wang L, Zhu J, He Z. A case of severe glutathione synthetase deficiency with novel GSS mutations. Braz J Med Biol Res. 2018 Jan 11;51(3):e6853. doi: 10.1590/1414-431X20176853[↩]
- Larsson A, Ristoff E, Anderson ME. Metabolic and molecular bases of inherited disease. In: Scriver CR, Beaudet AL, Sly WS, Valle D, editors. Metabolic and Molecular Bases of Inherited Disease. McGraw-Hill; New York: 2005.[↩][↩]
- Ristoff E, Mayatepek E, Larsson A. Long-term clinical outcome in patients with glutathione synthetase deficiency. J Pediatr. 2001 Jul;139(1):79-84. doi: 10.1067/mpd.2001.114480[↩][↩][↩][↩]
- Al-Jishi E, Meyer BF, Rashed MS, Al-Essa M, Al-Hamed MH, Sakati N, et al. Clinical, biochemical, and molecular characterization of patients with glutathione synthetase deficiency. Clin Genet. 1999;55:444–449. doi: 10.1034/j.1399-0004.1999.550608.x[↩]
- Njalsson R. Glutathione synthetase deficiency. Cell Mol Life Sci. 2005;62:1938–1945. doi: 10.1007/s00018-005-5163-7[↩]
- Larsson A, Ristoff E, Anderson ME. Glutathione synthetase deficiency and other disorders of the gamma-glutamyl cycle. In: Scriver CR, Beaudet AL, Sly WS, Valle D, Vogelstein B, Childs B, et al., editors. The metabolic and molecular bases of inherited disease. New York: McGraw-Hill; 2005.[↩]
- Erasmus, E., Mienie, L.J., de Vries, W.N., de Wet, W.J., Carlsson, B. and Larsson, A. (1993), Prenatal analysis in two suspected cases of glutathione synthetase deficiency. J Inherit Metab Dis, 16: 837-843. https://doi.org/10.1007/BF00714275[↩]
- Manning, N.J., Davies, N.P., Olpin, S.E., Carpenter, K.H., Smith, M.F., Pollitt, R.J., Duncan, S.L.B., Larsson, A. and Carlsson, B. (1994), Prenatal diagnosis of glutathione synthase deficiency. Prenat. Diagn., 14: 475-478. https://doi.org/10.1002/pd.1970140611[↩]
- Simon, E., Vogel, M., Fingerhut, R., Ristoff, E., Mayatepek, E. and Spiekerkötter, U. (2009), Diagnosis of glutathione synthetase deficiency in newborn screening. J Inherit Metab Dis, 32: 269-272. https://doi.org/10.1007/s10545-009-1213-x[↩]
- Ben Ameur S, Aloulou H, Nasrallah F, Kamoun T, Kaabachi N, Hachicha M. Hemolytic anemia and metabolic acidosis: think about glutathione synthetase deficiency. Fetal Pediatr Pathol. 2015 Feb;34(1):18-20. doi: 10.3109/15513815.2014.947543[↩]
- Spielberg SP, Boxer LA, Corash LM, Schulman JD. Improved erythrocyte survival with high-dose vitamin E in chronic hemolyzing G6PD and glutathione synthetase deficiencies. Ann Intern Med. 1979 Jan;90(1):53-4. doi: 10.7326/0003-4819-90-1-53[↩]
- Njålsson R, Ristoff E, Carlsson K, Winkler A, Larsson A, Norgren S. Genotype, enzyme activity, glutathione level, and clinical phenotype in patients with glutathione synthetase deficiency. Hum Genet. 2005 Apr;116(5):384-9. doi: 10.1007/s00439-005-1255-6[↩][↩]
- Tinggi U. Selenium: its role as antioxidant in human health. Environ Health Prev Med. 2008 Mar;13(2):102-8. doi: 10.1007/s12199-007-0019-4[↩]
- Pejaver, R.K. and Watson, A.H. (1992), 5-Oxoprolinuria due to glutathione synthetase deficiency. J Inherit Metab Dis, 15: 937-938. https://doi.org/10.1007/BF01800239[↩]
- Boxer LA, Oliver JM, Spielberg SP, Allen JM, Schulman JD. Protection of granulocytes by vitamin E in glutathione synthetase deficiency. N Engl J Med. 1979 Oct 25;301(17):901-5. doi: 10.1056/NEJM197910253011702[↩]
- Pejaver, R.K. and Watson, A.H. (1994), High-dose vitamin E therapy in glutathione synthetase deficiency. J Inherit Metab Dis, 17: 749-750. https://doi.org/10.1007/BF00712019[↩]
- Potęga A. Glutathione-Mediated Conjugation of Anticancer Drugs: An Overview of Reaction Mechanisms and Biological Significance for Drug Detoxification and Bioactivation. Molecules. 2022 Aug 17;27(16):5252. doi: 10.3390/molecules27165252[↩]
- Hall MN, Niedzwiecki M, Liu X, Harper KN, Alam S, Slavkovich V, Ilievski V, Levy D, Siddique AB, Parvez F, Mey JL, van Geen A, Graziano J, Gamble MV. Chronic arsenic exposure and blood glutathione and glutathione disulfide concentrations in Bangladeshi adults. Environ Health Perspect. 2013 Sep;121(9):1068-74. doi: 10.1289/ehp.1205727[↩]
- Sonthalia S, Daulatabad D, Sarkar R. Glutathione as a skin whitening agent: Facts, myths, evidence and controversies. Indian J Dermatol Venereol Leprol. 2016 May-Jun;82(3):262-72. https://ijdvl.com/glutathione-as-a-skin-whitening-agent-facts-myths-evidence-and-controversies/[↩]
- Jariwalla RJ, Lalezari J, Cenko D, Mansour SE, Kumar A, Gangapurkar B, Nakamura D. Restoration of blood total glutathione status and lymphocyte function following alpha-lipoic acid supplementation in patients with HIV infection. J Altern Complement Med. 2008 Mar;14(2):139-46. doi: 10.1089/acm.2006.6397[↩]
- Gupta S.C., Patchva S., Aggarwal B.B. Therapeutic roles of curcumin: lessons learned from clinical trials. AAPS J. 2013;15:195–218. doi: 10.1208/s12248-012-9432-8[↩]
- Sinha R., Sinha I., Calcagnotto A., Trushin N., Haley J.S., Schell T.D., Richie J.P., Jr. Oral supplementation with liposomal glutathione elevates body stores of glutathione and markers of immune function. Eur. J. Clin. Nutr. 2018;72:105–111. doi: 10.1038/ejcn.2017.132[↩]
- Park E.Y., Shimura N., Konishi T., Sauchi Y., Wada S., Aoi W., Nakamura Y., Sato K. Increase in the protein-bound form of glutathione in human blood after the oral administration of glutathione. J. Agric. Food Chem. 2014;62:6183–6189. doi: 10.1021/jf501338z[↩]
- Richie J.P., Jr., Nichenametla S., Neidig W., Calcagnotto A., Haley J.S., Schell T.D., Muscat J.E. Randomized controlled trial of oral glutathione supplementation on body stores of glutathione. Eur. J. Nutr. 2015;54:251–263. doi: 10.1007/s00394-014-0706-z[↩]
- Sekhar R.V., Patel S.G., Guthikonda A.P., Reid M., Balasubramanyam A., Taffet G.E., Jahoor F. Deficient synthesis of glutathione underlies oxidative stress in aging and can be corrected by dietary cysteine and glycine supplementation. Am. J. Clin. Nutr. 2011;94:847–853. doi: 10.3945/ajcn.110.003483[↩][↩][↩][↩][↩][↩]
- Gaby A. 51: N-Acetylcysteine. In: Concord N.H., editor. Nutritional Medicine. Fritz Perlberg Publishing; Concord, NH, USA: 2011.[↩][↩]
- Duffy S.L., Lagopoulos J., Cockayne N., Lewis S.J., Hickie I.B., Hermens D.F., Naismith S.L. The effect of 12-wk ω-3 fatty acid supplementation on in vivo thalamus glutathione concentration in patients “at risk” for major depression. Nutrition. 2015;31:1247–1254. doi: 10.1016/j.nut.2015.04.019[↩]
- García-Rodríguez C.E., Mesa M.D., Olza J., Vlachava M., Kremmyda L.S., Diaper N.D., Noakes P.S., Miles E.A., Ramírez-Tortosa M.C., Liaset B., et al. Does consumption of two portions of salmon per week enhance the antioxidant defense system in pregnant women? Antioxid. Redox. Signal. 2012;16:1401–1406. doi: 10.1089/ars.2012.4508[↩]
- Richie J.P., Jr., Muscat J.E., Ellison I., Calcagnotto A., Kleinman W., El-Bayoumy K. Association of selenium status and blood glutathione concentrations in blacks and whites. Nutr. Cancer. 2011;63:367–375. doi: 10.1080/01635581.2011.535967[↩]
- Lenton K.J., Sané A.T., Therriault H., Cantin A.M., Payette H., Wagner J.R. Vitamin C augments lymphocyte glutathione in subjects with ascorbate deficiency. Am. J. Clin. Nutr. 2003;77:189–195. doi: 10.1093/ajcn/77.1.189[↩]
- Johnston C.S., Meyer C.G., Srilakshmi J.C. Vitamin C elevates red blood cell glutathione in healthy adults. Am. J. Clin. Nutr. 1993;58:103–105. doi: 10.1093/ajcn/58.1.103[↩]
- Taghizadeh M., Tamtaji O.R., Dadgostar E., Daneshvar Kakhaki R., Bahmani F., Abolhassani J., Aarabi M.H., Kouchaki E., Memarzadeh M.R., Asemi Z. The effects of omega-3 fatty acids and vitamin E co-supplementation on clinical and metabolic status in patients with Parkinson’s disease: A randomized, double-blind, placebo-controlled trial. Neurochem. Int. 2017;108:183–189. doi: 10.1016/j.neuint.2017.03.014[↩]
- Jain S.K., McVie R., Smith T. Vitamin E supplementation restores glutathione and malondialdehyde to normal concentrations in erythrocytes of type 1 diabetic children. Diabetes Care. 2000;23:1389–1394. doi: 10.2337/diacare.23.9.1389[↩]
- Bumrungpert A., Pavadhgul P., Nunthanawanich P., Sirikanchanarod A., Adulbhan A. Whey protein supplementation improves nutritional status, glutathione levels, and immune function in cancer patients: A randomized, double-blind controlled trial. J Med. Food. 2018;21:612–616. doi: 10.1089/jmf.2017.4080[↩]
- Terao J. Potential Role of Quercetin Glycosides as Anti-Atherosclerotic Food-Derived Factors for Human Health. Antioxidants. 2023;12:258. doi: 10.3390/antiox12020258[↩]
- Georgiou N, Kakava MG, Routsi EA, Petsas E, Stavridis N, Freris C, Zoupanou N, Moschovou K, Kiriakidi S, Mavromoustakos T. Quercetin: A Potential Polydynamic Drug. Molecules. 2023 Dec 17;28(24):8141. doi: 10.3390/molecules28248141[↩]
- Cui Z, Zhao X, Amevor FK, Du X, Wang Y, Li D, Shu G, Tian Y, Zhao X. Therapeutic application of quercetin in aging-related diseases: SIRT1 as a potential mechanism. Front Immunol. 2022 Jul 22;13:943321. doi: 10.3389/fimmu.2022.943321[↩]
- Lotfi N, Yousefi Z, Golabi M, Khalilian P, Ghezelbash B, Montazeri M, Shams MH, Baghbadorani PZ, Eskandari N. The potential anti-cancer effects of quercetin on blood, prostate and lung cancers: An update. Front Immunol. 2023 Feb 28;14:1077531. doi: 10.3389/fimmu.2023.1077531[↩]
- Zhang X, Tang Y, Lu G, Gu J. Pharmacological Activity of Flavonoid Quercetin and Its Therapeutic Potential in Testicular Injury. Nutrients. 2023 May 8;15(9):2231. doi: 10.3390/nu15092231[↩]
- Carrillo-Martinez EJ, Flores-Hernández FY, Salazar-Montes AM, Nario-Chaidez HF, Hernández-Ortega LD. Quercetin, a Flavonoid with Great Pharmacological Capacity. Molecules. 2024 Feb 25;29(5):1000. doi: 10.3390/molecules29051000[↩][↩][↩][↩]
- Hosseini A., Razavi B.M., Banach M., Hosseinzadeh H. Quercetin and metabolic syndrome: A review. Phytother. Res. PTR. 2021;35:5352–5364. doi: 10.1002/ptr.7144[↩]
- Shen P., Lin W., Deng X., Ba X., Han L., Chen Z., Qin K., Huang Y., Tu S. Potential Implications of Quercetin in Autoimmune Diseases. Front. Immunol. 2021;12:689044. doi: 10.3389/fimmu.2021.689044[↩]
- Endothelial function and cardiovascular disease: effects of quercetin and wine polyphenols. Free Radic Res. 2006 Oct;40(10):1054-65. https://www.ncbi.nlm.nih.gov/pubmed/17015250[↩]
- Committee of Pharmacognosy Publication. Pharmacognosy. Pharmacognosy. Seoul Korea: Dong Myoung; 2013. pp. 102–105.[↩]
- Li Y., Yao J., Han C., Yang J., Chaudhry M.T., Wang S., Liu H., Yin Y. Quercetin, Inflammation and Immunity. Nutrients. 2016;8:167. doi: 10.3390/nu8030167[↩]
- Mutha R.E., Tatiya A.U., Surana S.J. Flavonoids as natural phenolic compounds and their role in therapeutics: An overview. Futur. J. Pharm. Sci. 2021;7:25. doi: 10.1186/s43094-020-00161-8[↩]
- Roy A., Khan A., Ahmad I., Alghamdi S., Rajab B.S., Babalghith A.O., Alshahrani M.Y., Islam S., Islam M.R. Flavonoids a Bioactive Compound from Medicinal Plants and Its Therapeutic Applications. Biomed Res. Int. 2022;2022:5445291. doi: 10.1155/2022/5445291[↩]
- Tronina T., Łużny M., Dymarska M., Urbaniak M., Kozłowska E., Piegza M., Stępień Ł., Janeczko T. Glycosylation of Quercetin by Selected Entomopathogenic Filamentous Fungi and Prediction of Its Products’ Bioactivity. Int. J. Mol. Sci. 2023;24:11857. doi: 10.3390/ijms241411857[↩]
- Shorobi F.M., Nisa F.Y., Saha S., Chowdhury M.A.H., Srisuphanunt M., Hossain K.H., Rahman M.A. Quercetin: A Functional Food-Flavonoid Incredibly Attenuates Emerging and Re-Emerging Viral Infections through Immunomodulatory Actions. Molecules. 2023;28:938. doi: 10.3390/molecules28030938[↩]
- Marrelli M., Russo C., Statti G., Argentieri M.P., Meleleo D., Mallamaci R., Avato P., Conforti F. Phytochemical and biological characterization of dry outer scales extract from Tropea red onion (Allium cepa L. var. Tropea)–A promising inhibitor of pancreatic lipase. Phytomed. Plus. 2022;2:100235. doi: 10.1016/j.phyplu.2022.100235[↩]
- Intake of potentially anticarcinogenic flavonoids and their determinants in adults in The Netherlands. Hertog MG, Hollman PC, Katan MB, Kromhout D. Nutr Cancer. 1993; 20(1):21-9. https://www.ncbi.nlm.nih.gov/pubmed/8415127/[↩]
- Effects of the dietary flavonoid quercetin upon performance and health. Davis JM, Murphy EA, Carmichael MD. Curr Sports Med Rep. 2009 Jul-Aug; 8(4):206-13. https://www.ncbi.nlm.nih.gov/pubmed/19584608/[↩]
- Aguirre L., Arias N., Macarulla M.T., Gracia A., Portillo M.P. Beneficial effects of quercetin on obesity and diabetes. Open Nutraceuticals J. 2011;4:189–198.[↩]
- National Toxicology Program (1991) NTP Chemical Repository Data Sheet: Quercetin , Research Triangle Park, NC.[↩]
- Middleton E Jr, Kandaswami C, Theoharides TC. The effects of plant flavonoids on mammalian cells: implications for inflammation, heart disease and cancer. Pharmacol Rev 2000;52:673–751.[↩]
- Beecher GR. Overview of dietary flavonoids: nomenclature, occurrence and intake. J Nutr 2003;133:3248S–54S.[↩]
- Del Rio D, Rodriguez-Mateos A, Spencer JP, Tognolini M, Borges G, Crozier A. Dietary (poly)phenolics in human health: structures, bioavailability, and evidence of protective effects against chronic diseases. Antioxid Redox Signal 2013;18:1818–92.[↩][↩]
- Russo M, Spagnuolo C, Tedesco I, Bilotto S, Russo GL. The flavonoid quercetin in disease prevention and therapy: facts and fancies. Biochem Pharmacol 2012;83:6–15.[↩][↩][↩]
- Roskosko R Jr. ERK1/2 MAP kinases: structure, function and regulation. Pharmacol Res 2012;66:105–43.[↩]
- Gutiérrez-Venegas G, Bando-Campos CG. The flavonoids luteolin and quercetagetin inhibit lipoteichoic acid actions on H9c2 cardiomyocytes. Int Immunopharmacol 2010;10:1003–9.[↩]
- Walker EH, Pacold ME, Perisic O, Stephens L, Hawkins PT, Wymann MP et al. Structural determinants of phosphoinositide 3-kinase inhibition by wortmannin, LY294002, quercetin, myricetin, and staurosporine. Mol Cell 2000;6:909–19.[↩]
- Hausenloy DJ, Yellon DM. Preconditioning and postconditioning: united at reperfusion. Pharmacol Ther 2007;114:208–21.[↩][↩]
- Shao Z-H, Wojcik KR, Dossumbekova A, Hsu C, Mehendale SR, Li C-Q et al. Grape seed proanthocyanidins protect cardiomyocytes from ischemia and reperfusion injury via Akt-NOS signaling. J Cell Biochem 2009;107:697–705.[↩]
- Angeloni C, Spencer JPE, Leoncini E, Biagi PL, Hrelia S. Role of quercetin and its in vivo metabolites in protecting H9c2 cells against oxidative stress. Biochimie 2007;89:73–82.[↩]
- Sun B, Sun GB, Xiao J, Chen RC, Wang X, Wu Y et al. Isorhamnetin inhibits H2O2-induced activation of the intrinsic apoptotic pathway in H9c2 cardiomyocytes through scavenging reactive oxygen species and ERK inactivation. J Cell Biochem 2012;113:473–85.[↩]
- Nguyen H, Pellegrini MV, Gupta V. Alpha-Lipoic Acid. [Updated 2024 Jan 26]. In: StatPearls [Internet]. Treasure Island (FL): StatPearls Publishing; 2024 Jan-. Available from: https://www.ncbi.nlm.nih.gov/books/NBK564301[↩]
- Alpha-lipoic acid: a multifunctional antioxidant that improves insulin sensitivity in patients with type 2 diabetes. Evans JL, Goldfine ID. Diabetes Technol Ther. 2000 Autumn; 2(3):401-13. https://www.ncbi.nlm.nih.gov/pubmed/11467343/[↩]
- Reed LJ. A trail of research from lipoic acid to alpha-keto acid dehydrogenase complexes. J Biol Chem. 2001 Oct 19;276(42):38329-36. doi: 10.1074/jbc.R100026200[↩]
- Carreau JP. Biosynthesis of lipoic acid via unsaturated fatty acids. Methods Enzymol. 1979;62:152-8. doi: 10.1016/0076-6879(79)62212-7[↩]
- Anthony RM, MacLeay JM, Gross KL. Alpha-Lipoic Acid as a Nutritive Supplement for Humans and Animals: An Overview of Its Use in Dog Food. Animals (Basel). 2021 May 19;11(5):1454. doi: 10.3390/ani11051454[↩][↩][↩]
- Shay KP, Moreau RF, Smith EJ, Smith AR, Hagen TM. Alpha-lipoic acid as a dietary supplement: molecular mechanisms and therapeutic potential. BBA-General Subjects. 2009;1790:1149–1160. doi: 10.1016/j.bbagen.2009.07.026[↩]
- Lee SJ, Kang JG, Ryu OH, Kim CS, Ihm SH, Choi MG, Yoo HJ, Kim DS, Kim TW. Effects of α-lipoic acid on transforming growth factor β1-p38 mitogen-activated protein kinase-fibronectin pathway in diabetic nephropathy. Metabolism. 2009;58:616–623. doi: 10.1016/j.metabol.2008.12.006[↩]
- Hermann R., Niebch G., Borbe H.O., Fieger-Buschges H., Ruus P., Nowak H., Riethmuller H., Peukert M., Blume H. Enantioselective pharmacokinetics and bioavailability of different racemic alpha-lipoic formulations in healthy volunteers. Eur. J. Pharm. Sci. 1996;4:167–174. doi: 10.1016/0928-0987(95)00045-3[↩][↩][↩][↩][↩][↩][↩]
- Uchida R, Okamoto H, Ikuta N, Terao K, Hirota T. Enantioselective Pharmacokinetics of α-Lipoic Acid in Rats. Int J Mol Sci. 2015 Sep 21;16(9):22781-94. doi: 10.3390/ijms160922781[↩][↩]
- Alpha-lipoic acid as a dietary supplement: molecular mechanisms and therapeutic potential. Shay KP, Moreau RF, Smith EJ, Smith AR, Hagen TM. Biochim Biophys Acta. 2009 Oct; 1790(10):1149-60. https://www.ncbi.nlm.nih.gov/pmc/articles/PMC2756298/[↩][↩]
- Packer L, Cadenas E. Lipoic acid: energy metabolism and redox regulation of transcription and cell signaling. J Clin Biochem Nutr. 2011 Jan;48(1):26-32. doi: 10.3164/jcbn.11-005FR[↩]
- Bustamante J, Lodge JK, Marcocci L, Tritschler HJ, Packer L, Rihn BH. Alpha-lipoic acid in liver metabolism and disease. Free Radic Biol Med. 1998 Apr;24(6):1023-39. doi: 10.1016/s0891-5849(97)00371-7[↩]
- Reed L. Multienzyme complexes. Accts Chem Res. 1974;7:40–46.[↩]
- The pharmacology of the antioxidant lipoic acid. Biewenga GP, Haenen GR, Bast A. Gen Pharmacol. 1997 Sep; 29(3):315-31. https://www.ncbi.nlm.nih.gov/pubmed/9378235/[↩]
- Shay KP, Moreau RF, Smith EJ, Smith AR, Hagen TM. Alpha-lipoic acid as a dietary supplement: Molecular mechanisms and therapeutic potential. Biochimica et biophysica acta. 2009;1790(10):1149-1160. doi:10.1016/j.bbagen.2009.07.026. https://www.ncbi.nlm.nih.gov/pmc/articles/PMC2756298/[↩]
- Ross S.M. Clinical applications of lipoic acid in type II diabetes mellitus. Holist. Nurs. Pract. 2006;20:305–306. doi: 10.1097/00004650-200611000-00009[↩]
- Biewenga GP, Haenen GR, Bast A. The pharmacology of the antioxidant lipoic acid. Gen Pharmacol. 1997 Sep;29(3):315-31. doi: 10.1016/s0306-3623(96)00474-0[↩]
- Kramer K, Packer L. R-alpha-lipoic acid. In: Kramer K, Hoppe P, Packer L, eds. Nutraceuticals in Health and Disease Prevention. New York: Marcel Dekker, Inc.; 2001:129-164.[↩]
- Nguyen N, Takemoto JK. A Case for Alpha-Lipoic Acid as an Alternative Treatment for Diabetic Polyneuropathy. J Pharm Pharm Sci. 2018;21(1s):177s-191s. doi: 10.18433/jpps30100[↩]
- Hendler S.S., Rorvik D.R. PDR for Nutritional Supplements. Medical Economics Company, Inc.; Montvale, NJ, USA: 2001.[↩]
- Ziegler D., Ametov A., Barinov A., Dyck P.J., Gurieva I., Low P.A., Munzel U., Yakhno N., Raz I., Novosadova M., et al. Oral treatment with alpha-lipoic acid improves symptomatic diabetic polyneuropathy: The SYDNEY 2 trial. Diabetes Care. 2006;29:2365–2370. doi: 10.2337/dc06-1216[↩][↩]
- Smith A.R., Shenvi S.V., Widlansky M., Suh J.H., Hagen T.M. Lipoic acid as a potential therapy for chronic diseases associated with oxidative stress. Curr. Med. Chem. 2004;11:1135–1146. doi: 10.2174/0929867043365387[↩]
- Teichert J, Kern J, Tritschler HJ, Ulrich H, Preiss R. Investigations on the pharmacokinetics of alpha-lipoic acid in healthy volunteers. Int J Clin Pharmacol Ther. 1998 Dec;36(12):625-8.[↩][↩][↩][↩]
- Bernkop-Schnurch A., Reich-Rohrwig E., Marschutz M., Schuhbauer H., Kratzel M. Development of a sustained release dosage form for alpha-lipoic acid. II. Evaluation in human volunteers. Drug Dev. Ind. Pharm. 2004;30:35–42. doi: 10.1081/DDC-120027509[↩]
- Mignini F., Streccioni V., Tomassoni D., Traini E., Amenta F. Comparative crossover, randomized, open-label bioequivalence study on the bioequivalence of two formulations of thioctic acid in healthy volunteers. Clin. Exp. Hypertens. 2007;29:575–586. doi: 10.1080/10641960701744111[↩]
- Amenta F., Traini E., Tomassoni D., Mignini F. Pharmacokinetics of different formulations of tioctic (alpha-lipoic) acid in healthy volunteers. Clin. Exp. Hypertens. 2008;30:767–775. doi: 10.1080/10641960802563568[↩]
- Ziegler D., Nowak H., Kempler P., Vargha P., Low P.A. Treatment of symptomatic diabetic polyneuropathy with the antioxidant alpha-lipoic acid: A meta-analysis. Diabet. Med. 2004;21:114–121. doi: 10.1111/j.1464-5491.2004.01109.x[↩]
- Ziegler D., Hanefeld M., Ruhnau K.J., Hasche H., Lobisch M., Schutte K., Kerum G., Malessa R. Treatment of symptomatic diabetic polyneuropathy with the antioxidant alpha-lipoic acid: A 7-month multicenter randomized controlled trial (ALADIN III Study). ALADIN III Study Group. Alpha-lipoic acid in diabetic neuropathy. Diabetes Care. 1999;22:1296–1301. doi: 10.2337/diacare.22.8.1296[↩]
- Ziegler D., Low P.A., Litchy W.J., Boulton A.J.M., Vinik A.I., Freeman R., Samigullin R., Tritschler H., Munzel U., Maus J., et al. Efficacy and safety of antioxidant treatment with alpha-lipoic acid over 4 years in diabetic polyneuropathy: The NATHAN 1 trial. Diabetes Care. 2011;34:2054–2060. doi: 10.2337/dc11-0503[↩]
- Yadav V., Marracci G., Lovera J., Woodward W., Bogardus K., Marquardt W., Shinto L., Morris C., Bourdette D. Lipoic acid in multiple sclerosis: A pilot study. Mult. Scler. 2005;11:159–165. doi: 10.1191/1352458505ms1143oa[↩]
- Carlson DA, Smith AR, Fischer SJ, Young KL, Packer L. The plasma pharmacokinetics of R-(+)-lipoic acid administered as sodium R-(+)-lipoate to healthy human subjects. Altern Med Rev. 2007 Dec;12(4):343-51.[↩]
- Gleiter C.H., Schug B.S., Hermann R., Elze M., Blume H.H., Gundert-Remy U. Influence of food intake on the bioavailability of thioctic acid enantiomers. Eur. J. Clin. Pharmacol. 1996;50:513–514. doi: 10.1007/s002280050151[↩]
- Breithaupt-Grogler K., Niebch G., Schneider E., Erb K., Hermann R., Blume H.H., Schug B.S., Belz G.G. Dose-proportionality of oral thioctic acid–coincidence of assessments via pooled plasma and individual data. Eur. J. Pharm. Sci. 1999;8:57–65. doi: 10.1016/S0928-0987(98)00061-X[↩][↩]
- Keith D.J., Butler J.A., Bemer B., Dixon B., Johnson S., Garrard M., Sudakin D.L., Christensen J.M., Pereira C., Hagen T.M. Age and gender dependent bioavailability of R- and R,S-alpha-lipoic acid: A pilot study. Pharmacol. Res. 2012;66:199–206. doi: 10.1016/j.phrs.2012.05.002[↩][↩]
- Reed L.J. A trail of research from lipoic acid to alpha-keto acid dehydrogenase complexes. J. Biol. Chem. 2001;276:38329–38336. doi: 10.1074/jbc.R100026200[↩]
- Golbidi S, Badran M, Laher I. Diabetes and alpha lipoic Acid. Front Pharmacol. 2011 Nov 17;2:69. doi: 10.3389/fphar.2011.00069[↩]
- Petersen Shay K., Moreau R. F., Smith E. J., Hagen T. M. (2008). Is alpha-lipoic acid a scavenger of reactive oxygen species in vivo? Evidence for its initiation of stress signaling pathways that promote endogenous antioxidant capacity. IUBMB Life 60, 362–367 10.1002/iub.40[↩]
- Gorąca A., Huk-Kolega H., Piechota A., Kleniewska P., Ciejka E., Skibska B. Lipoic acid-biological activity and therapeutic potential. Pharm. Rep. 2011;63:849–858. doi: 10.1016/S1734-1140(11)70600-4[↩]
- Biewenga G.P., Haenen G.R., Bast A. The Pharmacology of the Antioxidant Lipoic Acid. Gen. Pharmac. 1997;29:315–331. doi: 10.1016/S0306-3623(96)00474-0[↩]
- Spector A., Huang R.-R.C., Yan G.-Z., Wang R.-R. Thioredoxin fragment 31–36 is reduced by dihydrolipoamide and reduces oxidized protein. Biochem. Biophys. Res. Commun. 1988;150:156–162. doi: 10.1016/0006-291X(88)90499-8[↩]
- Shay KP, Moreau RF, Smith EJ, Smith AR, Hagen TM. Alpha-lipoic acid as a dietary supplement: molecular mechanisms and therapeutic potential. Biochim Biophys Acta. 2009 Oct;1790(10):1149-60. doi: 10.1016/j.bbagen.2009.07.026[↩]
- Park S, Karunakaran U, Jeoung NH, Jeon JH, Lee IK. Physiological effect and therapeutic application of alpha lipoic acid. Curr Med Chem. 2014;21(32):3636-45. doi: 10.2174/0929867321666140706141806[↩]
- Molecular aspects of lipoic acid in the prevention of diabetes complications. Packer L, Kraemer K, Rimbach G. Nutrition. 2001 Oct; 17(10):888-95.[↩]
- Ziegler D, Ametov A, Barinov A, et al. Oral treatment with alpha-lipoic acid improves symptomatic diabetic polyneuropathy: the SYDNEY 2 trial. Diabetes Care. 2006;29:2365–2370.[↩]
- Han T, Bai J, Liu W, Hu Y. A systematic review and meta-analysis of α-lipoic acid in the treatment of diabetic peripheral neuropathy. Eur J Endocrinol. 2012 Oct;167(4):465-71. doi: 10.1530/EJE-12-0555[↩]
- Ziegler D. Thioctic acid for patients with symptomatic diabetic polyneuropathy: a critical review. Treat Endocrinol. 2004;3(3):173-89. doi: 10.2165/00024677-200403030-00005[↩]
- Ruhnau KJ, Meissner HP, Finn JR, Reljanovic M, Lobisch M, Schütte K, Nehrdich D, Tritschler HJ, Mehnert H, Ziegler D. Effects of 3-week oral treatment with the antioxidant thioctic acid (alpha-lipoic acid) in symptomatic diabetic polyneuropathy. Diabet Med. 1999 Dec;16(12):1040-3. doi: 10.1046/j.1464-5491.1999.00190.x[↩]
- Ziegler D, Hanefeld M, Ruhnau KJ, Hasche H, Lobisch M, Schütte K, Kerum G, Malessa R. Treatment of symptomatic diabetic polyneuropathy with the antioxidant alpha-lipoic acid: a 7-month multicenter randomized controlled trial (ALADIN III Study). ALADIN III Study Group. Alpha-Lipoic Acid in Diabetic Neuropathy. Diabetes Care. 1999 Aug;22(8):1296-301. doi: 10.2337/diacare.22.8.1296[↩]
- Ziegler D, Ametov A, Barinov A, Dyck PJ, Gurieva I, Low PA, Munzel U, Yakhno N, Raz I, Novosadova M, Maus J, Samigullin R. Oral treatment with alpha-lipoic acid improves symptomatic diabetic polyneuropathy: the SYDNEY 2 trial. Diabetes Care. 2006 Nov;29(11):2365-70. doi: 10.2337/dc06-1216[↩]
- Ziegler D, Low PA, Litchy WJ, Boulton AJ, Vinik AI, Freeman R, Samigullin R, Tritschler H, Munzel U, Maus J, Schütte K, Dyck PJ. Efficacy and safety of antioxidant treatment with α-lipoic acid over 4 years in diabetic polyneuropathy: the NATHAN 1 trial. Diabetes Care. 2011 Sep;34(9):2054-60. doi: 10.2337/dc11-0503[↩][↩]
- Ziegler D, Low PA, Freeman R, Tritschler H, Vinik AI. Predictors of improvement and progression of diabetic polyneuropathy following treatment with α-lipoic acid for 4 years in the NATHAN 1 trial. J Diabetes Complications. 2016 Mar;30(2):350-6. doi: 10.1016/j.jdiacomp.2015.10.018[↩]
- Alpha-Lipoic Acid. https://ncit.nci.nih.gov/ncitbrowser/ConceptReport.jsp?dictionary=NCI_Thesaurus&ns=NCI_Thesaurus&code=C61595[↩]
- Haritoglou C, Gerss J, Hammes HP, et al. Alpha-lipoic acid for the prevention of diabetic macular edema. Ophthalmologica. 2011;226(3):127-137. https://www.ncbi.nlm.nih.gov/pubmed/21811051[↩]
- Çakici N, Fakkel TM, van Neck JW, et al. Systematic review of treatments for diabetic peripheral neuropathy. Diabetic Medicine. 2016;33(11):1466-1476.[↩]
- Mayr JA, Feichtinger RG, Tort F, Ribes A, Sperl W. Lipoic acid biosynthesis defects. J Inherit Metab Dis. 2014 Jul;37(4):553-63. doi: 10.1007/s10545-014-9705-8[↩]
- Mayr JA, Zimmermann FA, Fauth C, Bergheim C, Meierhofer D, Radmayr D, Zschocke J, Koch J, Sperl W. Lipoic acid synthetase deficiency causes neonatal-onset epilepsy, defective mitochondrial energy metabolism, and glycine elevation. Am J Hum Genet. 2011 Dec 9;89(6):792-7. doi: 10.1016/j.ajhg.2011.11.011[↩]
- Tort F, Ferrer-Cortès X, Thió M, Navarro-Sastre A, Matalonga L, Quintana E, Bujan N, Arias A, García-Villoria J, Acquaviva C, Vianey-Saban C, Artuch R, García-Cazorla À, Briones P, Ribes A. Mutations in the lipoyltransferase LIPT1 gene cause a fatal disease associated with a specific lipoylation defect of the 2-ketoacid dehydrogenase complexes. Hum Mol Genet. 2014 Apr 1;23(7):1907-15. doi: 10.1093/hmg/ddt585[↩]
- Tripathi AK, Ray AK, Mishra SK, Bishen SM, Mishra H, Khurana A. Molecular and Therapeutic Insights of Alpha-Lipoic Acid as a Potential Molecule for Disease Prevention. Rev Bras Farmacogn. 2023;33(2):272-287. doi: 10.1007/s43450-023-00370-1[↩]
- Wang H-C, Chou H-C, Chen C-M. Molecular Mechanisms of Hyperoxia-Induced Neonatal Intestinal Injury. International Journal of Molecular Sciences. 2023; 24(5):4366. https://doi.org/10.3390/ijms24054366[↩]
- Radomska-Leśniewska D.M., Skopiński P. N-acetylcysteine as an anti-oxidant and anti-inflammatory drug and its some clinical applications. Cent. J. Immunol. 2012;37:57–66.[↩]
- Ershad M, Naji A, Patel P, et al. N-Acetylcysteine. [Updated 2024 Feb 29]. In: StatPearls [Internet]. Treasure Island (FL): StatPearls Publishing; 2024 Jan-. Available from: https://www.ncbi.nlm.nih.gov/books/NBK537183[↩][↩]
- LiverTox: Clinical and Research Information on Drug-Induced Liver Injury [Internet]. Bethesda (MD): National Institute of Diabetes and Digestive and Kidney Diseases; 2012-. Acetylcysteine. [Updated 2016 Nov 7]. Available from: https://www.ncbi.nlm.nih.gov/books/NBK548401[↩]
- El-Maddawy ZK, El-Sayed YS. Comparative analysis of the protective effects of curcumin and N-acetyl cysteine against paracetamol-induced hepatic, renal, and testicular toxicity in Wistar rats. Environ Sci Pollut Res Int. 2018 Feb;25(4):3468-3479. doi: 10.1007/s11356-017-0750-3[↩]
- Scalley RD, Conner CS. Acetaminophen poisoning: a case report of the use of acetylcysteine. Am J Hosp Pharm. 1978 Aug;35(8):964-7.[↩]
- N-Acetyl Cysteine (NAC). https://medlineplus.gov/druginfo/natural/1018.html[↩][↩]
- Gracey M., Burke V., Anderson C. M. Treatment of abdominal pain in cystic fibrosis by oral administration of n-acetyl cysteine. Archives of Disease in Childhood. 1969;44(235):404–405. doi: 10.1136/adc.44.235.404[↩]
- Idiopathic Pulmonary Fibrosis. Center for Drug Evaluation and Research (CDER) and the U.S. Food and Drug Administration (FDA). https://www.fda.gov/media/91396/download[↩]
- Dodd S, Dean O, Copolov DL, Malhi GS, Berk M. N-acetylcysteine for antioxidant therapy: pharmacology and clinical utility. Expert Opin Biol Ther. 2008 Dec;8(12):1955-62. doi: 10.1517/14728220802517901[↩][↩]
- Adair JC, Knoefel JE, Morgan N. Controlled trial of N-acetylcysteine for patients with probable Alzheimer’s disease. Neurology. 2001 Oct 23;57(8):1515-7. doi: 10.1212/wnl.57.8.1515[↩]
- Dean O, Giorlando F, Berk M. N-acetylcysteine in psychiatry: current therapeutic evidence and potential mechanisms of action. J Psychiatry Neurosci. 2011 Mar;36(2):78-86. doi: 10.1503/jpn.100057[↩][↩]
- Javaherforooshzadeh F, Shaker Z, Rashidi M, Akhondzadeh R, Hayati F. The effect of N-acetyl cysteine injection on renal function after coronary artery bypass graft surgery: a randomized double blind clinical trial. J Cardiothorac Surg. 2021 Jun 5;16(1):161. doi: 10.1186/s13019-021-01550-7[↩]
- Fliser D., Laville M., Covic A., Fouque D., Vanholder R., Juillard L., Van Biesen W., Ad-hoc working group of ERBP A European Renal Best Practice (ERBP) position statement on the Kidney Disease Improving Global Outcomes (KDIGO) clinical practice guidelines on acute kidney injury: part 1: definitions, conservative management and contrast-induced nephropathy. Nephrol. Dial. Transplant. 2012;27(12):4263–4272. doi: 10.1093/ndt/gfs375[↩]
- Diniz Y.S., Rocha K.K.H.R., Souza G.A., Galhardi C.M., Ebaid G.M.X., Rodrigues H.G., Novelli Filho J.L.V.B., Cicogna A.C., Novelli E.L.B. Effects of N-acetylcysteine on sucrose-rich diet-induced hyperglycaemia, dyslipidemia and oxidative stress in rats. Eur. J. Pharmacol. 2006;543:151–157. doi: 10.1016/j.ejphar.2006.05.039[↩]
- Campos K.E., Diniz Y.S., Cataneo A.C., Faine L.A., Alves M.J.Q.F., Novelli E.L.B. Hypoglycaemic and antioxidant effects of onion, Allium cepa: Dietary onion addition, antioxidant activity and hypoglycaemic effects on diabetic rats. Int. J. Food Sci. Nutr. 2003;54:241–246. doi: 10.1080/09637480120092062[↩]
- Atkuri KR, Mantovani JJ, Herzenberg LA, Herzenberg LA. N-Acetylcysteine–a safe antidote for cysteine/glutathione deficiency. Curr Opin Pharmacol. 2007 Aug;7(4):355-9. doi: 10.1016/j.coph.2007.04.005[↩]
- Vasdev S., Singal P., Gill V. The antihypertensive effect of cysteine. The International Journal of Angiology: Official Publication of the International College of Angiology, Inc. 2009;18(1):7–21. doi: 10.1055/s-0031-1278316[↩]
- Šalamon Š, Kramar B, Marolt TP, Poljšak B, Milisav I. Medical and Dietary Uses of N-Acetylcysteine. Antioxidants (Basel). 2019 Apr 28;8(5):111. doi: 10.3390/antiox8050111[↩]
- Niemi T. T. The effect of N-acetylcysteine on blood coagulation and platelet function in patients undergoing open repair of abdominal aortic aneurysm. Blood Coagulation and Fibrinolysis. 2006;17(1):29–34. doi: 10.1097/01.mbc.0000195922.26950.89[↩]
- Ardissino D., Savonitto S., Merlini P. A., et al. Effect of transdermal nitroglycerin or N-acetylcysteine, or both, in the long-term treatment of unstable Angina pectoris. Journal of the American College of Cardiology. 1997;29(5):941–947. doi: 10.1016/s0735-1097(97)00005-3[↩]
- Sansone RA, Sansone LA. Getting a Knack for NAC: N-Acetyl-Cysteine. Innov Clin Neurosci. 2011 Jan;8(1):10-4. https://www.ncbi.nlm.nih.gov/pmc/articles/PMC3036554/[↩]
- Kerksick C., Willoughby D. The antioxidant role of glutathione and N-Acetyl-Cysteine supplements and exercise-induced oxidative stress. Journal of the International Society of Sports Nutrition. 2005;2(2):p. 38. doi: 10.1186/1550-2783-2-2-38[↩]
- Fass D., Thorpe C. Chemistry and enzymology of disulfide cross-linking in proteins. Chemical Review. 2018;118(3):1169–1198. doi: 10.1021/acs.chemrev.7b00123[↩]
- De Flora S., Izzotti A., D’Agostini F., Balansky R. Mechanisms of N-acetylcysteine in the prevention of DNA damage and cancer, with special reference to smoking-related end-points. Carcinogenesis. 2001;22(7):999–1013. doi: 10.1093/carcin/22.7.999[↩]
- Halasi M., Wang M., Chavan T. S., Gaponenko V., Hay N., Gartel A. L. ROS inhibitor N-acetyl-L-cysteine antagonizes the activity of proteasome inhibitors. Biochemical Journal. 2013;454(2):201–208. doi: 10.1042/bj20130282[↩]
- Uraz S. N-acetylcysteine expresses powerful anti-inflammatory and antioxidant activities resulting in complete improvement of acetic acid-induced colitis in rats. Scandinavian Journal of Clinical and Laboratory Investigation. 2013;73(1):61–66. doi: 10.3109/00365513.2012.734859[↩]
- Zhitkovich A. N-Acetylcysteine: Antioxidant, aldehyde scavenger, and more. Chemical Research in Toxicology. 2019;32(7):1318–1319. doi: 10.1021/acs.chemrestox.9b00152[↩]
- Livingstone C. R., Andrews M. A., Jenkins S. M., Marriott C. Model systems for the evaluation of mucolytic drugs: acetylcysteine and S-carboxymethylcysteine. Journal of Pharmacy and Pharmacology. 1990;42(2):73–78. doi: 10.1111/j.2042-7158.1990.tb05357.x[↩]
- Fries G. R., Kapczinski F. N-acetylcysteine as a mitochondrial enhancer: a new class of psychoactive drugs? Brazilian Journal of Psychiatry. 2011;33:321–322. doi: 10.1590/s1516-44462011000400003[↩]
- Jiao Y. N-acetyl cysteine depletes reactive oxygen species and prevents dental monomer-induced intrinsic mitochondrial apoptosis in vitro in human dental pulp cells. PLOS ONE. 2016;11(1) doi: 10.1371/journal.pone.0147858.e0147858[↩]
- Rossignol D. A. The use of N-acetylcysteine as a chelator for metal toxicity. In: Frye R. E., Berk M., editors. The Therapeutic Use of N-Acetylcysteine (NAC) in Medicine. Singapore: Springer Singapore; 2019. pp. 169–179.[↩]
- Tomàs Casanova M. G. N-Acetylcysteine: An old drug with variable anti-influenza properties. Journal of Controversies in Biomedical Research. 2016;2(1):1–8. doi: 10.15586/jcbmr.2016.13[↩]
- Redondo P., Jimenez E., Perez A., García-Foncillas J. N-acetylcysteine downregulates vascular endothelial growth factor production by human keratinocytes in vitro. Archives of Dermatological Research. 2000;292(12):621–628. doi: 10.1007/s004030000187[↩]
- Douiev L., Soiferman D., Alban C., Saada A. The effects of ascorbate, N-acetylcysteine, and resveratrol on fibroblasts from patients with mitochondrial disorders. Journal of Clinical Medicine. 2016;6(1) doi: 10.3390/jcm6010001[↩]
- Martina V. Long-term N-acetylcysteine and L-arginine administration reduces endothelial activation and systolic blood pressure in hypertensive patients with type 2 diabetes. Diabetes Care. 2008;31(5):940–944. doi: 10.2337/dc07-2251[↩]
- Schwalfenberg GK. N-Acetylcysteine: A Review of Clinical Usefulness (an Old Drug with New Tricks). J Nutr Metab. 2021 Jun 9;2021:9949453. doi: 10.1155/2021/9949453[↩]
- Elbahnaswy S, Elshopakey GE. Recent progress in practical applications of a potential carotenoid astaxanthin in aquaculture industry: a review. Fish Physiol Biochem. 2024 Feb;50(1):97-126. doi: 10.1007/s10695-022-01167-0[↩]
- Nishida Y, Berg PC, Shakersain B, Hecht K, Takikawa A, Tao R, Kakuta Y, Uragami C, Hashimoto H, Misawa N, Maoka T. Astaxanthin: Past, Present, and Future. Mar Drugs. 2023 Sep 28;21(10):514. doi: 10.3390/md21100514[↩]
- Wang S, Qi X. The Putative Role of Astaxanthin in Neuroinflammation Modulation: Mechanisms and Therapeutic Potential. Front Pharmacol. 2022 Jun 24;13:916653. doi: 10.3389/fphar.2022.916653[↩]
- Nair A, Ahirwar A, Singh S, Lodhi R, Lodhi A, Rai A, Jadhav DA, Harish, Varjani S, Singh G, Marchand J, Schoefs B, Vinayak V. Astaxanthin as a King of Ketocarotenoids: Structure, Synthesis, Accumulation, Bioavailability and Antioxidant Properties. Mar Drugs. 2023 Mar 13;21(3):176. doi: 10.3390/md21030176[↩]
- Astaxanthin: a review of its chemistry and applications. Higuera-Ciapara I, Félix-Valenzuela L, Goycoolea FM. Crit Rev Food Sci Nutr. 2006; 46(2):185-96. https://www.tandfonline.com/doi/abs/10.1080/10408690590957188[↩]
- Li Y, Gong F, Guo S, Yu W, Liu J. Adonis amurensis Is a Promising Alternative to Haematococcus as a Resource for Natural Esterified (3S,3’S)-Astaxanthin Production. Plants (Basel). 2021 May 25;10(6):1059. doi: 10.3390/plants10061059[↩][↩]
- Sarada R., Ranga Rao A., Sandesh B.K., Dayananda C., Anila N., Chauhan V.S., Ravishankar G.A. Influence of different culture conditions on yield of biomass and value added products in microalgae. Dyn. Biochem. Proc. Biotechnol. Mol. Biol. 2012;6:77–85.[↩]
- Kidd P. Astaxanthin, cell membrane nutrient with diverse clinical benefits and anti-aging potential. Altern. Med. Rev. 2011;16:355–364. http://archive.foundationalmedicinereview.com/publications/16/4/355.pdf[↩]
- Nishida Y. Astaxanthin: Commercial production and its potential health-promoting effects. Oleoscience. 2012;12:525–531. doi: 10.5650/oleoscience.12.525[↩][↩]
- Ambati R.R., Phang S.-M., Ravi S., Aswathanarayana R.G. Astaxanthin: Sources, Extraction, Stability, Biological Activities and Its Commercial Applications—A Review. Mar. Drugs. 2014;12:128–152. doi: 10.3390/md12010128[↩][↩]
- Stachowiak B., Szulc P. Astaxanthin for the Food Industry. Molecules. 2021;26:2666. doi: 10.3390/molecules26092666[↩]
- Maoka T. Carotenoids in Marine Animals. Mar. Drugs. 2011;9:278–293. doi: 10.3390/md9020278[↩]
- Matsuno T. Aquatic animal carotenoids. Fish. Sci. 2001;67:771–783. doi: 10.1046/j.1444-2906.2001.00323.x[↩]
- Eric A. Johnson & Gil-Hwan An (1991) Astaxanthin from Microbial Sources, Critical Reviews in Biotechnology, 11:4, 297-326, DOI: 10.3109/07388559109040622 https://www.tandfonline.com/doi/abs/10.3109/07388559109040622[↩][↩][↩][↩]
- Ambati RR, Siew Moi P, Ravi S, Aswathanarayana RG. Astaxanthin: Sources, Extraction, Stability, Biological Activities and Its Commercial Applications—A Review. Marine Drugs. 2014;12(1):128-152. doi:10.3390/md12010128. https://www.ncbi.nlm.nih.gov/pmc/articles/PMC3917265/[↩]
- Sood B, Patel P, Keenaghan M. Coenzyme Q10. [Updated 2024 Jan 30]. In: StatPearls [Internet]. Treasure Island (FL): StatPearls Publishing; 2024 Jan-. Available from: https://www.ncbi.nlm.nih.gov/books/NBK531491[↩]
- Ernster L, Forsmark-Andrée P. Ubiquinol: an endogenous antioxidant in aerobic organisms. Clin Investig. 1993;71(8 Suppl):S60-5. doi: 10.1007/BF00226842[↩][↩]
- Folkers K. The potential of coenzyme Q 10 (NSC-140865) in cancer treatment. Cancer Chemother Rep 2. 1974 Dec;4(4):19-22.[↩]
- Ambrosone CB, Zirpoli GR, Hutson AD, et al. Dietary Supplement Use During Chemotherapy and Survival Outcomes of Patients With Breast Cancer Enrolled in a Cooperative Group Clinical Trial (SWOG S0221). J Clin Oncol. 2020 Mar 10;38(8):804-814. doi: 10.1200/JCO.19.01203[↩][↩]
- Cortes EP, Gupta M, Chou C, Amin VC, Folkers K. Adriamycin cardiotoxicity: early detection by systolic time interval and possible prevention by coenzyme Q10. Cancer Treat Rep. 1978 Jun;62(6):887-91.[↩][↩]
- Folkers K, Brown R, Judy WV, Morita M. Survival of cancer patients on therapy with coenzyme Q10. Biochem Biophys Res Commun. 1993 Apr 15;192(1):241-5. doi: 10.1006/bbrc.1993.1405[↩]
- Iarussi D, Auricchio U, Agretto A, Murano A, Giuliano M, Casale F, Indolfi P, Iacono A. Protective effect of coenzyme Q10 on anthracyclines cardiotoxicity: control study in children with acute lymphoblastic leukemia and non-Hodgkin lymphoma. Mol Aspects Med. 1994;15 Suppl:s207-12. doi: 10.1016/0098-2997(94)90030-2[↩]
- Lesser GJ, Case D, Stark N, Williford S, Giguere J, Garino LA, Naughton MJ, Vitolins MZ, Lively MO, Shaw EG; Wake Forest University Community Clinical Oncology Program Research Base. A randomized, double-blind, placebo-controlled study of oral coenzyme Q10 to relieve self-reported treatment-related fatigue in newly diagnosed patients with breast cancer. J Support Oncol. 2013 Mar;11(1):31-42. doi: 10.1016/j.suponc.2012.03.003[↩]
- Iwase S, Kawaguchi T, Yotsumoto D, Doi T, Miyara K, Odagiri H, Kitamura K, Ariyoshi K, Miyaji T, Ishiki H, Inoue K, Tsutsumi C, Sagara Y, Yamaguchi T. Efficacy and safety of an amino acid jelly containing coenzyme Q10 and L-carnitine in controlling fatigue in breast cancer patients receiving chemotherapy: a multi-institutional, randomized, exploratory trial (JORTC-CAM01). Support Care Cancer. 2016 Feb;24(2):637-646. doi: 10.1007/s00520-015-2824-4[↩]
- Folkers K, Osterborg A, Nylander M, Morita M, Mellstedt H. Activities of vitamin Q10 in animal models and a serious deficiency in patients with cancer. Biochem Biophys Res Commun. 1997 May 19;234(2):296-9. doi: 10.1006/bbrc.1997.6522[↩]
- Du J, Wang T, Huang P, Cui S, Gao C, Lin Y, Fu R, Shen J, He Y, Tan Y, Chen S. Clinical correlates of decreased plasma coenzyme Q10 levels in patients with multiple system atrophy. Parkinsonism Relat Disord. 2018 Dec;57:58-62. doi: 10.1016/j.parkreldis.2018.07.017[↩]
- Chang PS, Chou HH, Lai TJ, Yen CH, Pan JC, Lin PT. Investigation of coenzyme Q10 status, serum amyloid-β, and tau protein in patients with dementia. Front Aging Neurosci. 2022 Jul 25;14:910289. doi: 10.3389/fnagi.2022.910289[↩]
- Dahri M, Tarighat-Esfanjani A, Asghari-Jafarabadi M, Hashemilar M. Oral coenzyme Q10 supplementation in patients with migraine: Effects on clinical features and inflammatory markers. Nutr Neurosci. 2019 Sep;22(9):607-615. doi: 10.1080/1028415X.2017.1421039[↩][↩][↩][↩]
- Al Saadi T, Assaf Y, Farwati M, Turkmani K, Al-Mouakeh A, Shebli B, Khoja M, Essali A, Madmani ME. Coenzyme Q10 for heart failure. Cochrane Database Syst Rev. 2021 Feb 3;(2)(2):CD008684. doi: 10.1002/14651858.CD008684.pub3[↩]
- Rauchová H. Coenzyme Q10 effects in neurological diseases. Physiol Res. 2021 Dec 30;70(Suppl4):S683-S714. doi: 10.33549/physiolres.934712[↩]
- Ho CC, Tseng CY, Chen HW, Chiu YW, Tsai MC, Chang PS, Lin PT. Coenzyme Q10 status, glucose parameters, and antioxidative capacity in college athletes. J Int Soc Sports Nutr. 2020 Jan 10;17(1):5. doi: 10.1186/s12970-020-0334-3[↩]
- Cordero MD, Santos-García R, Bermejo-Jover D, Sánchez-Domínguez B, Jaramillo-Santos MR, Bullón P. Coenzyme Q10 in salivary cells correlate with blood cells in Fibromyalgia: improvement in clinical and biochemical parameter after oral treatment. Clin Biochem. 2012 Apr;45(6):509-11. doi: 10.1016/j.clinbiochem.2012.02.001[↩]
- Jafari M, Mousavi SM, Asgharzadeh A, Yazdani N. Coenzyme Q10 in the treatment of heart failure: A systematic review of systematic reviews. Indian Heart J. 2018 Jul;70 Suppl 1(Suppl 1):S111-S117. doi: 10.1016/j.ihj.2018.01.031[↩][↩][↩]
- Garrido-Maraver J, Cordero MD, Oropesa-Ávila M, Fernández Vega A, de la Mata M, Delgado Pavón A, de Miguel M, Pérez Calero C, Villanueva Paz M, Cotán D, Sánchez-Alcázar JA. Coenzyme q10 therapy. Mol Syndromol. 2014 Jul;5(3-4):187-97. doi: 10.1159/000360101[↩]
- Niklowitz P, Sonnenschein A, Janetzky B, Andler W, Menke T. Enrichment of coenzyme Q10 in plasma and blood cells: defense against oxidative damage. International Journal of Biological Sciences 2007;3(4):257-62.[↩]
- Bhagavan H.N., Chopra R.K. Coenzyme Q10: Absorption, tissue uptake, metabolism and pharmacokinetics. Free Radic. Res. 2006;40:445–453. doi: 10.1080/10715760600617843[↩]
- Flowers N., Hartley L., Todkill D., Stranges S., Rees K. Co-enzyme Q10 supplementation for the primary prevention of cardiovascular disease. Cochrane Database Syst. Rev. 2014;12:CD010405 https://www.ncbi.nlm.nih.gov/pubmed/25474484[↩]
- Yoneda T., Tomofuji T., Kawabata Y., Ekuni D., Azuma T., Kataoka K., Kunitomo M., Morita M. Application of coenzyme Q10 for accelerating soft tissue wound healing after tooth extraction in rats. Nutrients. 2014;6:5756–5769. doi: 10.3390/nu6125756[↩]
- Fouad A.A., Al-Mulhim A.S., Jresat I. Therapeutic effect of coenzyme Q10 against experimentally-induced hepatocellular carcinoma in rats. Environ. Toxicol. Pharmacol. 2013;35:100–108. doi: 10.1016/j.etap.2012.11.016[↩]
- PDQ Integrative, Alternative, and Complementary Therapies Editorial Board. Coenzyme Q10 (PDQ®): Health Professional Version. 2024 Apr 5. In: PDQ Cancer Information Summaries [Internet]. Bethesda (MD): National Cancer Institute (US); 2002-. Available from: https://www.ncbi.nlm.nih.gov/books/NBK65890[↩][↩][↩]
- Schniertshauer D, Müller S, Mayr T, Sonntag T, Gebhard D, Bergemann J. Accelerated Regeneration of ATP Level after Irradiation in Human Skin Fibroblasts by Coenzyme Q10. Photochem Photobiol. 2016 May;92(3):488-94. doi: 10.1111/php.12583[↩]
- Usui T, Ishikura H, Izumi Y, Konishi H, Dohmae N, Sawada H, Uchino H, Matsuda H, Konishi T. Possible prevention from the progression of cardiotoxicity in adriamycin-treated rabbits by coenzyme Q10. Toxicol Lett. 1982 Jun;12(1):75-82. doi: 10.1016/0378-4274(82)90201-6[↩]
- Iwamoto Y, Hansen IL, Porter TH, Folkers K. Inhibition of coenzyme Q10-enzymes, succinoxidase and NADH-oxidase, by adriamycin and other quinones having antitumor activity. Biochem Biophys Res Commun. 1974 Jun 4;58(3):633-8. doi: 10.1016/s0006-291x(74)80465-1[↩]
- Kędziora-Kornatowska K, Czuczejko J, Motyl J, Szewczyk-Golec K, Kozakiewicz M, Pawluk H, Kędziora J, Błaszczak R, Banach M, Rysz J. Effects of coenzyme Q10 supplementation on activities of selected antioxidative enzymes and lipid peroxidation in hypertensive patients treated with indapamide. A pilot study. Arch Med Sci. 2010 Aug 30;6(4):513-8. doi: 10.5114/aoms.2010.14461[↩]
- Cordero MD, Cano-García FJ, Alcocer-Gómez E, De Miguel M, Sánchez-Alcázar JA. Oxidative stress correlates with headache symptoms in fibromyalgia: coenzyme Q₁₀ effect on clinical improvement. PLoS One. 2012;7(4):e35677. doi: 10.1371/journal.pone.0035677[↩]
- Flowers N, Hartley L, Todkill D, Stranges S, Rees K. Co-enzyme Q10 supplementation for the primary prevention of cardiovascular disease. Cochrane Database of Systematic Reviews 2014, Issue 12. Art. No.: CD010405. DOI: 10.1002/14651858.CD010405.pub2. http://cochranelibrary-wiley.com/doi/10.1002/14651858.CD010405.pub2/full[↩]
- Folkers K, Langsjoen P, Willis R, Richardson P, Xia LJ, Ye CQ, et al. Lovastatin decreases coenzyme Q levels in humans. Proceedings of the National Academy of Sciences of the United States of America 1990;87:8931-4.[↩]
- Schaars CF, Stalenhoef AF. Effects of ubiquinone (coenzyme Q10) on myopathy in statin users. Current Opinion in Lipidology 2008;19:553-7.[↩]
- Kumar A, Kaur H, Devi P, Mohan V. Role of coenzyme Q10 (CoQ10) in cardiac disease, hypertension and Meniere-like syndrome. Pharmacology and Therapeutics 2009;124(3):259-68.[↩][↩]
- Caso G, Kelly P, McNurlan MA, Lawson WE. Effect of coenzyme q10 on myopathic symptoms in patients treated with statins. American Journal of Cardiology 2007;99(10):1409-12.[↩]
- Niklowitz P, Sonnenschein A, Janetzky B, Andler W, Menke T. Enrichment of coenzyme Q10 in plasma and blood cells: defence against oxidative damage. International Journal of Biological Sciences 2007;3(4):257-62.[↩][↩]
- Rosenfeldt FL, Haas SJ, Krum H, Hadj A, Ng K, Leong J-Y, et al. Coenzyme Q10 in the treatment of hypertension: a meta-analysis of the clinical trials. Journal of Human Hypertension 2007;21:297–306.[↩]
- McCarty MF. Coenzyme Q versus hypertension: does CoQ decrease endothelial superoxide generation?. Medical Hypotheses 1999;53(4):300-4.[↩]
- Gao L, Mao Q, Cao J, Wang Y, Zhou X, Fan L. Effects of coenzyme Q10 on vascular endothelial function in humans: a meta-analysis of randomized controlled trials. Atherosclerosis 2012;221(2):311-6.[↩]
- Ignarro LJ. Biological actions and properties of endothelium-derived nitric oxide formed and released from artery and vein. Circulation Research 1989;65:1-21.[↩]
- Kato T, Yoneda S. Reduction in blood viscosity by treatment with coenzyme Q10 in patients with ischemic heart disease. International Journal of Clinical Pharmacology, Therapy and Toxicology 1990;28(3):123-6.[↩]
- Turunen M, Wehlin L, Sjoberg M, Lundahl J, Dallner G, Brismar K, et al. Beta2-Integrin and lipid modifications indicate a non-antioxidant mechanism for the anti-atherogenic effect of dietary coenzyme Q10. Biochemical and Biophysical Research Communications 2002;296:255-60.[↩]
- Correction to: 2022 AHA/ACC/HFSA Guideline for the Management of Heart Failure: A Report of the American College of Cardiology/American Heart Association Joint Committee on Clinical Practice Guidelines. Circulation. 2022 Sep 27;146(13):e185. doi: 10.1161/CIR.0000000000001097. Epub 2022 Sep 26. Erratum for: Circulation. 2022 May 3;145(18):e895-e1032. doi: 10.1161/CIR.0000000000001063[↩][↩]
- Bhatt KN, Butler J. Myocardial Energetics and Heart Failure: a Review of Recent Therapeutic Trials. Curr Heart Fail Rep. 2018 Jun;15(3):191-197. doi: 10.1007/s11897-018-0386-8[↩]
- Belardinelli R, Muçaj A, Lacalaprice F, Solenghi M, Seddaiu G, Principi F, Tiano L, Littarru GP. Coenzyme Q10 and exercise training in chronic heart failure. Eur Heart J. 2006 Nov;27(22):2675-81. doi: 10.1093/eurheartj/ehl158[↩]
- Dai YL, Luk TH, Yiu KH, Wang M, Yip PM, Lee SW, Li SW, Tam S, Fong B, Lau CP, Siu CW, Tse HF. Reversal of mitochondrial dysfunction by coenzyme Q10 supplement improves endothelial function in patients with ischaemic left ventricular systolic dysfunction: a randomized controlled trial. Atherosclerosis. 2011 Jun;216(2):395-401. doi: 10.1016/j.atherosclerosis.2011.02.013[↩][↩]
- Zozina VI, Covantev S, Goroshko OA, Krasnykh LM, Kukes VG. Coenzyme Q10 in Cardiovascular and Metabolic Diseases: Current State of the Problem. Curr Cardiol Rev. 2018;14(3):164-174. doi: 10.2174/1573403X14666180416115428[↩]
- Watts GF, Playford DA, Croft KD, Ward NC, Mori TA, Burke V. Coenzyme Q(10) improves endothelial dysfunction of the brachial artery in Type II diabetes mellitus. Diabetologia. 2002 Mar;45(3):420-6. doi: 10.1007/s00125-001-0760-y[↩]
- Ayers J, Cook J, Koenig RA, Sisson EM, Dixon DL. Recent Developments in the Role of Coenzyme Q10 for Coronary Heart Disease: a Systematic Review. Curr Atheroscler Rep. 2018 May 16;20(6):29. doi: 10.1007/s11883-018-0730-1[↩]
- Alehagen U, Aaseth J, Alexander J, Johansson P. Still reduced cardiovascular mortality 12 years after supplementation with selenium and coenzyme Q10 for four years: A validation of previous 10-year follow-up results of a prospective randomized double-blind placebo-controlled trial in elderly. PLoS One. 2018 Apr 11;13(4):e0193120. doi: 10.1371/journal.pone.0193120[↩]
- Wong AP, Kassab YW, Mohamed AL, Abdul Qader AM. Review: Beyond conventional therapies: Complementary and alternative medicine in the management of hypertension: An evidence-based review. Pak J Pharm Sci. 2018 Jan;31(1):237-244.[↩]
- Cordero MD, Alcocer-Gómez E, de Miguel M, Culic O, Carrión AM, Alvarez-Suarez JM, Bullón P, Battino M, Fernández-Rodríguez A, Sánchez-Alcazar JA. Can coenzyme q10 improve clinical and molecular parameters in fibromyalgia? Antioxid Redox Signal. 2013 Oct 20;19(12):1356-61. doi: 10.1089/ars.2013.5260[↩]
- Cordero MD, Alcocer-Gómez E, de Miguel M, Cano-García FJ, Luque CM, Fernández-Riejo P, Fernández AM, Sánchez-Alcazar JA. Coenzyme Q(10): a novel therapeutic approach for Fibromyalgia? case series with 5 patients. Mitochondrion. 2011 Jul;11(4):623-5. doi: 10.1016/j.mito.2011.03.122[↩]
- Glover EI, Martin J, Maher A, Thornhill RE, Moran GR, Tarnopolsky MA. A randomized trial of coenzyme Q10 in mitochondrial disorders. Muscle Nerve. 2010 Nov;42(5):739-48. doi: 10.1002/mus.21758[↩]
- Safarinejad MR. Safety and efficacy of coenzyme Q10 supplementation in early chronic Peyronie’s disease: a double-blind, placebo-controlled randomized study. Int J Impot Res. 2010 Sep-Oct;22(5):298-309. doi: 10.1038/ijir.2010.20[↩]
- Zaleski AL, Taylor BA, Thompson PD. Coenzyme Q10 as Treatment for Statin-Associated Muscle Symptoms-A Good Idea, but…. Adv Nutr. 2018 Jul 1;9(4):519S-523S. doi: 10.1093/advances/nmy010[↩]
- Skarlovnik A, Janić M, Lunder M, Turk M, Šabovič M. Coenzyme Q10 supplementation decreases statin-related mild-to-moderate muscle symptoms: a randomized clinical study. Med Sci Monit. 2014 Nov 6;20:2183-8. doi: 10.12659/MSM.890777[↩]
- Mizuno K, Tanaka M, Nozaki S, Mizuma H, Ataka S, Tahara T, Sugino T, Shirai T, Kajimoto Y, Kuratsune H, Kajimoto O, Watanabe Y. Antifatigue effects of coenzyme Q10 during physical fatigue. Nutrition. 2008 Apr;24(4):293-9. doi: 10.1016/j.nut.2007.12.007. Epub 2008 Feb 13. Erratum in: Nutrition. 2008 Jun;24(6):616.[↩]
- Hershey AD, Powers SW, Vockell AL, Lecates SL, Ellinor PL, Segers A, Burdine D, Manning P, Kabbouche MA. Coenzyme Q10 deficiency and response to supplementation in pediatric and adolescent migraine. Headache. 2007 Jan;47(1):73-80. doi: 10.1111/j.1526-4610.2007.00652.x[↩]
- Yaghini O, Hoseini N, Ghazavi MR, Mansouri V, Nasiri J, Moosavian T, Salehi MM. A Comparative Study on the Efficacy of Coenzyme Q10 and Amitriptyline in the Prophylactic Treatment of Migraine Headaches in Children: A Randomized Controlled Trial. Adv Biomed Res. 2022 May 30;11:43. doi: 10.4103/abr.abr_235_20[↩]
- Sándor PS, Di Clemente L, Coppola G, Saenger U, Fumal A, Magis D, Seidel L, Agosti RM, Schoenen J. Efficacy of coenzyme Q10 in migraine prophylaxis: a randomized controlled trial. Neurology. 2005 Feb 22;64(4):713-5. doi: 10.1212/01.WNL.0000151975.03598.ED[↩]
- Shoeibi A, Olfati N, Soltani Sabi M, Salehi M, Mali S, Akbari Oryani M. Effectiveness of coenzyme Q10 in prophylactic treatment of migraine headache: an open-label, add-on, controlled trial. Acta Neurol Belg. 2017 Mar;117(1):103-109. doi: 10.1007/s13760-016-0697-z[↩]
- Chase M, Cocchi MN, Liu X, Andersen LW, Holmberg MJ, Donnino MW. Coenzyme Q10 in acute influenza. Influenza Other Respir Viruses. 2019 Jan;13(1):64-70. doi: 10.1111/irv.12608[↩]
- Mehrpooya M, Yasrebifar F, Haghighi M, Mohammadi Y, Jahangard L. Evaluating the Effect of Coenzyme Q10 Augmentation on Treatment of Bipolar Depression: A Double-Blind Controlled Clinical Trial. J Clin Psychopharmacol. 2018 Oct;38(5):460-466. doi: 10.1097/JCP.0000000000000938[↩]
- Izadi A, Ebrahimi S, Shirazi S, Taghizadeh S, Parizad M, Farzadi L, Gargari BP. Hormonal and Metabolic Effects of Coenzyme Q10 and/or Vitamin E in Patients With Polycystic Ovary Syndrome. J Clin Endocrinol Metab. 2019 Feb 1;104(2):319-327. doi: 10.1210/jc.2018-01221[↩]
- Salviati L, Trevisson E, Agosto C, et al. Primary Coenzyme Q10 Deficiency Overview. 2017 Jan 26 [Updated 2023 Jun 8]. In: Adam MP, Feldman J, Mirzaa GM, et al., editors. GeneReviews® [Internet]. Seattle (WA): University of Washington, Seattle; 1993-2024. Available from: https://www.ncbi.nlm.nih.gov/books/NBK410087[↩][↩][↩][↩][↩]
- Yuruk Yildirim Z, Toksoy G, Uyguner O, Nayir A, Yavuz S, Altunoglu U, Turkkan ON, Sevinc B, Gokcay G, Kurkcu Gunes D, Kiyak A, Yilmaz A. Primary coenzyme Q10 Deficiency-6 (COQ10D6): Two siblings with variable expressivity of the renal phenotype. Eur J Med Genet. 2020 Jan;63(1):103621. doi: 10.1016/j.ejmg.2019.01.011[↩][↩]
- Hassanzadeh K, Buccarello L, Dragotto J, Mohammadi A, Corbo M, Feligioni M. Obstacles against the Marketing of Curcumin as a Drug. Int J Mol Sci. 2020 Sep 10;21(18):6619. doi: 10.3390/ijms21186619[↩]
- Alibeiki, F., Jafari, N., Karimi, M., & Peeri Dogaheh, H. (2017). Potent anti-cancer effects of less polar Curcumin analogues on gastric adenocarcinoma and esophageal squamous cell carcinoma cells. Scientific reports, 7(1), 2559. https://doi.org/10.1038/s41598-017-02666-4[↩]
- Ahmad A., Sakr W.A., Rahman K.M. Novel targets for detection of cancer and their modulation by chemopreventive natural compounds. Front. Biosci. 2012;4:410–425. doi: 10.2741/e388[↩]
- LiverTox: Clinical and Research Information on Drug-Induced Liver Injury [Internet]. Bethesda (MD): National Institute of Diabetes and Digestive and Kidney Diseases; 2012-. Turmeric. [Updated 2024 Jun 1]. Available from: https://www.ncbi.nlm.nih.gov/books/NBK548561[↩]
- Nelson KM, Dahlin JL, Bisson J, Graham J, Pauli GF, Walters MA. The Essential Medicinal Chemistry of Curcumin. J Med Chem. 2017 Mar 9;60(5):1620-1637. doi: 10.1021/acs.jmedchem.6b00975[↩]
- Tagde P., Tagde P., Islam F., Tagde S., Shah M., Hussain Z.D., Rahman M.H., Najda A., Alanazi I.S., Germoush M.O., et al. The multifaceted role of curcumin in advanced nanocurcumin form in the treatment and management of chronic disorders. Molecules. 2021;26:7109. doi: 10.3390/molecules26237109[↩]
- Yang C, Zhu Q, Chen Y, Ji K, Li S, Wu Q, Pan Q, Li J. Review of the Protective Mechanism of Curcumin on Cardiovascular Disease. Drug Des Devel Ther. 2024 Jan 30;18:165-192. doi: 10.2147/DDDT.S445555[↩]
- Hsu CH, Cheng AL. Clinical studies with curcumin. Adv Exp Med Biol. 2007;595:471-80. doi: 10.1007/978-0-387-46401-5_21[↩]
- Tanwar V, Sachdeva J, Kishore K, et al. Dose-dependent actions of curcumin in experimentally induced myocardial necrosis: a biochemical, histopathological, and electron microscopic evidence. Cell Biochem Funct. 2010;28(1):74–82. doi: 10.1002/cbf.1623[↩]
- Sun H, Zhu J, Lu T, et al. Curcumin-mediated cardiac defects in mouse is associated with a reduced histone H3 acetylation and reduced expression of cardiac transcription factors. Cardiovasc Toxicol. 2014;14(2):162–169. doi: 10.1007/s12012-013-9240-0[↩][↩]
- Hanai H, Iida T, Takeuchi K, et al. Curcumin maintenance therapy for ulcerative colitis: randomized, multicenter, double-blind, placebo-controlled trial. Clin Gastroenterol Hepatol. 2006;4(12):1502–1506. doi: 10.1016/j.cgh.2006.08.008[↩]
- Na LX, Li Y, Pan HZ, Zhou XL, Sun DJ, Meng M, Li XX, Sun CH. Curcuminoids exert glucose-lowering effect in type 2 diabetes by decreasing serum free fatty acids: a double-blind, placebo-controlled trial. Mol Nutr Food Res. 2013 Sep;57(9):1569-77. doi: 10.1002/mnfr.201200131[↩]
- Hoehle SI, Pfeiffer E, Sólyom AM, et al. Metabolism of curcuminoids in tissue slices and subcellular fractions from rat liver. J Agric Food Chem. 2006;54(3):756–764. doi: 10.1021/jf058146a[↩]
- Zlotogorski, A.; Dayan, A.; Dayan, D.; Chaushu, G.; Salo, T.; Vered, M. (2013). Nutraceuticals as new treatment approaches for oral cancer -I: Curcumin. Oral Oncology 49: 187-191.[↩]
- Sharifi-Rad J., Rayess Y.E., Rizk A.A., Sadaka C., Zgheib R., Zam W., Sestito S., Rapposelli S., Neffe-Skocińska K., Zielińska D., et al. Turmeric and Its Major Compound Curcumin on Health: Bioactive Effects and Safety Profiles for Food, Pharmaceutical, Biotechnological and Medicinal Applications. Front. Pharmacol. 2020;11:01021. doi: 10.3389/fphar.2020.01021[↩]
- Bigford G.E., Del Rossi G. Supplemental substances derived from foods as adjunctive therapeutic agents for treatment of neurodegenerative diseases and disorders. Adv. Nutr. 2014;5:394–403. doi: 10.3945/an.113.005264[↩]
- WHO FOOD ADDITIVES SERIES: 52. CURCUMIN. https://apps.who.int/iris/bitstream/handle/10665/42849/WHO_TRS_922.pdf;jsessionid=0712A5359CCD928747F01055C86D8E11?sequence=1[↩]
- Zeng L., Yang T., Yang K., Yu G., Li J., Xiang W., Chen H. Efficacy and Safety of Curcumin and Curcuma longa Extract in the Treatment of Arthritis: A Systematic Review and Meta-Analysis of Randomized Controlled Trial. Front. Immunol. 2022;13:891822. doi: 10.3389/fimmu.2022.891822[↩]
- Shep D., Khanwelkar C., Gade P., Karad S. Safety and efficacy of curcumin versus diclofenac in knee osteoarthritis: A randomized open-label parallel-arm study. Trials. 2019;20:214. doi: 10.1186/s13063-019-3327-2[↩]
- Pivari F., Mingione A., Piazzini G., Ceccarani C., Ottaviano E., Brasacchio C., Dei Cas M., Vischi M., Cozzolino M.G., Fogagnolo P., et al. Curcumin Supplementation (Meriva®) Modulates Inflammation, Lipid Peroxidation and Gut Microbiota Composition in Chronic Kidney Disease. Nutrients. 2022;14:231. doi: 10.3390/nu14010231[↩]
- Panahi Y., Kianpour P., Mohtashami R., Jafari R., Simental-Mendía L.E., Sahebkar A. Efficacy and Safety of Phytosomal Curcumin in Non-Alcoholic Fatty Liver Disease: A Randomized Controlled Trial. Drug Res. 2017;67:244–251. doi: 10.1055/s-0043-100019[↩]
- https://www.fda.gov/media/132575/download[↩]
- Cao J, Wang T, Wang M. Investigation of the anti-cataractogenic mechanisms of curcumin through in vivo and in vitro studies. BMC Ophthalmol. 2018 Feb 17;18(1):48. doi: 10.1186/s12886-018-0711-8[↩]
- Mansouri K., Rasoulpoor S., Daneshkhah A., Abolfathi S., Salari N., Mohammadi M., Rasoulpoor S., Shabani S. Clinical effects of curcumin in enhancing cancer therapy: A systematic review. BMC Cancer. 2020;20:791. doi: 10.1186/s12885-020-07256-8[↩]
- Bachmeier B.E., Killian P.H., Melchart D. The role of curcumin in prevention and management of metastatic disease. Int. J. Mol. Sci. 2018;19:1716. doi: 10.3390/ijms19061716[↩]
- Islam M.R., Islam F., Nafady M.H., Akter M., Mitra S., Das R., Urmee H., Shohag S., Akter A., Chidambaram K., et al. Natural small molecules in breast cancer treatment: Understandings from a therapeutic viewpoint. Molecules. 2022;27:2165. doi: 10.3390/molecules27072165[↩]
- Aggarwal, B. B., Deb, L., & Prasad, S. (2014). Curcumin differs from tetrahydrocurcumin for molecular targets, signaling pathways and cellular responses. Molecules (Basel, Switzerland), 20(1), 185–205. https://doi.org/10.3390/molecules20010185[↩]
- Pulido-Moran, M., Moreno-Fernandez, J., Ramirez-Tortosa, C., & Ramirez-Tortosa, M. (2016). Curcumin and Health. Molecules (Basel, Switzerland), 21(3), 264. https://doi.org/10.3390/molecules21030264[↩]
- Jankun J, Wyganowska-Świątkowska M, Dettlaff K, Jelińska A, Surdacka A, Wątróbska-Świetlikowska D, Skrzypczak-Jankun E. Determining whether curcumin degradation/condensation is actually bioactivation (Review). Int J Mol Med. 2016 May;37(5):1151-8. doi: 10.3892/ijmm.2016.2524[↩]
- Hoehle SI, Pfeiffer E, Metzler M. Glucuronidation of curcuminoids by human microsomal and recombinant UDP-glucuronosyltransferases. Mol Nutr Food Res. 2007 Aug;51(8):932-8. doi: 10.1002/mnfr.200600283[↩]
- Prasad S, Tyagi AK, Aggarwal BB. Recent developments in delivery, bioavailability, absorption and metabolism of curcumin: the golden pigment from golden spice. Cancer Res Treat. 2014 Jan;46(1):2-18. doi: 10.4143/crt.2014.46.1.2[↩]
- Tsuda T . Curcumin as a functional food-derived factor: degradation products, metabolites, bioactivity, and future perspectives. Food Funct. 2018 Feb 21;9(2):705-714. doi: 10.1039/c7fo01242j[↩]
- Hoehle SI, Pfeiffer E, Sólyom AM, Metzler M. Metabolism of curcuminoids in tissue slices and subcellular fractions from rat liver. J Agric Food Chem. 2006 Feb 8;54(3):756-64. doi: 10.1021/jf058146a[↩]
- Liu W., Zhai Y., Heng X., Che F.Y., Chen W., Sun D., Zhai G. Oral bioavailability of curcumin: Problems and advancements. J. Drug Target. 2016;24:694–702. doi: 10.3109/1061186X.2016.1157883[↩]
- Metzler M., Pfeiffer E., Schulz S.I., Dempe J.S. Curcumin uptake and metabolism. Biofactors. 2013;39:14–20. doi: 10.1002/biof.1042[↩]
- Vareed S.K., Kakarala M., Ruffin M.T., Crowell J.A., Normolle D.P., Djuric Z., Brenner D.E. Pharmacokinetics of curcumin conjugate metabolites in healthy human subjects. Cancer Epidemiol. Biomark. Prev. 2008;17:1411–1417. doi: 10.1158/1055-9965.EPI-07-2693[↩]
- Pfeiffer E, Hoehle SI, Walch SG, Riess A, Sólyom AM, Metzler M. Curcuminoids form reactive glucuronides in vitro. J Agric Food Chem. 2007 Jan 24;55(2):538-44. doi: 10.1021/jf0623283[↩]
- Huang Y, Cao S, Zhang Q, Zhang H, Fan Y, Qiu F, Kang N. Biological and pharmacological effects of hexahydrocurcumin, a metabolite of curcumin. Arch Biochem Biophys. 2018 May 15;646:31-37. doi: 10.1016/j.abb.2018.03.030[↩]
- Dempe JS, Scheerle RK, Pfeiffer E, Metzler M. Metabolism and permeability of curcumin in cultured Caco-2 cells. Mol Nutr Food Res. 2013 Sep;57(9):1543-9. doi: 10.1002/mnfr.201200113[↩]
- Metzler M, Pfeiffer E, Schulz SI, Dempe JS. Curcumin uptake and metabolism. Biofactors. 2013 Jan-Feb;39(1):14-20. doi: 10.1002/biof.1042[↩]
- Dempe JS, Pfeiffer E, Grimm AS, Metzler M. Metabolism of curcumin and induction of mitotic catastrophe in human cancer cells. Mol Nutr Food Res. 2008 Sep;52(9):1074-81. doi: 10.1002/mnfr.200800029[↩]
- de Oliveira MR, Jardim FR, Setzer WN, Nabavi SM, Nabavi SF. Curcumin, mitochondrial biogenesis, and mitophagy: Exploring recent data and indicating future needs. Biotechnol Adv. 2016 Sep-Oct;34(5):813-826. doi: 10.1016/j.biotechadv.2016.04.004[↩]
- Lou Y, Zheng J, Hu H, Lee J, Zeng S. Application of ultra-performance liquid chromatography coupled with quadrupole time-of-flight mass spectrometry to identify curcumin metabolites produced by human intestinal bacteria. J Chromatogr B Analyt Technol Biomed Life Sci. 2015 Mar 15;985:38-47. doi: 10.1016/j.jchromb.2015.01.014[↩]
- An C.-Y., Sun Z.-Z., Shen L., Ji H.-F. Biotransformation of food spice curcumin by gut bacterium Bacillus megaterium DCMB-002 and its pharmacological implications. Food Nutr. Res. 2017;61 doi: 10.1080/16546628.2017.1412814[↩]
- Burapan S, Kim M, Han J. Curcuminoid Demethylation as an Alternative Metabolism by Human Intestinal Microbiota. J Agric Food Chem. 2017 Apr 26;65(16):3305-3310. doi: 10.1021/acs.jafc.7b00943[↩]
- Xu, X. Y., Meng, X., Li, S., Gan, R. Y., Li, Y., & Li, H. B. (2018). Bioactivity, Health Benefits, and Related Molecular Mechanisms of Curcumin: Current Progress, Challenges, and Perspectives. Nutrients, 10(10), 1553. https://doi.org/10.3390/nu10101553[↩][↩]
- Stahl W, Sies H. Lycopene: a biologically important carotenoid for humans? Arch Biochem Biophys. 1996 Dec 1;336(1):1-9. doi: 10.1006/abbi.1996.0525[↩]
- Rao AV, Ray MR, Rao LG. Lycopene. Adv Food Nutr Res. 2006;51:99-164. https://doi.org/10.1016/S1043-4526(06)51002-2[↩]
- Najm W, Lie D. Dietary supplements commonly used for prevention. Prim Care. 2008 Dec;35(4):749-67. doi: 10.1016/j.pop.2008.07.010[↩]
- Przybylska S. Lycopene—A Bioactive Carotenoid Offering Multiple Health Benefits: A Review. Int. J. Food Sci. Technol. 2020;55:11–32. doi: 10.1111/ijfs.14260[↩]
- NCI Thesaurus. https://ncit.nci.nih.gov/ncitbrowser/ConceptReport.jsp?dictionary=NCI_Thesaurus&ns=NCI_Thesaurus&code=C2226[↩]
- Magri V, Trinchieri A, Perletti G, Marras E. Activity of Serenoa repens, lycopene and selenium on prostatic disease: evidences and hypotheses. Arch Ital Urol Androl. 2008 Jun;80(2):65-78.[↩]
- Woodside J.V., McGrath A.J., Lyner N., McKinley M.C. Carotenoids and Health in Older People. Maturitas. 2015;80:63–68. doi: 10.1016/j.maturitas.2014.10.012[↩]
- Clinton SK. Lycopene: chemistry, biology, and implications for human health and disease. Nutr Rev. 1998 Feb;56(2 Pt 1):35-51. doi: 10.1111/j.1753-4887.1998.tb01691.x[↩]
- Kopec R, Schwartz SJ, Hadley C: Lycopene. In: Coates PM, Betz JM, Blackman MR, et al., eds.: Encyclopedia of Dietary Supplements. 2nd ed. Informa Healthcare, 2010, pp 504-17.[↩]
- Bin-Jumah M.N., Nadeem M.S., Gilani S.J., Mubeen B., Ullah I., Alzarea S.I., Ghoneim M.M., Alshehri S., Al-Abbasi F.A., Kazmi I. Lycopene: A Natural Arsenal in the War against Oxidative Stress and Cardiovascular Diseases. Antioxidants. 2022;11:232. doi: 10.3390/antiox11020232[↩][↩]
- Grabowska M., Wawrzyniak D., Rolle K., Chomczyński P., Oziewicz S., Jurga S., Barciszewski J. Let Food Be Your Medicine: Nutraceutical Properties of Lycopene. Food Funct. 2019;10:3090–3102. doi: 10.1039/C9FO00580C[↩]
- Imran M., Ghorat F., Ul-Haq I., Ur-Rehman H., Aslam F., Heydari M., Shariati M.A., Okuskhanova E., Yessimbekov Z., Thiruvengadam M., et al. Lycopene as a Natural Antioxidant Used to Prevent Human Health Disorders. Antioxidants. 2020;9:706. doi: 10.3390/antiox9080706[↩]
- Chen J., Cao X., Huang Z., Chen X., Zou T., You J. Research Progress on Lycopene in Swine and Poultry Nutrition: An Update. Animals. 2023;13:883. doi: 10.3390/ani13050883[↩]
- Story E.N., Kopec R.E., Schwartz S.J., Harris G.K. An uptade on the health effects of tomato lycopene. Annu. Rev. Food Sci. Technol. 2010;1:189–210. doi: 10.1146/annurev.food.102308.124120[↩]
- Petyaev I.M. Lycopene Deficiency in Ageing and Cardiovascular Disease. Oxidat. Med. Cell. Longev. 2016;3:1–6. doi: 10.1155/2016/3218605[↩]
- Pataro G., Carullo D., Falcone M., Ferrari G. Recovery of lycopene from industrially derived tomato processing by-products by pulsed electric fields-assisted extraction. Innovative Food Sci Emerging Technol. 2020;63[↩]
- Food and Nutrition Board, Institute of Medicine. Beta-carotene and other carotenoids. Dietary reference intakes for vitamin C, vitamin E, selenium, and carotenoids. Washington, D.C.: National Academy Press; 2000:325-400. https://nap.nationalacademies.org/read/9810/chapter/10[↩][↩][↩][↩][↩]
- van Breemen RB, Pajkovic N. Multitargeted therapy of cancer by lycopene. Cancer Lett. 2008 Oct 8;269(2):339-51. doi: 10.1016/j.canlet.2008.05.016[↩][↩]
- Stahl W, Junghans A, de Boer B, Driomina ES, Briviba K, Sies H. Carotenoid mixtures protect multilamellar liposomes against oxidative damage: synergistic effects of lycopene and lutein. FEBS Lett. 1998 May 8;427(2):305-8. doi: 10.1016/s0014-5793(98)00434-7[↩]
- Di Mascio P, Kaiser S, Sies H. Lycopene as the most efficient biological carotenoid singlet oxygen quencher. Arch Biochem Biophys. 1989 Nov 1;274(2):532-8. doi: 10.1016/0003-9861(89)90467-0[↩]
- Rao A.V., Shen H.L. Effect of low dose lycopene intake on lycopene bioavailability and oxidative stress. Nutr Res. 2002;22(10):1125–1131.[↩]
- Torregrosa-Crespo J, Montero Z, Fuentes JL, Reig García-Galbis M, Garbayo I, Vílchez C, Martínez-Espinosa RM. Exploring the Valuable Carotenoids for the Large-Scale Production by Marine Microorganisms. Mar Drugs. 2018 Jun 8;16(6):203. doi: 10.3390/md16060203[↩]
- Prathibha G., Yadav V. Lycopene: a plant pigment with prominent role on human health. Int J Curr Res. 2015;6(6):7006–7010.[↩]
- Cheng J, Miller B, Balbuena E, Eroglu A. Lycopene Protects against Smoking-Induced Lung Cancer by Inducing Base Excision Repair. Antioxidants (Basel). 2020 Jul 21;9(7):643. doi: 10.3390/antiox9070643[↩]
- McCormack T, Dent R, Blagden M. Very low LDL-C levels may safely provide additional clinical cardiovascular benefit: the evidence to date. Int J Clin Pract. 2016 Nov;70(11):886-897. doi: 10.1111/ijcp.12881[↩]
- Palozza P, Catalano A, Simone R, Cittadini A. Lycopene as a guardian of redox signalling. Acta Biochim Pol. 2012;59(1):21-5.[↩]
- Zhao Y, Xin Z, Li N, Chang S, Chen Y, Geng L, Chang H, Shi H, Chang YZ. Nano-liposomes of lycopene reduces ischemic brain damage in rodents by regulating iron metabolism. Free Radic Biol Med. 2018 Aug 20;124:1-11. doi: 10.1016/j.freeradbiomed.2018.05.082[↩]
- Van Breemen RB, Pajkovic N. Multitargeted therapy of cancer by lycopene. Cancer letters. 2008;269(2):339-351. doi:10.1016/j.canlet.2008.05.016. https://www.ncbi.nlm.nih.gov/pmc/articles/PMC2615641/[↩]
- Mordente A, Guantario B, Meucci E, Silvestrini A, Lombardi E, Martorana GE, Giardina B, Böhm V. Lycopene and cardiovascular diseases: an update. Curr Med Chem. 2011;18(8):1146-63. doi: 10.2174/092986711795029717[↩][↩]
- Human Metabolome Database. Lycopene. http://www.hmdb.ca/metabolites/HMDB03000[↩]
- U.S. National Library of Medicine. Medline Plus. Lycopene. https://medlineplus.gov/druginfo/natural/554.html[↩]
- Stahl W, Schwarz W, Sundquist AR, Sies H. cis-trans isomers of lycopene and beta-carotene in human serum and tissues. Arch Biochem Biophys. 1992 Apr;294(1):173-7. doi: 10.1016/0003-9861(92)90153-n[↩]
- Wang YH, Zhang RR, Yin Y, Tan GF, Wang GL, Liu H, Zhuang J, Zhang J, Zhuang FY, Xiong AS. Advances in engineering the production of the natural red pigment lycopene: A systematic review from a biotechnology perspective. J Adv Res. 2023 Apr;46:31-47. doi: 10.1016/j.jare.2022.06.010[↩][↩]
- Zhang L.F., Zhang H.W., Ndeurumi K.H., Parkin K.L., Venuste M. Thermally-induced geometrical isomerisation of lycopene and its potential influence on functional activity. Food Chem. 2012;132(4):2112–2117.[↩][↩]
- Lemmens L., Colle I., Van Buggenhout S., Palmero P., Loey A.V., Hendrickx M. Carotenoid bioaccessibility in fruit- and vegetable-based food products as affected by product (micro) structural characteristics and the presence of lipids: A review. Trends Food Sci Technol. 2014;38(2):125–135.[↩]
- Liang X.P., Ma C.C., Yan X.J., Liu X., Liu F. Advances in research on bioactivity, metabolism, stability and delivery systems of lycopene. Trends Food Sci Technol. 2019;93:185–196.[↩][↩]
- Srivastava S., Srivastava A.K. Lycopene; chemistry, biosynthesis, metabolism and degradation under various abiotic parameters. J Food Sci Technol. 2015;52(1):41–53.[↩][↩]
- Moran NE, Erdman JW Jr, Clinton SK. Complex interactions between dietary and genetic factors impact lycopene metabolism and distribution. Arch Biochem Biophys. 2013 Nov 15;539(2):171-80. doi: 10.1016/j.abb.2013.06.017[↩][↩]
- Mehta N., Patani P., Singhvi I. A review on tomato lycopene. Int. J. Pharm. Sci. Res. 2018;9:916–923. doi: 10.13040/IJPSR.0975-8232.9(3).916-23[↩][↩]
- Arab L, Steck S. Lycopene and cardiovascular disease. Am J Clin Nutr. 2000 Jun;71(6 Suppl):1691S-5S; discussion 1696S-7S. doi: 10.1093/ajcn/71.6.1691S[↩]
- Khan N, Afaq F, Mukhtar H. Cancer chemoprevention through dietary antioxidants: progress and promise. Antioxid Redox Signal. 2008 Mar;10(3):475-510. doi: 10.1089/ars.2007.1740[↩]
- Ilic D, Forbes KM, Hassed C. Lycopene for the prevention of prostate cancer. Cochrane Database Syst Rev. 2011 Nov 9;2011(11):CD008007. doi: 10.1002/14651858.CD008007.pub2[↩][↩]
- Kavanaugh CJ, Trumbo PR, Ellwood KC. The U.S. Food and Drug Administration’s evidence-based review for qualified health claims: tomatoes, lycopene, and cancer. J Natl Cancer Inst. 2007 Jul 18;99(14):1074-85. doi: 10.1093/jnci/djm037[↩]
- Kumar V. N. P, Elango P, Asmathulla S, kavimani S. A Systematic Review on Lycopene and its Beneficial Effects”. Biomed Pharmacol J 2017;10(4). https://biomedpharmajournal.org/vol10no4/a-systematic-review-on-lycopene-and-its-beneficial-effects[↩]
- Schierle J., Bretzel W., Bühler I., Faccin N., Hess D., Steiner K., Schüep W. Content and isomeric ratio of lycopene in food and human blood plasma. Food Chem. 1997;59:459–465. doi: 10.1016/S0308-8146(96)00177-X[↩]
- Walfisch Y., Walfisch S., Agbaria R., Levy J., Sharoni Y. Lycopene in serum, skin and adipose tissues after tomato-oleoresin supplementation in patients undergoing haemorrhoidectomy or peri-anal fistulotomy. Br. J. Nutr. 2003;90:759–766. doi: 10.1079/BJN2003955[↩]
- Kessy H.N., Zhang L., Zhang H. Lycopene (Z)—Isomers Enrichment and Separation. Int. J. Food Sci. Technol. 2013;48:2050–2056. doi: 10.1111/ijfs.12183[↩]
- Carotenoids. https://lpi.oregonstate.edu/mic/dietary-factors/phytochemicals/carotenoids[↩][↩][↩]
- Kelly M.E., Ramkumar S., Sun W., Colon Ortiz C., Kiser P.D., Golczak M., von Lintig J. The Biochemical Basis of Vitamin A Production from the Asymmetric Carotenoid β-Cryptoxanthin. ACS Chem. Biol. 2018;13:2121–2129. doi: 10.1021/acschembio.8b00290[↩]
- Britton G. Structure and properties of carotenoids in relation to function. FASEB J. 1995 Dec;9(15):1551-8.[↩]
- Yang J, Zhang Y, Na X, Zhao A. β-Carotene Supplementation and Risk of Cardiovascular Disease: A Systematic Review and Meta-Analysis of Randomized Controlled Trials. Nutrients. 2022 Mar 18;14(6):1284. doi: 10.3390/nu14061284[↩]
- Shankaranarayanan J, Arunkanth K, Dinesh K C. Beta Carotene -Therapeutic Potential and Strategies to Enhance Its Bioavailability. Nutri Food Sci Int J. 2018; 7(4): 555716. DOI: 10.19080/NFSIJ.2018.07.555716[↩][↩]
- Institute of Medicine. Food and Nutrition Board. Dietary Reference Intakes for Vitamin C, Vitamin E, Selenium, and Carotenoids. Washington, DC: National Academy Press; 2000. https://nap.nationalacademies.org/read/9810/chapter/1[↩]
- Cooperstone JL, Schwartz SJ. Recent insights into health benefits of carotenoids. Handbook on natural pigments in food and beverages. 2016. Jan 1:473–97.[↩]
- Badr AM, Shabana EF, Senousy HH, Mohammad HY. Anti-inflammatory and anti-cancer effects of β-carotene, extracted from Dunaliella bardawil by milking. J. Food Agric. Environ. 2014. Jul;12(3):24–31.[↩]
- Donhowe E.G., Kong F. Beta-carotene: Digestion, microencapsulation, and in vitro bioavailability. Food Bioprocess Technol. 2014;7:338–354. doi: 10.1007/s11947-013-1244-z[↩]
- Weber D, Grune T. The contribution of β-carotene to vitamin A supply of humans. Mol Nutr Food Res. 2012 Feb;56(2):251-8. doi: 10.1002/mnfr.201100230[↩]
- Omenn GS, Goodman GE, Thornquist MD, Balmes J, Cullen MR, Glass A, Keogh JP, Meyskens FL Jr, Valanis B, Williams JH Jr, Barnhart S, Cherniack MG, Brodkin CA, Hammar S. Risk factors for lung cancer and for intervention effects in CARET, the Beta-Carotene and Retinol Efficacy Trial. J Natl Cancer Inst. 1996 Nov 6;88(21):1550-9. doi: 10.1093/jnci/88.21.1550[↩]
- Kamangar F, Qiao YL, Yu B, Sun XD, Abnet CC, Fan JH, Mark SD, Zhao P, Dawsey SM, Taylor PR. Lung cancer chemoprevention: a randomized, double-blind trial in Linxian, China. Cancer Epidemiol Biomarkers Prev. 2006 Aug;15(8):1562-4. doi: 10.1158/1055-9965.EPI-06-0316[↩]
- Veeramachaneni S, Wang XD. Carotenoids and lung cancer prevention. Front Biosci (Schol Ed). 2009 Jun 1;1(1):258-74. doi: 10.2741/S25[↩]
- Mayne ST, Ferrucci LM, Cartmel B. Lessons learned from randomized clinical trials of micronutrient supplementation for cancer prevention. Annu Rev Nutr. 2012 Aug 21;32:369-90. doi: 10.1146/annurev-nutr-071811-150659[↩]
- Moyer VA; U.S. Preventive Services Task Force. Vitamin, mineral, and multivitamin supplements for the primary prevention of cardiovascular disease and cancer: U.S. Preventive services Task Force recommendation statement. Ann Intern Med. 2014 Apr 15;160(8):558-64. doi: 10.7326/M14-0198[↩]
- Verlaet AAJ, Ceulemans B, Verhelst H, et al. Effect of Pycnogenol® on attention-deficit hyperactivity disorder (ADHD): study protocol for a randomised controlled trial. Trials. 2017;18:145. doi:10.1186/s13063-017-1879-6. https://www.ncbi.nlm.nih.gov/pmc/articles/PMC5370458/[↩]
- Oliff H. Scientific and clinical monograph for Pycnogenol. Austin, TX: American Botanical Council; (2019). p. 1–46.[↩]
- Lee EL, Barnes J. Pine bark. J Prim Health Care. 2023 Jun;15(2):192-194. doi: 10.1071/HC23064[↩]
- Nattagh-Eshtivani E, Gheflati A, Barghchi H, Rahbarinejad P, Hachem K, Shalaby MN, et al. The role of Pycnogenol in the control of inflammation and oxidative stress in chronic diseases: Molecular aspects. Phytother Res. (2022) 36:2352–74. 10.1002/ptr.7454[↩]
- D’Andrea G. Pycnogenol: A blend of procyanidins with multifaceted therapeutic applications? Fitoterapia. (2010) 81:724–36. 10.1016/j.fitote.2010.06.011[↩]
- Grimm T, Skrabala R, Chovanova Z, Muchova J, Sumegova K, Liptakova A, et al. Single and multiple dose pharmacokinetics of maritime pine bark extract (pycnogenol) after oral administration to healthy volunteers. BMC Clin Pharmacol. 2006;6:4. doi: 10.1186/1472-6904-6-4. https://www.ncbi.nlm.nih.gov/pmc/articles/PMC1559639/[↩]
- Rohdewald P. A review of the French maritime pine bark extract (Pycnogenol), a herbal medication with a diverse clinical pharmacology. Int J Clin Pharmacol Ther. 2002;40:158–168.[↩]
- A review of the French maritime pine bark extract (Pycnogenol), a herbal medication with a diverse clinical pharmacology. Rohdewald P. Int J Clin Pharmacol Ther. 2002 Apr; 40(4):158-68.[↩]
- Rohdewald PA. Review of the French maritime pine bark extract (Pycnogenol), a herbal medication with a diverse clinical pharmacology. Int J Clin Pharmacol Ther. 2002;40:158–168. doi: 10.5414/CPP40158.[↩]
- Uhlenhut K, Högger P. Facilitated cellular uptake and suppression of inducible nitric oxide synthase by a metabolite of maritime pine bark extract (Pycnogenol) Free Radic Biol Med. 2012;53:305–313. doi: 10.1016/j.freeradbiomed.2012.04.013[↩]
- Cho KJ, Yun CH, Yoon DY, Cho YS, Rimbach G, Packer L, et al. Effect of bioflavonoids extracted from the bark of Pinus Maritima on proinflammatory cytokine interleukin-1 production in lipopolysaccharide-stimulated RAW 264.7. Toxicol Appl Pharmacol. 2000;168:64–71. doi: 10.1006/taap.2000.9001[↩]
- Peng YJ, Lee CH, Wang CC, Salter DM, Lee HS. Pycnogenol attenuates the inflammatory and nitrosative stress on joint inflammation induced by urate crystals. Free Radic Biol Med. 2012;52:765–774. doi: 10.1016/j.freeradbiomed.2011.12.003[↩]
- A review of the French maritime pine bark extract (Pycnogenol), a herbal medication with a diverse clinical pharmacology. Int J Clin Pharmacol Ther. 2002 Apr;40(4):158-68. https://www.ncbi.nlm.nih.gov/pubmed/11996210/[↩][↩][↩][↩][↩][↩][↩][↩]
- Treatment of osteoarthritis with Pycnogenol. The SVOS (San Valentino Osteo-arthrosis Study). Evaluation of signs, symptoms, physical performance and vascular aspects. Belcaro G, Cesarone MR, Errichi S, Zulli C, Errichi BM, Vinciguerra G, Ledda A, Di Renzo A, Stuard S, Dugall M, Pellegrini L, Errichi S, Gizzi G, Ippolito E, Ricci A, Cacchio M, Cipollone G, Ruffini I, Fano F, Hosoi M, Rohdewald P. Phytother Res. 2008 Apr; 22(4):518-23.[↩]
- National Center for Biotechnology Information, U.S. National Library of Medicine. Lutein. https://pubchem.ncbi.nlm.nih.gov/compound/Lutein[↩]
- Holden J.M., Eldridge A.L., Beecher G.R., Marilyn Buzzard I., Bhagwat S., Davis C.S., Douglass L.W., Gebhardt S., Haytowitz D., Schakel S. Carotenoid Content of U.S. Foods: An Update of the Database. J. Food Compos. Anal. 1999;12:169–196. doi: 10.1006/jfca.1999.0827[↩]
- Am J Clin Nutr February 2016, vol. 103 no. 2 481-494. The effects of lutein on cardiometabolic health across the life course: a systematic review and meta-analysis. http://ajcn.nutrition.org/content/103/2/481.long[↩]
- Khachik F., Spangler C.J., Smith J.C., Canfield L.M., Steck A., Pfander H. Identification, Quantification, and Relative Concentrations of Carotenoids and Their Metabolites in Human Milk and Serum. Anal. Chem. 1997;69:1873–1881. doi: 10.1021/ac961085i[↩]
- Canadian Institutes of Health Research. DrugBank. Lutein. https://www.drugbank.ca/drugs/DB00137[↩][↩][↩][↩]
- Stahl W. Macular Carotenoids: Lutein and Zeaxanthin. Dev. Ophthalmol. 2005;38:70–88. doi: 10.1159/000082768[↩]
- Widomsa J., Zareba M., Subczynski W.K. Can Xanthophyll-Membrane Interactions Explain Their Selective Presence in the Retina and the Brain? Foods. 2016;5:7. doi: 10.3390/foods5010007[↩]
- Böhm V., Lietz G., Olmedilla-Alonso B., Phelan D., Reboul E., Bánati D., Borel P., Corte-Real J., De Lera A.R., Desmarchelier C., et al. From Carotenoid Intake to Carotenoid Blood and Tissue Concentrations-Implications for Dietary Intake Recommendations. Nutr. Rev. 2021;79:544–573. doi: 10.1093/nutrit/nuaa008[↩]
- Yakovleva M.A., Panova I.G., Fel’dman T.B., Zak P.P., Tatikolov A.S., Sukhikh G.T., Ostrovsky M.A. Finding of Carotenoids in the Vitreous Body of Human Eye during Prenatal Development. Russ. J. Dev. Biol. 2007;38:317–321. doi: 10.1134/S1062360407050062[↩]
- Krinsky NI, Landrum JT, Bone RA. Biologic mechanisms of the protective role of lutein and zeaxanthin in the eye. Annu Rev Nutr. 2003;23:171-201. doi: 10.1146/annurev.nutr.23.011702.073307[↩]
- Kijlstra A, Tian Y, Kelly ER, Berendschot TT. Lutein: more than just a filter for blue light. Prog Retin Eye Res. 2012 Jul;31(4):303-15. doi: 10.1016/j.preteyeres.2012.03.002[↩]
- Whitehead AJ, Mares JA, Danis RP. Macular pigment: a review of current knowledge. Arch Ophthalmol. 2006 Jul;124(7):1038-45. doi: 10.1001/archopht.124.7.1038[↩]
- Bernstein P.S., Li B., Vachali P.P., Gorusupudi A., Shyam R., Henriksen B.S., Nolan J.M. Lutein, Zeaxanthin, and Meso-Zeaxanthin: The Basic and Clinical Science Underlying Carotenoid-Based Nutritional Interventions against Ocular Disease. Prog. Retin. Eye Res. 2016;50:34–66. doi: 10.1016/j.preteyeres.2015.10.003[↩]
- Wooten B.R., Hammond B.R. Macular Pigment: Influences on Visual Acuity and Visibility. Prog. Retin. Eye Res. 2002;21:225–240. doi: 10.1016/S1350-9462(02)00003-4[↩]
- Bernstein P.S., Delori F.C., Richer S., van Kuijk F.J.M., Wenzel A.J. The Value of Measurement of Macular Carotenoid Pigment Optical Densities and Distributions in Age-Related Macular Degeneration and Other Retinal Disorders. Vision Res. 2010;50:716–728. doi: 10.1016/j.visres.2009.10.014[↩][↩][↩]
- Hammond B.R., Fletcher L.M., Roos F., Wittwer J., Schalch W. A Double-Blind, Placebo-Controlled Study on the Effects of Lutein and Zeaxanthin on Photostress Recovery, Glare Disability, and Chromatic Contrast. Investig. Ophthalmol. Vis. Sci. 2014;55:8583–8589. doi: 10.1167/iovs.14-15573[↩][↩][↩]
- Stringham J.M., Hammond B.R. The Glare Hypothesis of Macular Pigment Function. Optom. Vis. Sci. 2007;84:859–864. doi: 10.1097/OPX.0b013e3181559c2b[↩]
- Loughman J., Nolan J.M., Howard A.N., Connolly E., Meagher K., Beatty S. The Impact of Macular Pigment Augmentation on Visual Performance Using Different Carotenoid Formulations. Investig. Ophthalmol. Vis. Sci. 2012;53:7871–7880. doi: 10.1167/iovs.12-10690[↩]
- Kvansakul J, Rodriguez-Carmona M, Edgar DF, Barker FM, Köpcke W, Schalch W, Barbur JL. Supplementation with the carotenoids lutein or zeaxanthin improves human visual performance. Ophthalmic Physiol Opt. 2006 Jul;26(4):362-71. doi: 10.1111/j.1475-1313.2006.00387.x[↩]
- Ma L, Lin XM, Zou ZY, Xu XR, Li Y, Xu R. A 12-week lutein supplementation improves visual function in Chinese people with long-term computer display light exposure. Br J Nutr. 2009 Jul;102(2):186-90. doi: 10.1017/S0007114508163000[↩]
- Stringham JM, Hammond BR. Macular pigment and visual performance under glare conditions. Optom Vis Sci. 2008 Feb;85(2):82-8. doi: 10.1097/OPX.0b013e318162266e. Erratum in: Optom Vis Sci. 2008 Apr;85(4):285.[↩]
- Yagi A, Fujimoto K, Michihiro K, Goh B, Tsi D, Nagai H. The effect of lutein supplementation on visual fatigue: a psychophysiological analysis. Appl Ergon. 2009 Nov;40(6):1047-54. doi: 10.1016/j.apergo.2009.04.013[↩]
- Stringham JM, Hammond BR Jr. Dietary lutein and zeaxanthin: possible effects on visual function. Nutr Rev. 2005 Feb;63(2):59-64. doi: 10.1111/j.1753-4887.2005.tb00122.x[↩]
- National Center for Biotechnology Information, U.S. National Library of Medicine. PubChem. Lutein. https://pubchem.ncbi.nlm.nih.gov/compound/Lutein[↩]
- Chew E.Y., Clemons T.E., SanGiovanni J.P., Danis R.P., Ferris F.L., Elman M.J., Antoszyk A.N., Ruby A.J., Orth D., Bressler S.B., et al. Secondary Analyses of the Effects of Lutein/Zeaxanthin on Age-Related Macular Degeneration Progression AREDS2 Report No. 3. JAMA Ophthalmol. 2014;132:142–149. doi: 10.1001/jamaophthalmol.2013.7376[↩][↩]
- Liu R., Wang T., Zhang B., Qin L., Wu C., Li Q., Ma L. Lutein and Zeaxanthin Supplementation and Association with Visual Function in Age-Related Macular Degeneration. Investig. Ophthalmol. Vis. Sci. 2015;56:252–258. doi: 10.1167/iovs.14-15553[↩][↩]
- Dawczynski J., Jentsch S., Schweitzer D., Hammer M., Lang G.E., Strobel J. Long Term Effects of Lutein, Zeaxanthin and Omega-3-LCPUFAs Supplementation on Optical Density of Macular Pigment in AMD Patients: The LUTEGA Study. Graefe’s Arch. Clin. Exp. Ophthalmol. 2013;251:2711–2723. doi: 10.1007/s00417-013-2376-6[↩]
- Chew E.Y., Clemons T.E., Keenan T.D.L., Agron E., Malley C.E., Domalpally A. The Results of the 10 Year Follow-on Study of the Age-Related Eye Disease Study 2 (AREDS2) Investig. Ophthalmol. Vis. Sci. 2001;62:1215.[↩]
- Stringham J.M., Hammond B.R. Macular Pigment and Visual Performance under Glare Conditions. Optom. Vis. Sci. 2008;85:82–88. doi: 10.1097/OPX.0b013e318162266e[↩]
- Stringham J.M., O’Brien K.J., Stringham N.T. Macular Carotenoid Supplementation Improves Disability Glare Performance and Dynamics of Photostress Recovery. Eye Vis. 2016;3:1–8. doi: 10.1186/s40662-016-0060-8[↩][↩]
- Johnson E.J., McDonald K., Caldarella S.M., Chung H.Y., Troen A.M., Snodderly D.M. Cognitive Findings of an Exploratory Trial of Docosahexaenoic Acid and Lutein Supplementation in Older Women. Nutr. Neurosci. 2008;11:75–83. doi: 10.1179/147683008X301450[↩][↩]
- Hammond B.R., Stephen Miller L., Bello M.O., Lindbergh C.A., Mewborn C., Renzi-Hammond L.M. Effects of Lutein/Zeaxanthin Supplementation on the Cognitive Function of Community Dwelling Older Adults: A Randomized, Double-Masked, Placebo-Controlled Trial. Front. Aging Neurosci. 2017;9:1–9. doi: 10.3389/fnagi.2017.00254[↩]
- Lindbergh C.A., Renzi-Hammond L.M., Hammond B.R., Terry D.P., Mewborn C.M., Puente A.N., Miller L.S. Lutein and Zeaxanthin Influence Brain Function in Older Adults: A Randomized Controlled Trial. J. Int. Neuropsychol. Soc. 2018;24:77–90. doi: 10.1017/S1355617717000534[↩][↩]
- Renzi-Hammond L.M., Bovier E.R., Fletcher L.M., Miller L.S., Mewborn C.M., Lindbergh C.A., Baxter J.H., Hammond B.R. Effects of a Lutein and Zeaxanthin Intervention on Cognitive Function: A Randomized, Double-Masked, Placebo-Controlled Trial of Younger Healthy Adults. Nutrients. 2017;9:1246. doi: 10.3390/nu9111246[↩][↩]
- Bernstein P.S., Arunkumar R. The Emerging Roles of the Macular Pigment Carotenoids throughout the Lifespan and in Prenatal Supplementation. J. Lipid Res. 2021;62:1–33. doi: 10.1194/jlr.TR120000956[↩]
- Vishwanathan R., Kuchan M.J., Sen S., Johnson E.J. Lutein and preterm infants with decreased concentrations of brain carotenoids. J. Pediatr. Gastroenterol. Nutr. 2014;59:659–665. doi: 10.1097/MPG.0000000000000389[↩]
- Bernstein P.S., Sharifzadeh M., Liu A., Ermakov I., Nelson K., Sheng X., Panish C., Carlstrom B., Hoffman R.O., Gellermann W. Blue-light reflectance imaging of macular pigment in infants and children. Investig. Ophthalmol. Vis. Sci. 2013;54:4034–4040. doi: 10.1167/iovs.13-11891[↩]
- Bettler J., Zimmer J.P., Neuringer M., DeRusso P.A. Serum lutein concentrations in healthy term infants fed human milk or infant formula with lutein. Eur. J. Nutr. 2010;49:45–51. doi: 10.1007/s00394-009-0047-5[↩]
- Johnson L., Norkus E., Abbasi S., Gerdes J., Bhutani V. Contribution of beta-carotene (BC) from BC enriched formulae to individual and total serum carotenoids in term infants. FASEB J. 1995;9:1869.[↩][↩]
- Gazzolo D, Picone S, Gaiero A, Bellettato M, Montrone G, Riccobene F, Lista G, Pellegrini G. Early Pediatric Benefit of Lutein for Maturing Eyes and Brain-An Overview. Nutrients. 2021 Sep 17;13(9):3239. doi: 10.3390/nu13093239[↩][↩]
- Zhang Y, Kong L, Lawrence JC, Tan L. Utilization of Biopolymer-Based Lutein Emulsion as an Effective Delivery System to Improve Lutein Bioavailability in Neonatal Rats. Nutrients. 2024 Jan 31;16(3):422. doi: 10.3390/nu16030422[↩]
- Johnson E.J., Vishwanathan R., Johnson M.A., Hausman D.B., Davey A., Scott T.M., Green R.C., Miller L.S., Gearing M., Woodard J., et al. Relationship between Serum and Brain Carotenoids, α -Tocopherol, and Retinol Concentrations and Cognitive Performance in the Oldest Old from the Georgia Centenarian Study. J. Aging Res. 2013;2013 doi: 10.1155/2013/951786[↩]
- Liu X.H., Yu R.B., Liu R., Hao Z.X., Han C.C., Zhu Z.H., Ma L. Association between Lutein and Zeaxanthin Status and the Risk of Cataract: A Meta-Analysis. Nutrients. 2014;6:452–465. doi: 10.3390/nu6010452[↩]
- Lindbergh C.A., Mewborn C.M., Hammond B.R., Renzi-Hammond L.M., Curran-Celentano J.M., Miller L.S. Relationship of Lutein and Zeaxanthin Levels to Neurocognitive Functioning: An FMRI Study of Older Adults. J. Int. Neuropsychol. Soc. 2017;23:11–22. doi: 10.1017/S1355617716000850[↩]
- Bovier E.R., Hammond B.R. A Randomized Placebo-Controlled Study on the Effects of Lutein and Zeaxanthin on Visual Processing Speed in Young Healthy Subjects. Arch. Biochem. Biophys. 2015;572:54–57. doi: 10.1016/j.abb.2014.11.012[↩]
- Bovier E.R., Renzi L.M., Hammond B.R. A Double-Blind, Placebo-Controlled Study on the Effects of Lutein and Zeaxanthin on Neural Processing Speed and Efficiency. PLoS ONE. 2014;9:e108178. doi: 10.1371/journal.pone.0108178[↩]
- Lai J.S., Veetil V.O., Lanca C., Lee B.L., Godfrey K.M., Gluckman P.D., Shek L.P., Yap F., Tan K.H., Chong Y.S., et al. Maternal Lutein and Zeaxanthin Concentrations in Relation to Offspring Visual Acuity at 3 Years of Age: The GUSTO Study. Nutrients. 2020;12:274. doi: 10.3390/nu12020274[↩]
- Mahmassani H.A., Switkowski K.M., Scott T.M., Johnson E.J., Rifas-Shiman S.L., Oken E., Jacques P.F. Maternal Intake of Lutein and Zeaxanthin during Pregnancy Is Positively Associated with Offspring Verbal Intelligence and Behavior Regulation in Mid-Childhood in the Project Viva Cohort. J. Nutr. 2021;151:615–627. doi: 10.1093/jn/nxaa348[↩]
- Cheatham C.L., Sheppard K.W. Synergistic Effects of Human Milk Nutrients in the Support of Infant Recognition Memory: An Observational Study. Nutrients. 2015;7:9079–9095. doi: 10.3390/nu7115452[↩]
- Barnett S.M., Khan N.A., Walk A.M., Raine L.B., Moulton C., Cohen N.J., Kramer A.F., Hammond B.R., Renzi-Hammond L., Hillman C.H. Macular Pigment Optical Density Is Positively Associated with Academic Performance among Preadolescent Children. Nutr. Neurosci. 2018;21:632–640. doi: 10.1080/1028415X.2017.1329976[↩]
- Ma L, Hao ZX, Liu RR, Yu RB, Shi Q, Pan JP. Graefes Arch Clin Exp Ophthalmol. 2014 Jan;252(1):63-70. doi: 10.1007/s00417-013-2492-3. Epub 2013 Oct 23. A dose-response meta-analysis of dietary lutein and zeaxanthin intake in relation to risk of age-related cataract. https://www.ncbi.nlm.nih.gov/pubmed/24150707[↩]
- Bae J-M. Reinterpretation of the results of a pooled analysis of dietary carotenoid intake and breast cancer risk by using the interval collapsing method. Epidemiology and Health. 2016;38:e2016024. doi:10.4178/epih.e2016024. https://www.ncbi.nlm.nih.gov/pmc/articles/PMC4974449/[↩]
- Chen J, Jiang W, Shao L, Zhong D, Wu Y, Cai J. Int J Food Sci Nutr. 2016 Nov;67(7):744-53. doi: 10.1080/09637486.2016.1197892. Epub 2016 Jun 30. Association between intake of antioxidants and pancreatic cancer risk: a meta-analysis. https://www.ncbi.nlm.nih.gov/pubmed/27356952[↩]
- Perry A, Rasmussen H, Johnson EJ. Xanthophyll (lutein, zeaxanthin) content in fruits, vegetables and corn and egg products. J Food Compos Anal 2009:9–15.[↩]
- Chung HY, Rasmussen HM, Johnson EJ. Lutein bioavailability is higher from lutein-enriched eggs than from supplements and spinach in men. J Nutr. 2004 Aug;134(8):1887-93. doi: 10.1093/jn/134.8.1887[↩]
- Bowen PE, Herbst-Espinosa SM, Hussain EA, Stacewicz-Sapuntzakis M. Esterification does not impair lutein bioavailability in humans. J Nutr. 2002 Dec;132(12):3668-73. doi: 10.1093/jn/132.12.3668[↩]
- Yoshizako H, Hara K, Takai Y, Kaidzu S, Obana A, Ohira A. Comparison of macular pigment and serum lutein concentration changes between free lutein and lutein esters supplements in Japanese subjects. Acta Ophthalmol. 2016 Sep;94(6):e411-6. doi: 10.1111/aos.13106[↩]
- Norkus EP, Norkus KL, Dharmarajan TS, Schierle J, Schalch W. Serum lutein response is greater from free lutein than from esterified lutein during 4 weeks of supplementation in healthy adults. J Am Coll Nutr. 2010 Dec;29(6):575-85. doi: 10.1080/07315724.2010.10719896[↩]
- Bone RA, Landrum JT. Dose-dependent response of serum lutein and macular pigment optical density to supplementation with lutein esters. Arch Biochem Biophys. 2010 Dec 1;504(1):50-5. doi: 10.1016/j.abb.2010.06.019[↩]
- Sherry CL, Oliver JS, Renzi LM, Marriage BJ. Lutein supplementation increases breast milk and plasma lutein concentrations in lactating women and infant plasma concentrations but does not affect other carotenoids. J Nutr. 2014 Aug;144(8):1256-63. doi: 10.3945/jn.114.192914[↩]
- Ma L, Liu R, Du JH, Liu T, Wu SS, Liu XH. Lutein, Zeaxanthin and Meso-zeaxanthin Supplementation Associated with Macular Pigment Optical Density. Nutrients. 2016 Jul 12;8(7):426. doi: 10.3390/nu8070426[↩]
- Maiani G., Periago Caston M.J., Catasta G., Toti E., Cambrodon I.G., Bysted A., Granado-Lorencio F., Olmedilla-Alonso B., Knuthsen P., Valoti M., et al. Carotenoids: Actual knowledge on food sources, intakes, stability and bioavailability and their protective role in humans. Mol. Nutr. Food Res. 2009;53:S194–S218. doi: 10.1002/mnfr.200800053[↩]
- Abdel-Aal el-SM, Young JC, Akhtar H, Rabalski I. Stability of lutein in wholegrain bakery products naturally high in lutein or fortified with free lutein. J Agric Food Chem. 2010 Sep 22;58(18):10109-17. doi: 10.1021/jf102400t[↩]
- De La Parra C., Saldivar S.O.S., Lui R.H. Effect of processing on the phytochemical profiles and antioxidant activity of corn for production of masa, tortillas and tortilla chips. J. Agric. Food Chem. 2007;55:4177–4183. doi: 10.1021/jf063487p[↩]
- Abdel-Aal el-SM, Young JC, Rabalski I, Hucl P, Fregeau-Reid J. Identification and quantification of seed carotenoids in selected wheat species. J Agric Food Chem. 2007 Feb 7;55(3):787-94. doi: 10.1021/jf062764p[↩]
- Abdel-Aal E-SM, Akhtar H, Zaheer K, Ali R. Dietary Sources of Lutein and Zeaxanthin Carotenoids and Their Role in Eye Health. Nutrients. 2013;5(4):1169-1185. doi:10.3390/nu5041169. https://www.ncbi.nlm.nih.gov/pmc/articles/PMC3705341[↩]
- Salehi B, Venditti A, Sharifi-Rad M, Kręgiel D, Sharifi-Rad J, Durazzo A, Lucarini M, Santini A, Souto EB, Novellino E, Antolak H, Azzini E, Setzer WN, Martins N. The Therapeutic Potential of Apigenin. Int J Mol Sci. 2019 Mar 15;20(6):1305. doi: 10.3390/ijms20061305[↩][↩]
- Jang JY, Sung B, Kim ND. Role of Induced Programmed Cell Death in the Chemopreventive Potential of Apigenin. Int J Mol Sci. 2022 Mar 29;23(7):3757. doi: 10.3390/ijms23073757[↩]
- Chen P, Chen F, Guo Z, Lei J, Zhou B. Recent advancement in bioeffect, metabolism, stability, and delivery systems of apigenin, a natural flavonoid compound: challenges and perspectives. Front Nutr. 2023 Jul 26;10:1221227. doi: 10.3389/fnut.2023.1221227[↩]
- Mattila P, Astola J, Kumpulainen J. Determination of flavonoids in plant material by HPLC with diode-array and electro-array detections. J Agric Food Chem. 2000 Dec;48(12):5834-41. doi: 10.1021/jf000661f[↩]
- Kashyap P, Shikha D, Thakur M, Aneja A. Functionality of apigenin as a potent antioxidant with emphasis on bioavailability, metabolism, action mechanism and in vitro and in vivo studies: A review. J Food Biochem. 2022 Apr;46(4):e13950. doi: 10.1111/jfbc.13950[↩]
- Birt DF, Walker B, Tibbels MG, Bresnick E. Anti-mutagenesis and anti-promotion by apigenin, robinetin and indole-3-carbinol. Carcinogenesis. 1986;7:959–963. doi: 10.1093/carcin/7.6.959[↩]
- Papay ZE, Kosa A, Boddi B, Merchant Z, Saleem IY, Zariwala MG, Klebovich I, Somavarapu S, Antal I. Study on the pulmonary delivery system of apigenin-loaded albumin nanocarriers with antioxidant activity. J Aerosol Med Pulm Drug Deliv. 2017;30:274–288. doi: 10.1089/jamp.2016.1316[↩]
- Wang YC, Huang KM. In vitro anti-inflammatory effect of apigenin in the Helicobacter pylori-infected gastric adenocarcinoma cells. Food Chem Toxicol. 2013;53:376–383. doi: 10.1016/j.fct.2012.12.018[↩]
- Yan X., Qi M., Li P., Zhan Y., Shao H. Apigenin in cancer therapy: Anti-cancer effects and mechanisms of action. Cell Biosci. 2017;7:50. doi: 10.1186/s13578-017-0179-x[↩]
- Pápay Z.E., Kósa A., Böddi B., Merchant Z., Saleem I.Y., Zariwala M.G., Klebovich I., Somavarapu S., Antal I. Study on the pulmonary delivery system of apigenin-loaded albumin nanocarriers with antioxidant activity. J. Aerosol Med. Pulm. Drug Deliv. 2017;30:274–288. doi: 10.1089/jamp.2016.1316[↩]
- Zhu ZY, Gao T, Huang Y, Xue J, Xie ML. Apigenin ameliorates hypertension-induced cardiac hypertrophy and down-regulates cardiac hypoxia inducible factor-lalpha in rats. Food Funct. 2016;7:1992–1998. doi: 10.1039/C5FO01464F[↩]
- Ozcelik B, Kartal M, Orhan I. Cytotoxicity, antiviral and antimicrobial activities of alkaloids, flavonoids, and phenolic acids. Pharm Biol. 2011;49:396–402. doi: 10.3109/13880209.2010.519390[↩]
- Huang C, Wei YX, Shen MC, Tu YH, Wang CC, Huang HC. Chrysin, abundant in Morinda citrifolia fruit water-EtOAc extracts, combined with apigenin synergistically induced apoptosis and inhibited migration in human breast and liver cancer cells. J Agric Food Chem. 2016;64:4235–4245. doi: 10.1021/acs.jafc.6b00766[↩]
- Lee YM, Lee G, Oh TI, Kim BM, Shim DW, Lee KH, Kim YJ, Lim BO, Lim JH. Inhibition of glutamine utilization sensitizes lung cancer cells to apigenin-induced apoptosis resulting from metabolic and oxidative stress. Int J Oncol. 2016;48:399–408. doi: 10.3892/ijo.2015.3243[↩]
- Zhao G, Han X, Cheng W, Ni J, Zhang Y, Lin J, Song Z. Apigenin inhibits proliferation and invasion, and induces apoptosis and cell cycle arrest in human melanoma cells. Oncol Rep. 2017;37:2277–2285. doi: 10.3892/or.2017.5450[↩]
- Gupta S, Afaq F, Mukhtar H. Involvement of nuclear factor-kappa B, Bax and Bcl-2 in induction of cell cycle arrest and apoptosis by apigenin in human prostate carcinoma cells. Oncogene. 2002;21:3727–3738. doi: 10.1038/sj.onc.1205474[↩]
- Angulo P, Kaushik G, Subramaniam D, Dandawate P, Neville K, Chastain K, Anant S. Natural compounds targeting major cell signaling pathways: a novel paradigm for osteosarcoma therapy. J Hematol Oncol. 2017;10:10. doi: 10.1186/s13045-016-0373-z[↩]
- Xu M, Wang S, Song YU, Yao J, Huang K, Zhu X. Apigenin suppresses colorectal cancer cell proliferation, migration and invasion via inhibition of the Wnt/β-catenin signaling pathway. Oncol Lett. 2016 May;11(5):3075-3080. doi: 10.3892/ol.2016.4331[↩]
- Yan X, Qi M, Li P, Zhan Y, Shao H. Apigenin in cancer therapy: anti-cancer effects and mechanisms of action. Cell Biosci. 2017 Oct 5;7:50. doi: 10.1186/s13578-017-0179-x[↩][↩][↩]
- Cardenas H, Arango D, Nicholas C, Duarte S, Nuovo GJ, He W, Voss OH, Gonzalez-Mejia ME, Guttridge DC, Grotewold E, Doseff AI. Dietary apigenin exerts immune-regulatory activity in vivo by reducing NF-kappaB activity, halting leukocyte infiltration and restoring normal metabolic function. Int J Mol Sci. 2016;17:323. doi: 10.3390/ijms17030323[↩]
- Adel, M, Zahmatkeshan, M, Akbarzadeh, A, Rabiee, N, Ahmadi, S, Keyhanvar, P, et al. Chemotherapeutic effects of Apigenin in breast cancer: preclinical evidence and molecular mechanisms; enhanced bioavailability by nanoparticles. Biotechnol Rep (Amst). (2022) 34:e00730. doi: 10.1016/j.btre.2022.e00730[↩]
- Kashyap D., Sharma A., Tuli H.S., Sak K., Garg V.K., Buttar H.S., Setzer W.N., Sethi G. Apigenin: A Natural Bioactive Flavone-Type Molecule with Promising Therapeutic Function. J. Funct. Foods. 2018;48:457–471. doi: 10.1016/j.jff.2018.07.037[↩]
- Nabavi S., Habtemariam S., Daglia M., Nabavi S. Apigenin and Breast Cancers: From Chemistry to Medicine. Anticancer Agents Med. Chem. 2015;15:728–735. doi: 10.2174/1871520615666150304120643[↩]
- Rein M.J., Renouf M., Cruz-Hernandez C., Actis-Goretta L., Thakkar S.K., da Silva Pinto M. Bioavailability of Bioactive Food Compounds: A Challenging Journey to Bioefficacy. Br. J. Clin. Pharmacol. 2013;75:588–602. doi: 10.1111/j.1365-2125.2012.04425.x[↩]
- Thomas, S. D.; Jha, N. K.; Jha, S. K.; Sadek, B.; Ojha, S. Pharmacological and Molecular Insight on the Cardioprotective Role of Apigenin. Nutrients. 2023, 15(2), 385. DOI: 10.3390/nu15020385[↩]
- Fossatelli L, Maroccia Z, Fiorentini C, Bonucci M. Resources for Human Health from the Plant Kingdom: The Potential Role of the Flavonoid Apigenin in Cancer Counteraction. Int J Mol Sci. 2023 Dec 23;25(1):251. doi: 10.3390/ijms25010251[↩][↩]
- Schneider H., Blaut M. Anaerobic Degradation of Flavonoids by Eubacterium Ramulus. Arch. Microbiol. 2000;173:71–75. doi: 10.1007/s002030050010[↩]
- Al-Ishaq R.K., Liskova A., Kubatka P., Büsselberg D. Enzymatic Metabolism of Flavonoids by Gut Microbiota and Its Impact on Gastrointestinal Cancer. Cancers. 2021;13:3934. doi: 10.3390/cancers13163934[↩]
- Wang M, Firrman J, Liu L, Yam K. A Review on Flavonoid Apigenin: Dietary Intake, ADME, Antimicrobial Effects, and Interactions with Human Gut Microbiota. Biomed Res Int. 2019 Oct 16;2019:7010467. doi: 10.1155/2019/7010467[↩]
- Tang D., Chen K., Huang L., Li J. Pharmacokinetic properties and drug interactions of apigenin, a natural flavone. Expert Opinion on Drug Metabolism & Toxicology. 2017;13(3):323–330. doi: 10.1080/17425255.2017.1251903[↩]
- Lefort É. C., Blay J. Apigenin and its impact on gastrointestinal cancers. Molecular Nutrition & Food Research. 2013;57(1):126–144. doi: 10.1002/mnfr.201200424[↩]
- Simirgiotis M. J., Schmeda-Hirschmann G., Bórquez J., Kennelly E. J. The Passiflora tripartita (banana passion) fruit: a source of bioactive flavonoid C-glycosides isolated by HSCCC and characterized by HPLC-DAD-ESI/MS/MS. Molecules. 2013;18(2):1672–1692. doi: 10.3390/molecules18021672[↩]
- Hostetler GL, Ralston RA, Schwartz SJ. Flavones: Food Sources, Bioavailability, Metabolism, and Bioactivity. Adv Nutr. 2017 May 15;8(3):423-435. doi: 10.3945/an.116.012948[↩]
- Antioxidant Supplements: What You Need To Know. https://www.nccih.nih.gov/health/antioxidant-supplements-what-you-need-to-know[↩]
- Albanes D, Heinonen OP, Taylor PR, Virtamo J, Edwards BK, Rautalahti M, Hartman AM, Palmgren J, Freedman LS, Haapakoski J, Barrett MJ, Pietinen P, Malila N, Tala E, Liippo K, Salomaa ER, Tangrea JA, Teppo L, Askin FB, Taskinen E, Erozan Y, Greenwald P, Huttunen JK. Alpha-Tocopherol and beta-carotene supplements and lung cancer incidence in the alpha-tocopherol, beta-carotene cancer prevention study: effects of base-line characteristics and study compliance. J Natl Cancer Inst. 1996 Nov 6;88(21):1560-70. doi: 10.1093/jnci/88.21.1560[↩][↩]
- Institute of Medicine. Food and Nutrition Board. Dietary Reference Intakes: Vitamin C, Vitamin E, Selenium, and Carotenoids. Washington, DC: National Academy Press, 2000. https://nap.nationalacademies.org/read/9810/chapter/1[↩]
- Sesso HD, Buring JE, Christen WG, Kurth T, Belanger C, MacFadyen J, Bubes V, Manson JE, Glynn RJ, Gaziano JM. Vitamins E and C in the prevention of cardiovascular disease in men: the Physicians’ Health Study II randomized controlled trial. JAMA. 2008 Nov 12;300(18):2123-33. doi: 10.1001/jama.2008.600[↩]